Quorum Sensing in Staphylococcus aureus Infections
Introduction to Staphylococcus aureus
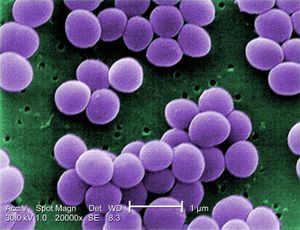
By Elizabeth Eder
Staphylococcus aureus is a gram-positive cocci-shaped bacterium that commonly colonizes human epithelia. Epithelial surfaces include the skin and respiratory tract, and in these areas S. aureus is considered a natural component of the human microbiome and does not serve as a benefit or detriment to its human host [1]. First identified in 1880, S. aureus is a facultative anaerobe, meaning it generates ATP with or without oxygen [2]. While S. aureus is not naturally pathogenic on epithelial surfaces, infections can occur in these areas and range from pimples and impetigo to pneumonia and meningitis [1, 3]. Pathogenicity can develop through the infection of S. aureus in the bloodstream, and these infections are of great medical relevance due to their prevalence and virulence. Infections can be community-acquired (CA) or hospital-acquired (HA), with the latter illustrating the challenge of sterility in healthcare settings [4].
Compounding the issue of infection is the bacteria’s ability to develop drug resistance, resulting in strains such as methicillin-resistant Staphylococcus aureus (MRSA) [5]. These strains have been of increasing concern in the medical field for their persistence in nosocomial infections and comprise the majority of S. aureus infections [2, 4]. The frequency of S. aureus infections, and the frequency of those infections acquiring methicillin-resistance, is increasing in hospitals in the U.S. [2, 5]. The CDC reported a 62% increase in S. aureus-related hospitalizations from 1999 to 2005, and suggested that MRSA be considered “a national priority for disease control” [5]. Understanding the molecular mechanism of virulence is critical for addressing the issue of S. aureus and MRSA infections, because the resistance that S. aureus develops seriously limits antibiotic usefulness [FIG 1]. Despite the advances in patient care, technology, and heath environment sterility, S. aureus infections continue to be an issue in U.S. hospitals and communities [4]. One mechanism of S. aureus that is currently being studied for its potential contribution to pathogenicity is the quorum sensing (QS) system of communication. Quorum sensing contributes to biofilm formation, antibiotic resistance, and host defense resistance, making the system of great importance for S. aureus infections [1, 7].
Medical Relevance and MRSA
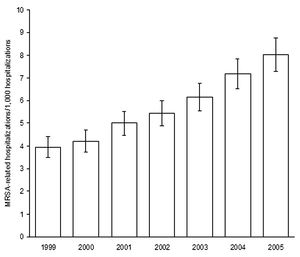
Staphylococcus aureus can become pathogenic whether or not it develops antibiotic resistance, but cases where strains do become resistant are of great concern for the morbidity and mortality associated with them [2]. The economic costs infections impose on hospitals are also serious and significant [4]. S. aureus isolates that do begin to acquire antimicrobial drug resistance are usually resistant to methicillin and other beta-lactam drugs, but progress through a series of stages prior to complete resistance [5]. Isolates are initially considered susceptible, and then progress to intermediate, and finally resistant.
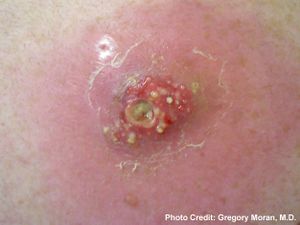
Staphylococcus aureus infections can be categorized into five main types: localized skin infection, diffuse skin infection, deep localized infections, toxinoses, and other infections [3, 6]. Localized skin infections can result in cutaneous or subcutaneous abscesses and possibly bacteremia [FIG 2]. Bacteremia is the infection of bacteria in the blood, which is normally a sterile and bacteria-free environment. When bacteria such as S. aureus are found in the blood, intravenous antibiotics are used as treatment to prevent further spread from the blood into the heart endocardium or bone marrow. Diffuse skin infections include impetigo, which results in facial sores and blisters and is also treated with antibiotics. Deep localized infections include infection of bone and bone marrow, called osteomyelitis, as well as septic arthritis. Toxinoses include toxic shock syndrome and gastroenteritis, but are not necessarily caused by bacterial infection but by bacterial toxins. S. aureus produces toxins that can lead to toxinosis when ingested. The other infections S. aureus can cause include endocarditis, pneumonia, and other inflammatory responses [4].
Antibiotics used to treat MRSA are vancomycin, linezolid, teicoplanin, tigecycline, daptomycin, and telavancin. Vancomycin remains the most common, though different studies investigating each antibiotic have revealed pros and cons to each [4]. The over-prescription and over-use of antibiotics has been controversial in aiding the progression of multiple species of bacteria towards antibiotic resistance, and as strains continually develop less susceptibility newer antibiotics with different antimicrobial targets are in demand [2, 5]. In healthcare settings infections by both S. aureus and MRSA have increased over 1999-2005 as reported by the CDC [FIG 3]. S. aureus-related infections in the U.S. increased from 294,570 to 477,927 and of those totals the number of MRSA infections increased from 127,036 to 278,203. Resistance to ampicillin, cephalothin, and erythromycin also increased over that period of time [5].
Quorum Sensing System
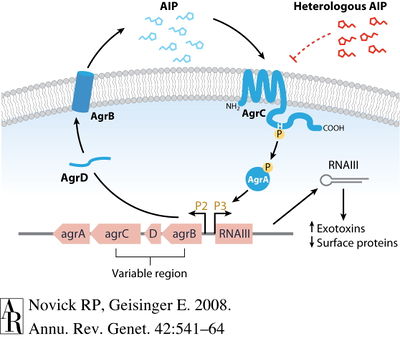
Quorum sensing (QS) is a kind of chemical communication between individual bacterium and groups of bacteria that is dependent on the surrounding environmental conditions, cell density, and other regulatory factors [7]. This communication can influence bacterial behavior, benefitting the bacteria when collaboration produces a response to the environment. The quorum sensing system in Staphylococcus aureus occurs through the accessory gene regulator (agr) [7, 8, 9]. It is a complex molecular mechanism and though the pathway is elucidated, the response of QS itself is not well understood.
Agr functions to increase the expression of certain virulence factors secreted by S. aureus and decrease certain cell surface proteins [8]. There is evidence that agr contributes to S. aureus pathogenicity in several infection types, including subcutaneous abscesses, endocarditis, and arthritis. On a molecular level, the agr locus contains promoters P2 and P3 that encodes transcripts for RNAII and RNAIII, respectively [7, 8]. The P2 and P3 operons code for different proteins that have individual roles in agr-sensing. P2 operon encodes the RNAII transcript that produces gene products agrB, agr D, agrC, and agrA [8]. These four products comprise the actual signaling system [FIG 4]. The P3 operon encodes the RNAIII transcript that produces only one gene product—a toxin called delta-hemolysin—but that increases transcription of several virulence factors. However, it has been noted that the agr system is not necessary for the expression of virulence factors like toxins. AgrD and AgrB work together to produce an auto-inducing peptide (AIP). AgrD encodes for pro-AIP and transmembrane protein AgrB processes the pro-AIP to AIP, transporting it to the external cellular space. AIP will continue to accumulate in the external environment beyond the cell membrane, eventually binding to AgrC, a kinase embedded within the membrane. The AgrC kinase phosphorylates regulator AgrA, which then is responsible for the autoinduction of the agr locus by binding to the P2 and P3 promoters and initiating the cycle again. The agr-sensing system is a circuit that is auto-activating [8].
The agr system is an important component of QS, and has its own accessory regulators that are identified but not well understood functionally. Regulators of agr affect expression in differing environments and cell densities, and can either encourage or inhibit biofilm formation. In S. aureus one agr regulator is a two-component system, comprised of AgrA and AgrC that works to sense surroundings. DNA-binding proteins like staphylococcal accessory regulator (SarA) contribute to agr regulation at the transcriptional level, reportedly required for full agr transcription. Repressor of toxins (Rot) is another transcriptional regulator of the agr locus and has been shown to work in antithesis to agr. Additional regulators at the transcriptional and environmental level have been studied but are not fully understood in this complex network [8].
QS and Pathogenicity

Quorum sensing has been noted to control systems ranging from biofilm formation to antibiotic resistance to virulence factor secretion [7]. All of these mechanisms are critical for the pathogenicity of S. aureus and MRSA in vivo. Biofilm formation is not itself considered a benefit to virulence, but a mechanism that ultimately increases individual cellular virulence and proliferation. QS via the agr system works to regulate S. aureus biofilms [7]. A biofilm is a grouping a cells together in an adherent colony, and is considered high cell density [9]. Biofilms have been observed to increase antibiotic resistance and are thus of great relevance to S. aureus infections in a healthcare setting [7, 10]. Inhibition of biofilm formation occurs once a colony that began at low cell density has grown to high cell density and results in dispersal of the healthiest and newest cell growth. The newest cell growth is better prepared to produce virulence factors having been established in a biofilm community. Detachment and dispersal occurs when cell surface proteins decrease and cells lose adhesion [FIG 5] [7]. Surfactant molecules have been proposed to be critical in biofilm structure and detachment, based on systems in Bacillus subtilis and Pseudomonas aeruginosa. In Staphylococci, phenol-soluble modulin (PSM) peptides have surfactant molecule properties and are under transcriptional control of AgrA. Agr controls biofilm formation and in S. aureus PSMs regulated by agr contribute to biofilm detachment [9]. Agr also regulates production of alpha-toxin, delta-hemolysin, and adhesins necessary for biofilm formation and maturation [7, 10].
The strategy behind biofilm manipulation lends itself to successful infection—the few cells to initiate an infection must express surface virulence factors in an effort to avoid immune system defenses. Surface virulence factors include Protein A and clumping factor [1]. Once cells survive they can adhere to a surface and colonize an infection site—clumping factor and adhesins assist S. aureus with colonization. As the colony grows, the high number of cells does not need to expend energy expressing cell surface virulence factors, which are consequently down-regulated by QS. Detachment due to PSM production and loss of adhesion then releases cells from the biofilm to continue infection at a new site, and surface virulence factors are again expressed when mobilized [9].
QS via agr increases production of virulence factors, which include various enzymes and toxins. Coagulase is an enzyme that coats the cell surface in fibrin as protection from immune defenses like phagocytosis. Toxins include superantigens, proteases, exfoliative toxins, hemolysins (alpha, beta, gamma, delta), and leukocidin [1, 10].
Infection Prevention and Treatment
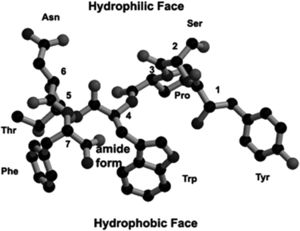
Antibiotic therapy, when used properly and with caution, is effective in eliminating many types of S. aureus infections. MRSA infections, however, pose a greater challenge. Few antibiotics are currently effective in elimination of MRSA, and there have been cases of susceptible colonies (MSSA) evolving into resistant colonies (MRSA) within the host [11]. Intermediate susceptibility has been reported for S. aureus strains in vancomycin as well (VISA) [4]. Vancomycin remains a stronghold for MRSA treatment, but in the future antibiotics under development include dalbavancin, oritavancin, ceftobiprole, and ceftaroline [12]. Dalbavancin and oritavancin are in the class of lipoglycopeptides, while ceftobiprole and ceftaroline are cephalosporins.
As antibiotics continually seek to target different microbial components, an important target that is also under consideration in S. aureus and MRSA infections is the QS system. There is evidence of a potential drug target in the QS system that acts to disrupt the cell-to-cell communication and prevent virulence by inhibiting RNAIII [10]. A natural product found in the bark of witch hazel trees, hamamelitannin, is an analog of RNAIII-inhibiting peptide (RIP) [FIG 6]. RIP is likely an antagonist to the TRAP receptor, TRAP being a membrane-associated protein part of the S. aureus agr system. Hamamelitannin does not reduce growth of S. aureus, MRSA, or VISA in vitro, and so is not considered an antibiotic, but does prevent RNAIII transcript production and cell adhesion. The disruption of the QS system via RNAIII is important when considered in conjunction with prevention of cell adhesion—since a disrupted QS system would result in increased biofilm formation, and no cell adhesion eliminates this component of S. aureus infection as well [10].
Hammamelitannin has been recognized as a QS inhibitor (QSI) and studied for its antibiofilm properties in S. aureus infection. In conjunction with antibiotics such as vancomycin or clindamycin, hammamelitannin is effective in killing S. aureus biofilm cells at levels greater than QSI or antibiotic alone [13]. This method of combining treatments is promising for tackling complex infections like biofilm-associated S. aureus and MRSA. A distinction between acute and chronic S. aureus infection has been hypothesized to result from the infection’s biofilm formation abilities. If the QS system is disrupted so that agr function is lost, the infection may survive better long-term due to the persistence of biofilm. Acute infections rely more heavily on the virulence factors S. aureus secretes, and hypothetical therapies aimed at eliminating agr function could convert an acute infection into a chronic infection characterized by biofilms [1].
Practices to avoid S. aureus and MRSA infections have been determined and compiled in numerous studies, with varying efficacies. Infection prevention methods within the healthcare environment include screening of patients and staff, hand washing and antimicrobials, as well as gowns, gloves, and environmental cleaning. Issues presented by these methods include cost effectiveness, poor compliance, and little effectiveness [4].
References
[1] Yarwood JM, Schlievert PM. 2003. Quorum sensing in Staphylococcus infections. J. Clin. Invest. 112:1620-1625.
[2] Boucher HW and Corey GR. 2008. Epidemiology of methicillin-resistant Staphylococcus aureus. Clinical Infectious Diseases 46:344-349.
[3] Howden BP, Davies JK, Johnson PDR, Stinear TP, Grayson ML. 2010. Reduced vancomycin susceptibility in Staphylococcus aureus, including vancomycin-intermediate and heterogeneous vancomycin-intermediate strains: resistance mechanisms, laboratory detection, and clinical implications. Clinical Microbiology Reviews 23(1):99-139.
[4] Haddadin AS, Fappiano SA, Lipsett PA. 2002. Methicillin resistant Staphylococcus aureus (MRSA) in the intensive care unit. Postgrad Med J 78:385-392.
[5] Klein E, Smith DL, Laxminarayan R. 2007. Hospitalizations and deaths caused by methicillin-resistant Staphylococcus aureus, United States, 1999–2005. Emerg Infect Dis 13(12):1840-1846.
[6] Diekema DJ, Pfaller MA, Schmitz FJ, Smayevsky J, Bell J, Jones RN, Beach M. 2001. Survey of infections due to Staphylococcus species: frequency of occurrence and antimicrobial susceptibility of isolates collected in the United States, Canada, Latin America, Europe, and the Western Pacific for the SENTRY Antimicrobial Surveillance Program, 1997-1999. Clinical Infectious Diseases 32:S114-S132.
[7] Yarwood JM, Bartels DJ, Volper EM, Greenberg EP. 2004. Quorum sensing in Staphylococcus aureus biofilms. J Bacteriol 186:1838-1850.
[8] Novick RP and Geisinger E. 2008. Quorum sensing in Staphylococci. Annu Rev Genet 42:541-564.
[9] Periasamy S, Joo H, Duong AC, Bach TL, Tan VY, Chatterjee SS, Cheung GY, Otto M. 2011. How Staphylococcus aureus biofilms develop their characteristic structure. PNAS 109:1281-1286.
[10] Kiran MD, Adikesavan NV, Cirioni O, Giacometti A, Silvestri C, Scalise G, Ghiselli R, Saba V, Orlando F, Shoham M, Balaban N. 2008. Discovery of a quorum-sensing inhibitor of drug-resistant staphylococcal infections by structure-based virtual screening. Mol Pharmacol 73:1578-1586.
[11] Gao W, Chua K, Davies JK, Newton HJ, Seemann T, Harrison PF, Holmes NE, Rhee HW, Hong JI, Hartland EL, Stinear TP, Howden BP. 2010. Two novel point mutations in clinical Staphylococcus aureus reduce linezolid susceptibility and switch on the stringent response to promote persistent infection. PLoS Pathogens 6(6):e1000944.
[12] Micek ST. 2007. Alternatives to Vancomycin for the treatment of Methicillin-resistant Staphylococcus aureus infections. Clinical Infectious Diseases 45:S184-S190.
[13] Brackman G, Cos P, Maes L, Nelis HJ, Coenye T. 2011. Quorum Sensing inhibitors increase the susceptibility of bacterial biofilms to antibiotics in vitro and in vivo. Antimicrobial Agents and Chemotherapy 55(6):2655-2661.
Authored for BIOL 291.00 Health Service and Biomedical Analysis, taught by Joan Slonczewski, 2016, Kenyon College.