Adhesion and Biofilms on Contact Lenses
Introduction
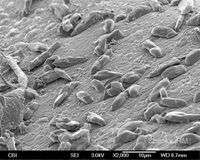
About 125 million people in the world wear contact lenses; for many people, wearing contact lenses can be used to correct vision [1] [2] [3]. However, there are potential risks when wearing contact lenses, such as experiencing adverse reactions and ocular infections [1] [2] [4] [5].
A list of the possible responses can be found here.
In some of these cases, the involvement of microbes and their biofilms on the surfaces of contact lenses have been reported [1] [2] [4] [5]. The findings are significant because a combination of wearing contact lenses and the presence of microbes, and/or the formation of biofilms has been suggested for higher rates of infections [4] [6] [7]. When the contact lenses are inserted, the cornea will be continually exposed to microbes in the biofilm, which could then lead to ocular infections [7].
A further reading on contact lenses can be found here.
Biofilm Formation on Contact Lenses
A general definition and characteristics of a biofilm can be found here.
The “conditioning film,” which is a layer of organic molecules including lipids and proteins deposited from tears onto the contact lenses, is the surface that the microbe first binds and absorbs to [1] [2] [8]. With the help of the "conditioning film," the microbes are able to reach the contact lenses [1]. Initially, a few individual microbes arrive and adhere to the contact lenses; gradually, the surfaces accumulates more microbes [1]. The attachment is generally described as reversible and involving Van der Waals forces [1] [9]. Eventually, production of EPS then results in irreversible attachment between the microbes, leading to the development and growth of a biofilm on the contact lenses [1] [9]. The microbes may then grow and colonize due to the “conditioning film” being able to provide nutrients [2]. Afterwards, quorum sensing, signalling between cells, becomes important within biofilms; it can aid microbes in attachment onto surfaces and it can be involved with expression of certain genes such as those needed for differentiation [7] [10].
Physical Environment
Characteristics of contact lenses such as hydrophobicity and the type of surface may also be factors in adhesion and biofilm formation respectively [1]. Hydrophobicity is a factor in the adhesion and the type of microbes on contact lenses because it affects the "conditioning film." [1]. Microbes that adhere hydrophobic surfaces tend to use polymers that are non-polar while microbes that adhere hydrophilic surfaces tend to use EPS and lipopolysacchardies (LPS) [7]. Biofilm formation tends to be on the posterior surface of the contact lenses rather than the anterior surface [11]. There are less defence against adherence and colonization on the posterior surface, where the contact lenses would meet the cornea; the interaction between the two surfaces could cause the natural defence mechanisms present on the cornea to not function properly [11] [12] [13]. The natural defence mechanisms would include blinking of the eyes, the surface of the cornea, and tears containing antimicrobial agents [12]. They would be present on the anterior surface though, thus warding off biofilm formation [11] [12].
Biological Interactions – Gene Transfer and Resistance
Because of the nature of the biofilm, treatment to infections may be a problem as resistance to antimicrobial agents and antibiotics may be gained, enhanced and promoted to other microbes [7] [14] [15] [16]. Microbes within the biofilm transcribe different sets of genes and may also exchange genes and plasmids with one another more rapidly through conjugation [7]. Antibiotic resistance can then be acquired through the exchange of genes and plasmids [7]. The resistance may also be due to the matrix of the biofilms, which slows down or inactivates the substances [15]. Another reason could be the slower growth rates of microbes within the biofilms, which lead to a decrease of uptake of the substances [15].
Key Micoorganisms
Bacteria that have been associated with contact lenses and ocular infections include Archromobacter, Delftia, Staphylococcus, Stenotrophomonas, and Streptococci species, and Pseudomonas aeruginosa [12] [16]. The key microorganisms discussed in this section would be ones with specific factors for adhesion and implicated with ocular infections [17] [18] [19].
Pseudomonas aeruginosa
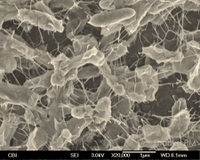
Pseudomonas aeruginosa is a gram negative bacteria most commonly found to be the cause of these ocular infections [19]. A reason why P. aeruginosa has been observed in microbial keratitis is due to its ability adhere tightly to the surfaces of contact lenses [1]. When P. aeruginosa interacts and adhere with the contact lenses, its production of slime becomes an important factor [17]. Other factors involved may be swimming by flagellum, pili and various sites on the bacteria itself [20]. Once the immune response occurs however, neutrophils and their components such as its DNA and F actin, can aid in and increase the formation of biofilms on the contact lenses [21]. Infections may occur due to P. aeruginosa's ability to perform quorum sensing, which allows the biofilm to evade detection of the immune system and express virulence factors when ready [19].
A further reading on P. aeruginosa can be found here.
Staphylococcus epidermidis
Another significant microbe associated with contact lenses and microbial keratitis is Staphylococcus epidermis. [17] [18]. The slime of S. epidermidis has polysaccharide adhesion (PS/A), which has been shown to be involved in attachment to surfaces such as contact lenses [18]. It was discovered that the bacteria strains who could and couldn't produce slime were able to colonize the contact lenses after adhesion; the former resulted in adhesion at a greater extent [18] [22]. Polysaccharide intracellular adhesions (PIA), which is involved in biofilm formation, may also work with the slime and PS/A during adhesion to surfaces [9] [23].
A further reading on S. epidermidis can be found here.
Current Research
In order to prevent future growth of bacteria and devise treatments for infections that may arise, researchers have suggested studying biofilms on contact lenses [4]. To lower the rates of ocular infections, suggestions has been made to use more effective antibiotics and to modify the contact lenses’ material [14] [15]. Implementing these ideas could then prevent the adhesion and biofilm formation of microbes [14] [15]. For example, antibiotics can be present on the contact lenses; the results could lead to prevention of adhesion and growth but eventual resistance [2] [15].
References
(1) Bruinsma, G. M., van der Mei, H. C., & Busscher, H. J. (2001). Bacterial adhesion to surface hydrophilic and hydrophobic contact lenses. Biomaterials, 22(24): 3217-3224.
(2) Willcox, M. D., Harmis, N., Cowell, Williams, T., & Holden. (2001). Bacterial interactions with contact lenses; effects of lens material, lens wear and microbial physiology. Biomaterials, 22(24): 3235-3247.
(3) Barr, J. (2005). Contact Lens Spectrum's annual report of major corporate and product developments and events in the contact lens industry in 2004, as well as predictions for 2005. Retrieved from http://www.clspectrum.com/articleviewer.aspx?articleid=12733
(4) Zegans, M. E., Becker, H. I., Budzik, J., & O'Toole, G. (2002). The role of bacterial biofilms in ocular infections. DNA and Cell Biology, 21(5-6): 415-420.
(5) Willcox, M. D. P. (2013). Microbial adhesion to silicone hydrogel lenses: A review. Eye & Contact Lens, 39(1): 61-66.
(6) Elder, M. J., Stapleton, F., Evans, E., & Dart, J. K. (1995). Biofilm-related infections in ophthalmology. Eye (London, England), 9: 102-109.
(7) Donlan, R. M. (2002). Biofilms: Microbial life on surfaces. Emerging Infectious Diseases, 8(9): 881-890.
(8) Soltys-Robitaille, C. E., Ammon, J., D M, Valint, J., P L, & Grobe, 3., G L. (2001). The relationship between contact lens surface charge and in-vitro protein deposition levels. Biomaterials, 22(24): 3257-3260.
(9) Dutta, D., Cole, N., & Willcox, M. (2012). Factors influencing bacterial adhesion to contact lenses. Molecular Vision, 18: 14-21.
(10) Rédei, G. P. (2008). Quorum Sensing. In Encyclopedia of genetics, genomics, proteomics, and informatics. (Vol. 1, pp. 1614-1615) New York.
(11) Tam, C., Mun, J. J., Evans, D. J., & Fleiszig, S. M. J. (2010). The impact of inoculation parameters on the pathogenesis of contact lens-related infectious keratitis. Investigative Ophthalmology & Visual Science, 51(6): 3100-3106.
(12) Liesegang, T. J. (1997). Contact lens-related microbial keratitis: Part II: Pathophysiology. Cornea, 16(3): 265-273.
(13) Henriques, M., Sousa, C., Lira, M., Elisabete, M., Oliveira, R., Oliveira, R., & Azeredo, J. (2005). Adhesion of Pseudomonas aeruginosa and Staphylococcus epidermidis to silicone-hydrogel contact lenses. Optometry and Vision Science : Official Publication of the American Academy of Optometry, 82(6): 446-450.
(14) McLaughlin-Borlace, L., Stapleton, F., Matheson, M., & Dart, J. K. (1998). Bacterial biofilm on contact lenses and lens storage cases in wearers with microbial keratitis. Journal of Applied Microbiology, 84(5): 827-838.
(15) Donlan, R. M. (2000). Role of biofilms in antimicrobial resistance. ASAIO Journal, 46(6): S47-S52.
(16) Wiley, L., Bridge, D. R., Wiley, L. A., Odom, J. V., Elliott, T., & Olson, J. C. (2012). Bacterial biofilm diversity in contact lens-related disease: Emerging role of Achromobacter, Stenotrophomonas, and Delftia. Investigative Ophthalmology & Visual Science, 53(7): 3896.
(17) Madison Slusher, M., Myrvik, Q.N., Lewis, J.C., & Gristina A.G. (1987). Extended-Wear Lenses, Biofilm, and Bacterial Adhesion. JAMA Ophthalmology, 105(1): 110-115.
(18) Fleiszig, S. M., Evans, D. J., Mowrey-McKee, M. F., Payor, R., Zaidi, T. S., Vallas, V., & Pier, G. B. (1996). Factors affecting Staphylococcus epidermidis adhesion to contact lenses. Optometry and Vision Science : Official Publication of the American Academy of Optometry, 73(9): 590-594.
(19) Willcox, M. D. P. (2007). Pseudomonas aeruginosa infection and inflammation during contact lens wear: A review. Optometry and Vision Science : Official Publication of the American Academy of Optometry, 84(4): 273-278.
(20) Tran, V. B., Fleiszig, S. M. J., Evans, D. J., & Radke, C. J. (2011). Dynamics of flagellum- and pilus-mediated association of Pseudomonas aeruginosa with contact lens surfaces. Applied and Environmental Microbiology, 77(11): 3644-3652.
(21) Robertson, D. M., Cavanagh, H. D., Nick, J. A., Parks, Q. M., Young, R. L., Kret, J., Poch, K.R., Malcolm, C., Nichols, D.P., Nichols, M., Zhu, M., Cavanagh, D.H., & Nick, J.A. (2011). Disruption of contact lens-associated Pseudomonas aeruginosa biofilms formed in the presence of neutrophils. Investigative Ophthalmology & Visual Science, 52(5): 2844-2850.
(22) Garcia-Saenz, M. C., Arias-Puente, A., Fresnadillo-Martinez, M. J., & Paredes-Garcia, B. (2002). Adherence of two strains of staphylococcus epidermidis to contact lenses. Cornea, 21(5): 511-515.
(23) Cafiso, V., Stefani, S., Bertuccio, T., Santagati, M., Campanile, F., Amicosante, G., Perilli, M. G., Selan, L., Artini, M., Nicoletti, G. & Stefani, S. (2004). Presence of the ica operon in clinical isolates of Staphylococcus epidermidis and its role in biofilm production. Clinical Microbiology and Infection, 10(12): 1081-1088.
(24) Shanks, R.M.Q. Scanning electron micrograph of dirty contact lens, Retrieved November 22, 2013, from: http://eyemicrobiology.upmc.com/Images/Sub/PhotoGallery/Biofilms/PhotoDirtyLens.jpg
(25) Shanks, R.M.Q. Scanning electron micrograph of Pseudomonas aeruginosa biofilm (false colored), Retrieved November 22, 2013, from: http://eyemicrobiology.upmc.com/Images/Sub/PhotoGallery/Biofilms/PhotoPaerugBiofilm.jpg