The Gut Microbiome & Parkinson's disease
Introduction to Parkinson's disease: A Gut-Brain Connection
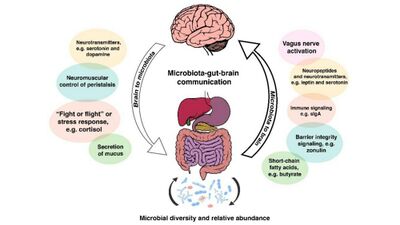
By Ania Axas
Parkinson’s disease (PD) results from the death of dopaminergic neurons in the substantia nigra, which is part of the basal ganglia structure in the midbrain.[1] PD genes discovered have led to the reasoning that misfolded proteins and the dysfunction of the ubiquitin-proteasome pathway are crucial to the pathogenesis of PD.[1] As a common neurodegenerative disorder, Parkinson’s presents with both motor and nonmotor symptoms.[2] The nonmotor symptoms often begin much earlier than the motor symptoms, and it has now been found that a neuronal protein responsible for regulating synaptic vesicle trafficking and neurotransmitter release, α-synuclein, is found in the enteric nervous system and substantia nigra of PD patients.[2] While the cause of PD is not known, microglial activation and further neuroinflammation is linked with α-syn being misfolded in PD.[3] Also, even though α-syn is most commonly found in the brain, certain aggregates have been found in peripheral locations like the enteric nervous system (ENS); these findings contribute to the support of the gut-brain axis as a bidirectional communication pathways between the enteric and central nervous systems and the GI system.[3] Healthy intestinal microbiota play an important part in keeping the blood-brain barrier (BBB) working properly with the help of regulated tight junction protein expression and then mediated by short chain fatty acids (SCFAs).[3] The primary substrate for the production of SCFAs like propionate, butyrate, and acetate is fiber; butyrate is an SCFA capable of causing disruption to gut barrier function and promotes inflammation of the gut.[4] Keeping all of these facts in mind, this new knowledge contributes to more evidence linking the gut microbiome and related symptoms as a precursor to PD.
Parkinson’s is one of the most common neurodegenerative diseases, second only after Alzheimer’s disease; PD’s estimated prevalence is about 1% among people over the age of 65.[3] In 1990, there were approximately 2.5 million PD patients while in 2016, the number increased to over 6.1 million patients.[3] The trend is increasing as the global population ages and it is also said to increase among males. [3] By 2030, PD prevalence is estimated to reach about 1,238,000 cases[3], which means that more research must be done in order to lessen the reality of these predictions. Parkinson’s disease is a multifactorial disease which has environmental as well as genetic risk factors[3]. Particular environmental factors include but are not limited to caffeine consumption, cigarette smoking, head injury, and exposure to herbicides/pesticides or heavy metals.[3] Genetically, 187 genes in ~90 loci have been linked to idiopathic (spontaneously deriving) PD and 23 genes have been associated with Mendelian forms of PD.[3]
In addition, the best current medication for the treatment of PD is levodopa (L-dopa), which is the precursor to dopamine but unlike dopamine, can cross the BBB.[5] L-dopa is able to convert dopamine in the central and peripheral nervous system as this allows for the control of the symptoms relating to the general slowness PD causes and to improve the quality of life in Parkinson’s disease patients.[5] However, recent research has indicated that device-assisted therapies may also have an effect on the gut microbiome in Parkinson’s disease and should certainly be explored as an alternative option to oral medications.[6]
Dysbiosis of the Gut Microbiome
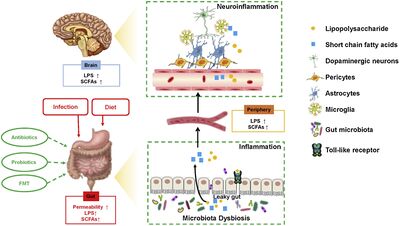
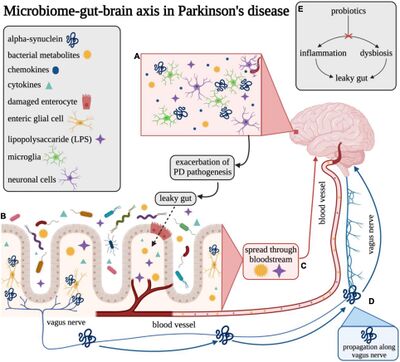
The gut microbiota include a diverse group of bacterial species which can be found in the GI tract.[4] Many factors contribute to the gut microbiota, and some of these factors include antibiotic treatments the host has used and their specific diet.[4] A stable and healthy gut microbiome aids in maintaining metabolism, homeostatic balance, gut immunity, and regulation of the gut-brain axis and blood-brain barrier.[4]
Dysbiosis is defined as a reduction in microbial diversity combined with the loss of certain beneficial bacterial strains like Bacteroides and butyrate-producing bacteria (Firmicutes).[7] A rise in pathobionts like Proteobacteria, which are symbiotic bacteria capable of becoming pathogenic under particular conditions, are also seen in dysbiosis of the gut microbiome.[7] Since microbial metabolites and microbiota can affect host metabolism, behavior, immunity, and cognition, more attention has been placed on possible phenotypic correlations in PD pathogenesis.[4] For example, a reduction in the amount of Prevotellaceae from PD patients’ fecal samples was found when compared to a healthy population.[4] Further, there is a direct correlation between how much Enterobacteriaceae was found in PD patients and the severity of gait difficulty and postural instability.[4] Overall, consistent findings that studies have observed relate to an increase in the bacteria in the Enterobacteriaceae and Helicobacter families as well as increases in Akkermansia and Lactobacillus.[8] Lactobacillaceae has often been linked with reduced concentrations of ghrelin, which is a gut-brain peptide hormone implicated in upregulating intestinal tight junction proteins, protecting normal nigrostriatal dopamine function, and stimulating intestinal motility.[8] Akkermansia has been found to use mucus as a carbon source and then further degrades the colonic mucus barrier, disrupting the permeability of the intestines and increasing susceptibility to pathogens capable of causing inflammation on intestinal walls.[8]
There is much evidence proving the fact that PD and gut dysbiosis is connected.[4] Another example of this is the high prevalence of Helicobacter pylori (H. pylori) infection in patients, which was then attributed to gastric irritation and dyspepsia (indigestion) symptoms as either nonmotor symptoms of PD or side effects of levodopa.[4] If H. pylori gets eliminated from the gut microbiome, an improvement in the absorption of L-dopa is observed and better responses of motor function to treatment are also seen.[8] Additional small intestinal bacterial overgrowth was observed in patients with PD, and is associated with malabsorption, flatulence, bloating, and severe fluctuations in motor symptoms.[4] A recent study even reported that bacteria associated with anti-inflammatory properties, like Roseburia, Coprococcus, and Blautia, were all reduced significantly in fecal samples taken from PD patients.[4] Additionally, there was a reduction in Faecalibacterium and an increase in Ralstonia in PD patients’ mucosa; this increase has the ability to shift the microbial balance in the colon to become more inflammatory and uncomfortable.[4] Yet another study has also shown microbial imbalance, with the number of Lactobacillus being higher while Clostridium coccoides, Bacteroides fragilis, and Prevotella were all lower.[4] A reduction in Prevotella can be detrimental to the gut microbiome because this leads to a decrease in mucin synthesis and an increase in gut permeability.[4] Understanding how these bacteria interact with the host gut microbiome is important because this informs the greater population which bacteria are necessary for a healthy microbial system.
Bacteria of the Gut Microbiome and Interactions with L-dopa
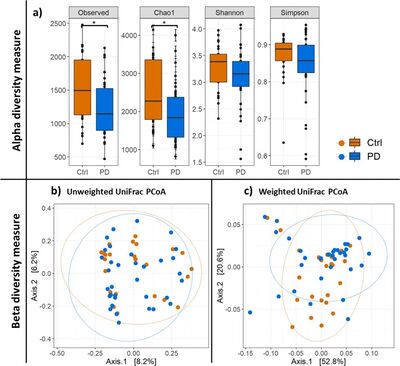
Medications prescribed for PD can have a multitude of different effects on patients with the disease. The medication most often prescribed for the treatment of Parkinson’s, levodopa, has been shown to cause alterations to the composition of gut microbiota.[9] A recent study done by Weis et al. (2019) used sequencing techniques to screen for differences in fecal microbiota composition when comparing PD patients and controls.[9] One of the most interesting findings the researchers established included an Alpha diversity index, which revealed a significant decrease in PD patient gut bacterial diversity when compared to the control group.[9] This could have much to do with the medications the patients are on in addition to individual food and diet preferences.
What is even more fascinating relates to the finding that PD patients treated with L-dopa managed to show much higher relative abundances of Clostridiales family XI, Enterococcaceae, and the genera Finegoldia and Peptoniphilus.[9] Clostridiales in particular tend to reduce inflammation and relieve patients of possible allergic diseases, so not having an abundant population of this bacteria proves to make the gut microbiome of a PD patient healthier.[10] However, bacteria like Enterococcaeceae can often deplete the GI tract of large swaths of protective commensals.[11] Specifically, the most common enterococcal species found in the gut microbiota are E. faecalis, E. faecium, E. durans, and E. hirae which can prove problematic as they are antibiotic resistant.[11] Further, the bacteria Finegoldia can also be difficult to deal with for PD patients since it is an opportunistic pathogen; these types of pathogens can cause disease when the host’s resistance is low, as would be the case in the gut microbiome of PD patients.[12] Finally, the heightened population gram-positive anaerobic cocci (GPAC) Peptoniphilus could also prove troublesome for PD patients as these anaerobic bacteria are isolated mainly from polymicrobial infections; this increases patients’ risk of more complications associated with the gut microbiome and PD.[13]
The research done by Weis et al. (2019) also proved that there were in fact lower levels of necessary bacteria like Ruminococcus gauvreauii and Faecalibacterium when compared to the control group.[9] The decreased level of these bacteria is detrimental to PD patients’ gut microbiome because Faecalibacterium is known to be highly prevalent in the healthy human gut microbiome.[14] It is found at lower levels in people with GI inflammatory diseases, which have been linked to PD.[14] Further, the reduction of Ruminococcus gauvreauii is problematic as well since they are one of the most important gut microbial mutualists.[15] As a marker of a healthy gut microbiome, Ruminococcus gauvreauii has been associated with many anti-inflammatory properties in the gut.[15] Many studies have examined the connection between the gut microbiome and PD, and the clinical, experimental, and epidemiological data all display that inflammation of the intestines can contribute to PD’s pathogenesis.[16] Even more studies suggest that PD may even begin in the gastrointestinal tract and system years prior to the development of motor symptoms.[16] Knowing this, patients with inflammatory bowel disease (IBD) are at a higher risk for developing PD when compared to individuals without IBD.[16] This is because there may be a genetic link between PD and IBD, as evidenced by gut inflammation’s ability to induce the loss of dopaminergic neurons.[16] These data suggest that the gut-brain axis is much more important than previously thought, and that the bacteria within the gut can have a positive or negative impact on the development of diseases and disorders.
The question then becomes: what do bacteria have to do with the bidirectional pathway between the gut and the brain? Researchers have used everything from germ-free (GF) animal models, infection studies, and intervention studies using antibiotics, prebiotics, and probiotics.[3] It has been thought that commensal bacteria have the ability to directly and indirectly influence the pathology of PD through the nervous (enteric and central) and circulatory systems.[3] A multi-tier network is involved in the GI tract and for neural communication; this network begins with enteric glial cells and the myenteric submucosal plexus.[3] Enteric glia are a vast population of peripheral neuroglia associated with processes of enteric neurons and cell bodies in the digestive tract[17], while the myenteric submucosal plexus (Auerbach’s plexus) is located between the circular and longitudinal muscle layers along most of the digestive tract.[18] A notable finding also includes the idea that catecholaminergic neurons, the neurons that contain the neurotransmitter dopamine which is implicated in PD,[19] are tightly placed by the opening of the bowels.[3] In addition, the vagus nerve (responsible for controlling digestion, the immune system, and heart rate[20]) innervates the myenteric plexus; the neurons at this location then lead to the prevertebral ganglia in the spinal cord and then further to the brain centers.[3] Finally, certain cytokines in the bloodstream have been shown to be increased in PD individuals’ serum, which then correlates to the progression of PD symptom severity.[3]
Current Research
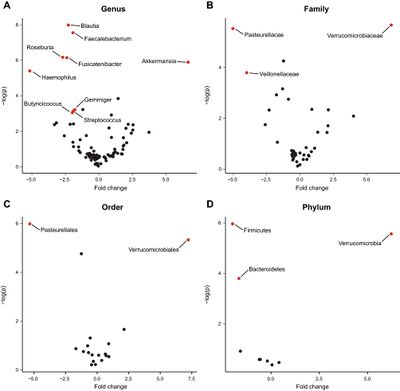
Current research regarding microbiome feedback in PD ranges from oral medication usage to the newest idea in the fight against Parkinson’s disease: device-assisted therapies (DATs).[6] In a study done by Lubomski et al. (2021), clinical data along with stool samples were collected from a total of 21 OD patients starting either levodopa-carbidopa intestinal gel (LCIG) or deep brain stimulation (DBS).[6] The control subjects for the study were ten healthy spousal subjects.[6] Using 16S amplicon sequencing, stool DNA was sequenced and allowed a comparison of gut microbiome stability between the groups.[6] Using a polymerase chain reaction, DNA integrity was confirmed and universal primers to the V3-V4 (341f and 805r) regions and entire rRNA gene (27f and 1492r) of the bacterial 16S ribosomal DNA were applied.[6] Researchers found altered gut microbiome compositions between the healthy and PD participants at varying taxonomic levels.[6] These differences ranged between participants undergoing DBS as this found an underrepresentation of Dorea and an overrepresentation of Pseudoflavonifractor, Clostridium_XlVa, Parabacteroides, and Bliophila.[6] For the participants undergoing LCIG therapy, there was an overrepresentation of Escherichia/Shigella and Pseudoflavonifractor, and an underrepresentation of Gemmiger.[6]
Overall, the only changes made with these new treatment options proved short-term changes within the gut microbiome after initiating PD DATs.[6] Before the DATs were introduced, PD-associated gut microbiome responses were observed and compared to changes after the respective DATs were started.[6] The results yielded many DAT-specific changes in the composition of the gut microbiome in both the DBS and LCIG options, meaning that DATs have the potential to influence the gut microbiome in Parkinson’s disease.[6] As the first acute-longitudinal study of the gut microbiome in relation to PD, the researchers were able to identify temporal stability in patients over a two-week pre-DAT initiation period and showed microbiota differences when comparing the results to healthy subjects.[6] Further, more variation in gut microbiome composition was evident in PD patients over an additional four week post-initiation sampling period.[6]
What is consistent with other studies of the gut microbiome in PD is that there was an overrepresentation of Verrucomicrobia because of Akkermansia in PD patient groupings due to the higher proportion of mucolytic communities; the organisms responsible for this occupy the gut at the mucosal lining and have stress-response strategies which increase due to fasting and periods of lowered gut motility.[6] This could relate to changes in the gut’s environment which are driven by turnover of mucin responsible for altering gut motility and contributing to PD pathology.[6] The overrepresentation of Akkermansia was consistent with upper GI dysfunction and constipation severity, while an underrepresentation of Gemmiger supports a PD-specific gut microbiome as it has never before been reported in other studies.[6] Future research should include a longer study with a larger sample size of the population, but with the addition of participants beginning apomorphine infusions since they are the third most commonly used DAT in PD.[6] Examining gut microbiome and clinical connections which result from beginning DATs with the use of shotgun metagenomic sequencing; this type of sequencing allows for a more detailed degree of taxonomic resolution, which provides greater insight into bacterial metabolic function.[6]
On the clinical side
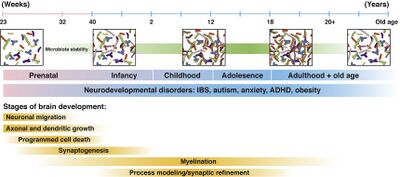
Demonstrating causal relationships in humans when it comes to the clinical implications of brain-gut disorders is challenging.[21] What is yet to be explored pertains to possible early life influences and how they have an impact on the development of the gut microbiome and brain connection network.[21] More specifically, preclinical studies have shown that there is a direct impact on gastrointestinal disorders correlated with a developmental component.[21] Changes in the gut-brain axis and their interactions have been suggested as disease mechanisms in not only Parkinson’s, but attention-deficit hyperactivity disorder (ADHD), Alzheimer’s disease, epilepsy, strokes, and autism spectrum disorder.[21] Translational studies have recently shown that transplantation of fecal microbiota from human donors with depression or anxiety can transmit some features or symptoms of their condition to mouse recipients.[21]
Other medical conditions, including irritable bowel syndrome (IBS) and obesity, have much to do with the gut microbiome.[21] Studies have reported microbial shifts in the fecal microbial community between IBS and healthy subjects.[21] IBS symptom severity has also been associated with dysbiosis, which relates to the dysbiosis of the gut microbiome in PD.[21] Stress has also been linked to a reduction in Lactobacilli in both preclinical and clinical studies; therefore, any IBS-related differences may represent changes of autonomic nervous system modulation of the gut microbiome.[21] In obesity, enteroendocrine cells interact with the distal gut and the gut microbiota along with metabolites then modulate eating behavior.[21] Preclinical studies have shown that fecal transplantation from hyperphagic obese mice to germ-free mice successfully induced weight gain and hyperphagic behavior.[21] Changes in brain microstructure in obesity have also been noted, as there a distinct microbial brain signatures with the ability to differentiate lean and obese subjects.[21] Subjects after bariatric surgery and undergoing fecal transplantation were shown to transmit the surgery’s weight loss effects to a germ-free non operated recipient, which reduced food intake and induced overall weight loss.[21] The gut microbiota clearly has many clinical implications even beyond PD. This research and information can be used to improve conventional therapies for disorders relating to the gut-brain relationship.[21]
Conclusion
More and more research is being done on the gut-brain axis in relation to Parkinson’s disease, and finding ways to alter the gut microbiome to include more of the essential bacteria flora earlier can potentially hinder the pathology of PD altogether. Understanding what causes dysbiosis of the gut microbiome, which medications can alter the bacteria within the gut, and being aware of even more clinical implications such as obesity and IBS/IBD can make more researchers interested in finding a less elusive connection between the brain and gut. Device-assisted therapies are a very new mode of exploring the gut microbiome and its connection to Parkinson’s disease. More studies comparing the use of DATs to medications like L-dopa are necessary because they can help develop new treatment options for patients with PD. It is important to keep in mind that much research is yet to be done on this topic, and more is definitely coming as conventional medicine and therapies are changing as new knowledge and opinions are being formed. With the help of new research and more information being made available about the bidirectional relationship between the gut and the brain, more people are aware of the implications their gut microbiota have on their overall and even neurological health.
References
- ↑ 1.0 1.1 Dauer, W. Przedborski, S. "Parkinson's disease: Mechanisms and Models" 2003. Neuron 39:889-909.
- ↑ 2.0 2.1 Elfil, M. Kamel, S. Kandil, M. Koo, B. Schaefer, S. "Implications of the Gut Microbiome in Parkinson's Disease" 2020. Movement Disorders 35:921-933.
- ↑ 3.00 3.01 3.02 3.03 3.04 3.05 3.06 3.07 3.08 3.09 3.10 3.11 3.12 3.13 3.14 3.15 Klann, E. Dissanayake, U. Gurrala, A. Farrer, M. Shukla, A. Ramirez-Zamora, A. Mai, V. Vedam-Mai, V. "The Gut–Brain Axis and Its Relation to Parkinson’s Disease: A Review" 2022. frontiers in Aging Neuroscience
- ↑ 4.00 4.01 4.02 4.03 4.04 4.05 4.06 4.07 4.08 4.09 4.10 4.11 4.12 4.13 Sun, M. Shen, Y. "Dysbiosis of gut microbiota and microbial metabolites in Parkinson’s Disease" 2018. Aging Research Reviews 45:53-61.
- ↑ 5.0 5.1 Gandhi, K. Saadabadi, A. "Levodopa (L-Dopa)" 2021. StatPearls.
- ↑ 6.00 6.01 6.02 6.03 6.04 6.05 6.06 6.07 6.08 6.09 6.10 6.11 6.12 6.13 6.14 6.15 6.16 6.17 6.18 Lubomski, M. Xu, X. Holmes, A. Yang, J. Sue, C. Davis, R. "The impact of device-assisted therapies on the gut microbiome in Parkinson’s disease" 2022. Journal of Neurology 269:780-795.
- ↑ 7.0 7.1 Humphreys, C. "19 - Intestinal Permeability" 2020. Textbook of Natural Medicine (Fifth Edition) 4:166-177.
- ↑ 8.0 8.1 8.2 8.3 Bhattarai, Y. Kashyap, P. "Parkinson’s disease: Are gut microbes involved?" 2020. American Journal of Physiology, Gastrointestinal and Liver Physiology.
- ↑ 9.0 9.1 9.2 9.3 9.4 Weis, S. Schwiertz, A. Unger, M. Becker, A. Fabender, K Ratering, S. Kohl, M. Schnell, S. Schafer, K. Egert, M. "Effect of Parkinson’s disease and related medications on the composition of the fecal bacterial microbiota" 2019. npj Parkinson's Disease 5.
- ↑ Guo, P. Zhang, K. Ma, X. He, P. "Clostridium species as probiotics: potentials and challenges" 2020. Journal of Animal Science and Biotechnology 11.
- ↑ 11.0 11.1 Dubin, K. Pamer, E. "Enterococci and their interactions with the intestinal microbiome" 2014. Microbiol Spectr 5(6).
- ↑ Neumann, A. Bjorck, L. Frick, I. "Finegoldia magna, an Anaerobic Gram-Positive Bacterium of the Normal Human Microbiota, Induces Inflammation by Activating Neutrophils" 2020. Frontiers in Microbiology 11:65.
- ↑ Kononen, E. "250 - Anaerobic Cocci and Anaerobic Gram-Positive Nonsporulating Bacilli" 2015. Mandell, Douglas, and Bennett's Principles and Practice of Infectious Diseases (Eighth Edition) 2:2781-2786.
- ↑ 14.0 14.1 Laursen, M. Laursen, R. Larnkjaer, A. Molgaard, C. Michaelsen, K. Frokiaer, H. Bahl, M. Licht, T. "Faecalibacterium Gut Colonization Is Accelerated by Presence of Older Siblings" 2017. mSphere 2:(6).
- ↑ 15.0 15.1 Reau, A. Suen, G. "The Ruminococci: key symbionts of the gut ecosystem" 2018. PubMed 3:199-208.
- ↑ 16.0 16.1 16.2 16.3 Brudek, T. "Inflammatory Bowel Diseases and Parkinson’s Disease" 2019. Journal of Parkinson's Disease 9:331-344.
- ↑ Seguella, L. Gulbransen, B. "Enteric glial biology, intercellular signalling and roles in gastrointestinal disease" 2021. Nature Reviews Gastroenterology & Hepatology 18:571-587.
- ↑ Orlando, L. Orlando, R. "Esophagus, Anatomy" 2004. Encyclopedia of Gastroenterology 763-766.
- ↑ Tamargo, J. Delpon, E. "54 - Pharmacologic Bases of Antiarrhythmic Therapy" 2014. Cardiac Electrophysiology: From Cell to Bedside (Sixth Edition) 529-540.
- ↑ "Vagus Nerve" 2022. Cleveland Clinic.
- ↑ 21.00 21.01 21.02 21.03 21.04 21.05 21.06 21.07 21.08 21.09 21.10 21.11 21.12 21.13 Osadchiy, V. Martin, C. Mayer, E. "The Gut–Brain Axis and the Microbiome: Mechanisms and Clinical Implications" 2019. Clinical Gastroenterology and Hepatology 17:322-332.
Authored for BIOL 238 Microbiology, taught by Joan Slonczewski, 2022, Kenyon College