User:Salmamashkoor1
Metabolism: The Productive Package of Catabolism and Anabolism
Metabolism is comprised of exergonic catabolic pathways and endergonic anabolic pathways, which are coupled as the products of catabolism act as starting materials for anabolism. The catabolic pathways are responsible for generating energy and reductant. Different substrates are fed into catabolic pathways, where they are oxidized. This oxidation utilizes the carbon source and energy provided by the substrate. Anabolic pathways carry out biosynthetic processes by using the energy produced by catabolism to create cell materials from nutrients in the environment1. As a consequence of microbial diversity, there exists a wide array of metabolic diversity that includes, but is not limited to, heterotrophic and lithotrophic metabolism. Heterotrophs use organic substrates as input for catabolism whereas lithotrophic metabolism feeds inorganic compounds into catabolic pathways. In addition to the various types of metabolism that could occur, the pathways may function under a range of oxygen conditions2.
Catabolism: The Driving Force of Metabolism
Catabolism provides key components for anabolism such as energy and reductant. The substrates fed into catabolism are oxidized to generate energy, which is usually available in the high-energy bonds of ATP. Other forms of energy used among cells are ultimately transformed into ATP. The reductants NAD+ and NADP+ are reduced to NADH and NADPH by accepting the electrons released by the oxidation of the substrate. The reductant cycled between catabolism and anabolism is mainly NADP+, which is reduced to NADPH in catabolic processes. NADPH is capable of carrying two protons and two electrons, which will be provided as input for anabolism. The NAD+ reductant participates solely in catabolism and is reduced to NADH to provide protons and electrons for catabolic respiration1.
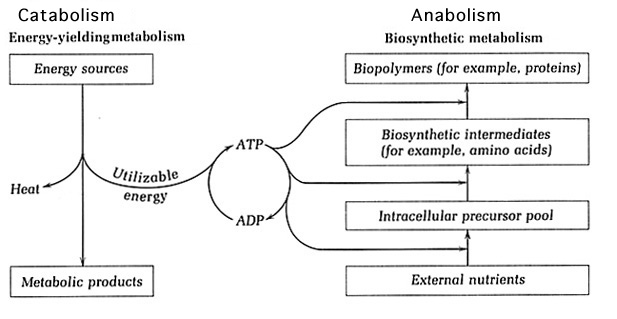
The Environment and Respiration
(1) Respiration
Respiration is the procedure by which all bacteria obtain energy1. It requires four key components to drive the complete oxidation of a substrate. These components include the tricarboxylic acid cycle (TCA cycle), the electron transport chain system (denoted by ETC), a terminal electron acceptor, and a transmembrane ATPase enzyme. The tricarboxylic acid cycle, also known as the citric acid cycle, is used when the substrate is an organic compound and thus is of relevance in heterotrophic metabolism. It is characterized by the complete oxidation of the organic substrate, which yields carbon dioxide and the release of electrons. The electron transport chain system is a series of electron carriers along the plasma membrane. The electron carriers take the electrons released by the TCA cycle and transport them from one electron carrier to the next, until the electrons are passed to the terminal electron acceptor. The terminal electron acceptor used depends on the type of oxygen relationship exercised by the microbe. No matter the oxygen relationship, the terminal electron acceptor will be reduced in the last step of the ETC as it accepts the electron that has been passed along between electron carriers. When the electrons enter the ETC, they are characterized by a very low redox potential. When the electrons leave the ETC they are characterized by a high redox potential. This increase in redox potential (E’o) indicates a release of energy that can be taken up by cells to produce ATP. The ETC also establishes a proton gradient as the electrons move through the system, which generates a proton motive force. The transmembranous ATPase enzyme produces ATP by utilizing the proton motive force across the membrane that is established by the ETC3.
(2) Environment and Oxygen Relationships
The oxygen available in the environment determines the method of respiration, which is demonstrated by obligate aerobes, obligate anaerobes, facultative anaerobes, microaerophilic bacteria, and aerotolerant bacteria. Thus respiration may operate under a number of conditions, depending on the substrates available in the environment. The oxygen relationship exhibited by the microorganism is determined by their oxygen consumption, which ultimately alters the oxygen environment2.
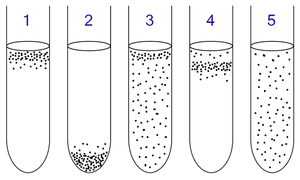
1. Obligate aerobic bacteria gather at the top of the test tube in order to absorb maximal amount of oxygen.
2. Facultative bacteria gather mostly at the top, since aerobic respiration is advantageous (ie, energetically favorable); but as lack of oxygen does not hurt them, they can be found all along the test tube.
3. Microaerophiles gather at the upper part of the test tube but not at the top. They require oxygen, but at a lower concentration.
4. Aerotolerant bacteria are not affected at all by oxygen, and they are evenly spread along the test tube. 4
Obligate Aerobe
Obligate aerobes live oxygen-abundant environments because these bacteria require O2 to respire. In the aerobic respiratory ETC, O2 behaves as the terminal electron acceptor as it is the last electron carrier in the system to accept the electrons being passed down the chain. When oxygen uptake is surpassed by oxygen consumption, the oxygen in the environment is available to the microorganism5. Thus the microorganism is capable of engaging in aerobic respiration by using O2 as the terminal electron acceptor.
Obligate Anaerobe
Obligate anaerobes are poisoned by the presence of O2 in the environment. In anaerobic respiration the electron acceptor may be an inorganic compound (such as SO4, S, NO3, NO2) or an organic compound like fumarate. When oxygen consumption is greater than oxygen uptake, then the oxygen in the environment becomes depleted and the microorganism carries out anaerobic respiration. Thus the microorganism uses a terminal electron acceptor other than O2. Commonly, microorganisms are able to use a variety of terminal electron acceptors other than O2 when anaerobic conditions are prevalent. This flexibility forms the foundation of favoritism for terminal electron acceptors. When the more favourable terminal electron acceptor is present in the environment for anaerobic bacteria, being the terminal electron acceptor that is correlated with the greatest growth, then this will be the terminal electron acceptor employed. If it is not present, then the next favorable and available terminal electron acceptor will be utilized in the ETC5.
Facultative Anaerobe
Facultative anaerobes can respire with or without O2. If O2 is present in the environment, then these bacteria will metabolize aerobically because O2 is the most favourable terminal electron acceptor. If O2 levels in the environment are depleted, then these bacteria will metabolize anaerobically5.
Microaerophilic Bacteria
Microaerophilic bacteria need O2 for metabolism as they cannot carry out anaerobic respiration. Thus microaerophilic bacteria are able to use O2 as a terminal electron acceptor. Though these bacteria are able to survive in the presence of O2, relatively high concentrations of O2 in the environment can be lethal. These bacteria grow in environments where the concentration of O2 is low, but oxygen is prevalent nonetheless5.
Aerotolerant Organisms
Aerotolerant bacteria respire anaerobically. Unlike other practicers of anaerobic respiration, aerotolerant bacteria are not poisoned by the presence of O2 in the environment. Thus they are found to be distributed evenly throughout O2 rich and O2 depleted environments5.
Heterotrophic Metabolism
Heterotrophic metabolism is comprised of fermentations and respirations. Heterotrophs include animals, fungi, protozoa, many bacteria, and a few fungi. This type of metabolism is characterized by the usage of organic compounds as input for catabolism. These compounds ultimately provide a carbon source and an energy source6.
(1) Fermentation
Fermentation is the process that gets energy by oxidizing an organic compound, usually a sugar like glucose, using organic electron donors and acceptors6. The ultimate outcome of this process is the production of ATP by substrate level phosphorylation. A common form of fermentation is carbohydrate catabolism, which yields pyruvate through the oxidation of glucose. These fermentation pathways begin with glucose, which is fed into a pathway of glycolysis. The Embden-Meyerhof pathway is one of three possible glycolysis pathways in bacteria7. The objective of any given glycolysis pathway is the dissimilation of sugars1.
Saccharomyces and lactic acid bacteria are some examples of bacteria that use the Embden-Meyerhof pathway for glycolysis. Saccharomyces, a genus including the species of yeast, utilize this pathway to generate energy, carbon dioxide, and ethanol from the oxidation of glucose. Lactic acid bacteria employ this pathway to generate lactic acid and energy. Many other bacteria make use of this pathway to produce an array of gases, alcohols, and fatty acids. The general function of the Embden-Meyerhof pathway is glucose dissimilation, which is demonstrated by the oxidation of glucose to two pyruvic acids1. The first three reactions are phosphorylation steps that result in fructose-1,6-diphosphate. The fructose-1,6-diphosphate is then cleaved by the enzyme fructose 1,6-diphosphate aldolase into two glyceraldehyde-3-phosphate triodes. Then each of the two triode-molecules undergoes oxidations and phosphorylations. The outcome of the modifications of a single triode molecule is the production of pyruvic acid. Subsequently each of the two pyruvic acids is transformed into pyruvate as they both undergo two substrate level phosphorylations, accompanied by the generation of two ATP. The overall yield of both the triode alterations in the Embden-Meyerhof pathway is the production of four ATP and two pyruvic acids, which will ultimately become two pyruvates through successive phosphorylations7. The objective of any given glycolysis pathway is the dissimilation of sugars1. The pyruvic acid product of the Embden-Meyerhof pathway can enter a number of consequential pathways that will reduce the pyruvic acid in different ways. Lactic acid bacteria undergo an additional step in which they reduce the pyruvate to lactic acid. Yeast undergo an additional step in which they reduce pyruvate to ethanol and carbon dioxide. The preceding pathway used to reduce the pyruvate will determine the types of products generated by the Embden-Meyerhof pathway8.
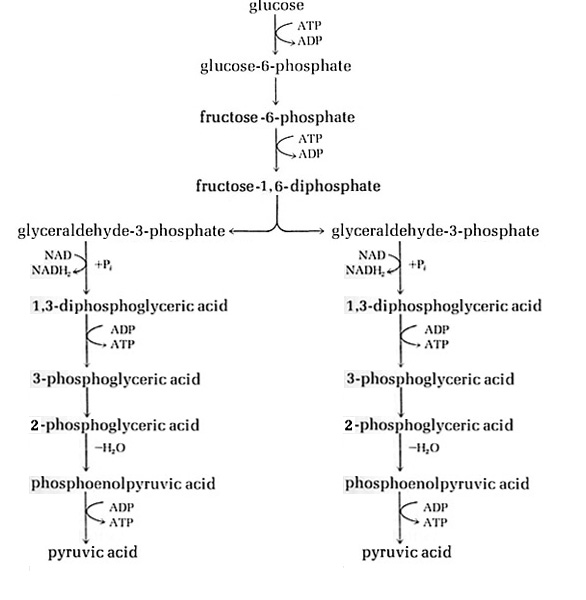
(2) Respiration
Aerobic heterotrophic respiration of glucose begins with the input of an organic substrate (like glucose) that is completely oxidized to carbon dioxide by glycolysis and the TCA cycle. The electrons released from this process are taken up by NAD+, converting the reductant into NADH, which feeds the electrons into the ETC3. The ETC moves these electrons towards the terminal electron acceptor of O2, establishing a proton motive force along the membrane in the process. The terminal electron acceptor (O2) is reduced to H2O as it accepts the electrons2. The overall net reaction of aerobic respiration of glucose is as follows1:
Glucose + 6O2 → 6CO2 + H2O
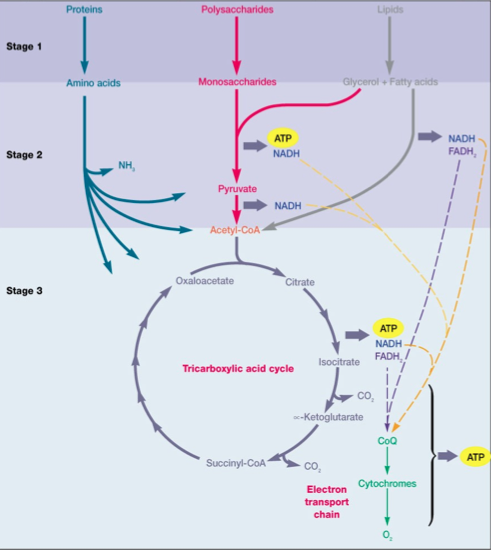
Lithotrophic Metabolism
Lithotrophic metabolism uses an inorganic substrate as an input for catabolism, and may also govern under various oxygen conditions. The general process remains the same as electrons are removed from the inorganic substrate by means of oxidation and introduced into the ETC to produce energy in the form of ATP9. Most lithotrophs use carbon dioxide as a carbon source. Facultative lithotrophs are capable of using organic compounds in addition to other energy sources. Examples of lithotrophs include hydrogen bacteria and methanogens1.
(1) Hydrogen Bacteria
Hydrogen bacteria are facultative lithotrophs because they use H2, an inorganic substrate, as input for catabolism. As facultative lithotrophs, hydrogen bacteria are also able to use an organic substrate for catabolism, but if H2 is available in the environment it will be utilized as the most favoured substrate. The catabolic pathways require hydrogenases to oxidize H2 for energy9. They are categorized into two groups, distinguished by their oxygen relationships: Microaerophiles and obligate aerobes.
Microaerophilic Hydrogen Bacteria
The first group is characterized by oxidizing H2 under microaerophilic conditions, indicating that these bacteria are capable of surviving in the presence of oxygen, but are unable to survive in relatively high concentrations of oxygen. The microaerophilic property is demonstrated as O2 is employed as the terminal electron acceptor of the ETC. Furthermore, the activity of the hydrogenases (oxidization of H2) is inhibited by O2 but O2 is still used as a terminal electron acceptor. Chemotrophic and phototrophic bacteria are common examples of this category of hydrogen bacteria9.
Obligate Aerobic Hydrogen Bacteria
The second group use hydrogen for aerobic respiration and the reduction of the terminal electron acceptor (O2). Alcaligenes eutrophus are an example of these types of hydrogen bacteria as they use H2 as an electron donor under aerobic conditions. The aerobic hydrogen-oxidizing bacteria can have two types of hydrogenases to activate or release hydrogen: a NAD-reducing soluble enzyme or the membrane bound enzyme, which is unable to reduce pyridine nucleotides. Alcaligenes eutrophus, A. ruhlandii, and Pseudomonas saccharophila have both types of hydrogenases. Nocardia opaca, along with other species of the Nocardia genus, only have the NAD-reducing enzyme. The majority of hydrogen-oxidizing bacteria only have a membrane-bound hydrogenase. These bacteria include Alicaligenes latus, Aquaspirillum autotrophicum, Paracoccus dentrificans, many species of the Pseudomonads genus (not including Pseudomonas saccharopila), and the coryneform nitrogen-fixing strains9.
(2) Methanogens
Methanogens oxidize a range of substrates (including H2 + CO2, methanol, methylamines, and acetate) into methane in a process known as methanogenesis9. The process of methanogenesis associates with different complexes of coenzymes, each specific for the oxidation of particular substrates. When methanogenesis uses the H2 + CO2 substrate, it oxidizes H2 and uses the electrons released from this process to reduce CO2. The required coenzymes for this procedure are the C1 intermediates, which are a type of carrier-bound enzyme. These enzymes guide the stepwise reduction of CO2 to methane using the electrons released by the oxidation of H2. When methanogenesis uses methanol and methylamine substrates to form methane, a portion of the methyl groups is oxidized to CO2. This generates reducing equivalents that are used to reduce other methyl groups to methane. The coenzymes required for this are the C1 intermediates used in the methanogensis of CO2. When methanogenesis converts acetate to methane and CO2, the first thing that is required is the activation of acetyl-CoA, which is then cleaved. This cleavage produces a coenzyme-bound methyl moiety and an enzyme-bound carbonyl group. The carbonyl group is oxidized to carbon dioxide equivalents, which are then used to reduce the methyl moiety to methane. All forms of methanogenesis produce transmembrane ion gradients. Transmembranous ATPase enzymes utilize the ion gradient produced by methanogenesis to synthesize ATP10.
Anabolism: Biosynthetic Processes that rely on Catabolic Products
Catabolism oxidizes substrates, whether they are organic (heterotrophic) or inorganic (lithotrophic), and uses them throughout respiratory processes to generate energy. This may occur in an aerobic or anaerobic fashion, but the general outcome remains the same. The electrons and protons generated are carried by the NADPH reductant into anabolic pathways to deliver electrons and protons for biosynthesis. The ATP energy produced is used in endergonic anabolic pathways to create cell materials. Using these products of catabolism, the anabolic pathways are able to build a variety of monomers that will later be incorporated into macromolecules11.
References
- JM, Lane; Brighton CT, BJ Menkowitz (1977). "Anaerobic and Aerobic Metabolism in Articular Cartilage". The Journal of Rheumatology 4 (4): 344. PMID 604473.
- M., Blaut (1994). "Metabolism of Methanogens". Institut Fur Mikrobiologie. 66 3 (1): 187. PMID 7747931. Retrieved 27 October 2013.
- Schink, Bernhard; Schlegel (1978). "Hans-Gunter". Biochimie 60: 297. PMID 667183. Retrieved 27 October 2013.
- "Microaerophile". Wikipedia. Retrieved 21 November 2013.
- Haddock, Bruce; Cline Jones (1977). "Bacterial Respiration". Bacterbiological Reviews 41 (1): 47. PMID 413996. Retrieved 25 October 2013.
- JB, Russell; GM, Cook (1995). "Energetics of bacterial growth: Balance of Anabolic and Catabolic Reactions". Microbial Rev 59 (1): 48. PMID 7708012. Retrieved 27 October 2013.
- Ostergaard, Simon; L. Olsson, J. Nielsen (2000). "Metabolic engineering of Saccharomyces cerevisiae". Microbiol. MolBiol. Rev 64: 34. PMID 98985.
- Zablotny, R.; DG. Fraeknkel (1967). "Glucose and Gluconate Metabolism in a Mutant of Escherichia coli Lacking Gluconate-6-phosphate Dehydrase". Journal of Bacteriology 1579. PMID 276652. Retrieved 26 October 2013.
- Todar, Kenneth. "Diversity of Microbial Metabolism". University of Wisconsin-Madison. Retrieved 25 October 2013.
- DP, Kelly (1971). "Autotrophy: Concepts of Lithotrophic Bacteria and Their Organic Metabolism". Annu Rev Microbiol 25: 177. PMID 4342704. Retrieved 27 October 2013.
- Jurtshuk, P Jr. (1996). "Bacterial Metabolism". Medical Microbiology 4. PMID NBK7917.