Bioremediation: Difference between revisions
(38 intermediate revisions by 5 users not shown) | |||
Line 1: | Line 1: | ||
{{Curated}} | {{Curated}} | ||
Through agriculture, industry, and daily life, harmful chemicals have been released into the earth’s air, soil, and water. Depending on | Through agriculture, industry, and daily life, harmful chemicals have been released into the earth’s air, soil, and water. Depending on their concentrations, these substances can have destructive consequences on ecosystems, as well as cause severe damage to humans and other organisms nearby. Soil pollution is of special importance because of its impact on surface, groundwater and air contamination and can easily spread and be consumed by humans. | ||
[[Image:Bioremediation_images.jpeg|upright=3|thumb|Retrieved from Tiedje, J. M. (1993). Bioremediation from an ecological perspective. In situ bioremediation: When does it work, 110-120.]] | |||
<b>Biodegradation</b> is the biologically catalyzed | <b>Biodegradation</b> is the biologically catalyzed modification of an organic chemical's structure. However, this modification can be through different metabolic pathways and does not necessarily mean a reduction in toxicity. Mineralization, one type of biodegradation, is defined as the conversion of an organic substance to its inorganic constituents, rendering the original compound harmless. [23]. Transformation is defined as any metabolically-induced change in the chemical composition of a compound [14]. | ||
<b>Bioremediation</b> refers to the use of microorganisms to degrade contaminants that pose environmental and human risks. Bioremediation processes typically involve the actions of many different microbes acting in parallel or sequence to complete the degradation process. Both in situ (in place) and ex situ (removal and treatment in another place) remediation approaches are used. The versatility of microbes to degrade a vast array of pollutants makes bioremediation a technology that can be applied in different soil conditions [3]. Though it can be inexpensive and in situ approaches can reduce disruptive engineering practices, bioremediation is still not a common practice [1]. | |||
A widely used approach to bioremediation involves stimulating naturally occurring microbial communities, providing them with nutrients and other needs, to break down a contaminant. This is termed <b>biostimulation.</b> Biostimulation can be achieved through changes in pH, moisture, aeration, or additions of electron donors, electron acceptors or nutrients. Another bioremediation approach is termed <b>bioaugmentation</b>, where organisms selected for high degradation abilities are used to inoculate the contaminated site [3]. These two approaches are not mutually exclusive- they can be used simultaneously. | |||
Recent awareness of the dangers of many chemicals used in society has led to research on formulation of products that are more easily degraded in the environment. | |||
From an ecological point of view, bioremediation depends on the various interactions between three factors: substrate (pollutant), organisms, and environment, as shown in the figure at right [4]. The interactions of these factors affect biodegradability, bioavailability, and physiological requirements, which are important in assessing the feasibility of bioremediation [4]. <b>Biodegradability</b>, or whether a chemical can be degraded or not, is determined by the presence or absence of organisms that are able to degrade a chemical of interest and how widespread these organisms are in the site [4]. The substrate (pollutant) can interact with its surrounding environment to change its <b>bioavailability</b>, or availability to organisms that are capable of degrading it; for example, substrate has low bioavailability if it is tightly bound to soil organic matter or trapped inside aggregates [4]. <b>Physiological requirements</b>, or set of conditions required by organisms to carry out bioremediation in the environment, include nutrient availability, optimal pH, and availability of electron acceptors, such as oxygen and nitrate [4]. Also, the environment needs to be habitable for organisms involved in bioremediation [4]. | From an ecological point of view, bioremediation depends on the various interactions between three factors: substrate (pollutant), organisms, and environment, as shown in the figure at right [4]. The interactions of these factors affect biodegradability, bioavailability, and physiological requirements, which are important in assessing the feasibility of bioremediation [4]. <b>Biodegradability</b>, or whether a chemical can be degraded or not, is determined by the presence or absence of organisms that are able to degrade a chemical of interest and how widespread these organisms are in the site [4]. The substrate (pollutant) can interact with its surrounding environment to change its <b>bioavailability</b>, or availability to organisms that are capable of degrading it; for example, substrate has low bioavailability if it is tightly bound to soil organic matter or trapped inside aggregates [4]. <b>Physiological requirements</b>, or set of conditions required by organisms to carry out bioremediation in the environment, include nutrient availability, optimal pH, and availability of electron acceptors, such as oxygen and nitrate [4]. Also, the environment needs to be habitable for organisms involved in bioremediation [4]. | ||
Line 65: | Line 64: | ||
A majority of heavy metal pollutants come from human sources that accumulate over time. | A majority of heavy metal pollutants come from human sources that accumulate over time. | ||
There are natural forms of contamination from normal biological processes which include: | There are also natural forms of contamination from normal biological processes, which include: | ||
1. Weathering of minerals over time | 1. Weathering of minerals over time | ||
2. Erosion and volcanic activities | 2. [https://en.wikipedia.org/wiki/Erosion Erosion] and [https://en.wikipedia.org/wiki/Volcano volcanic activities] | ||
3. Forest fires and biogenic source | 3. [https://en.wikipedia.org/wiki/Wildfire Forest fires] and biogenic source | ||
4. Particles released by vegetation | 4. Particles released by vegetation | ||
Heavy metals can be absorbed by microbes at cellular binding sites. Extracellular polymers of these microbes can complex heavy metals through various mechanisms [21]. These specialized microorganisms can mineralize the organic contaminants to metabolic intermediates which are used as primary substrates for cell growth. The microbes prevalent in heavily metal-contaminated soil can alter the oxidation of the heavy metals by immobilizing them [21], allowing them to be easily removed. Bioremediation of heavy metals from microbes is not heavily researched, mostly due to an incomplete understanding of the genetics of the microbes used in metal adsorption. ''[https://microbewiki.kenyon.edu/index.php/Geomicrobiology Geomicrobiology]'' takes a better look at the interactions between microbes and inorganic material. | Heavy metals can be absorbed by microbes at cellular binding sites. Extracellular polymers of these microbes can complex heavy metals through various mechanisms [21]. These specialized microorganisms can mineralize the organic contaminants to metabolic intermediates, which are used as primary substrates for cell growth. The microbes prevalent in heavily metal-contaminated soil can alter the oxidation state of the heavy metals by immobilizing them [21], allowing them to be easily removed. Bioremediation of heavy metals from microbes is not heavily researched, mostly due to an incomplete understanding of the genetics of the microbes used in metal adsorption. ''[https://microbewiki.kenyon.edu/index.php/Geomicrobiology Geomicrobiology]'' takes a better look at the interactions between microbes and inorganic material. | ||
=='''Organisms'''== | =='''Organisms'''== | ||
Line 114: | Line 113: | ||
===='''Biodegradation Capacities of White rot fungi'''==== | ===='''Biodegradation Capacities of White rot fungi'''==== | ||
Using fungi as potential treatment of contaminants began in 1985 when the white rot species Phanerochaete chrysosporium was discovered to metabolize multiple key environmental pollutants. The most important feature of these fungi is their enzymatic functional ability to metabolize complex chemicals such as lignin. Similar abilities were later discovered in other white rot fungal species. In addition, white rot fungi are highly advantageous because they degrade lignin extracellularly through its hyphal extension. This allows them to access soil contaminants that other organisms are incapable of and maximize surface area for enzymatic interaction. These inexpensive fungi can tolerate extreme environmental conditions, such as pH, temperature, and moisture content. While many microbial organisms that are used for bioremediation require pre-conditioning of the environment for them to survive in, white rot fungi can directly be applied into most systems because they degrade based upon nutrient deprivation. [18] | Using fungi as potential treatment of contaminants began in 1985 when the white rot species Phanerochaete chrysosporium was discovered to metabolize multiple key environmental pollutants. The most important feature of these fungi is their enzymatic functional ability to metabolize complex chemicals such as lignin. Similar abilities were later discovered in other white rot fungal species. In addition, white rot fungi are highly advantageous because they degrade lignin extracellularly through its hyphal extension. This allows them to access soil contaminants that other organisms are incapable of and maximize surface area for enzymatic interaction. These inexpensive fungi can tolerate extreme environmental conditions, such as pH, temperature, and moisture content. While many microbial organisms that are used for bioremediation require pre-conditioning of the environment for them to survive in, white rot fungi can directly be applied into most systems because they degrade based upon nutrient deprivation. [18] | ||
[[Image:040504062021.jpg|right|thumb|Scanning electron micrograph (SEM) depicts ''Phanerochaete chrysosporium'' fungi; Mag. .5x]] | |||
===='''''[[Phanerochaete chrysosporium]]'''==== | ===='''''[[Phanerochaete chrysosporium]]'''==== | ||
<i>P. chrysosporium</i> was the first fungi linked to degradation of organic pollutants. Extensive research has show this it has strong potential for bioremediation in pesticides, PAHs, dioxins, carbon tetrachloride, and many other pollutants. Among fungal systems, <i>P. chrysosporium</i> has become the model for bioremediation. Other notable species of white rot fungi include <i>Pleurotus ostreatus</i> and <i>Trametes versicolor</i>. [18] | <i>P. chrysosporium</i> was the first fungi linked to degradation of organic pollutants. Extensive research has show this it has strong potential for bioremediation in pesticides, PAHs, dioxins, carbon tetrachloride, and many other pollutants. Among fungal systems, <i>P. chrysosporium</i> has become the model for bioremediation. Other notable species of white rot fungi include <i>Pleurotus ostreatus</i> and <i>Trametes versicolor</i>. [18] | ||
===='''Bioremediation of Hydrocarbon Pollutants'''==== | ===='''Bioremediation of Hydrocarbon Pollutants'''==== | ||
Line 140: | Line 139: | ||
The role of archaea in bioremediation has not been studied as commonly as that of bacteria [10]. Nevertheless, numbers of researchers have shown their ability to degrade various pollutants and scientists began to discover more about their potential in participating in bioremediation. Below lists some important facts regarding archaea’s potential role in bioremediation. | The role of archaea in bioremediation has not been studied as commonly as that of bacteria [10]. Nevertheless, numbers of researchers have shown their ability to degrade various pollutants and scientists began to discover more about their potential in participating in bioremediation. Below lists some important facts regarding archaea’s potential role in bioremediation. | ||
- Biodegradation by extreme halophilic archaea was not recognized widely in the past, but scientists have found out that extreme halophilic archaea have greater catabolic diversity than expected [9] | - Biodegradation by extreme [https://en.wikipedia.org/wiki/Halophile halophilic] archaea was not recognized widely in the past, but scientists have found out that extreme halophilic archaea have greater catabolic diversity than expected [9] | ||
- Hydrocarbon-contamination is observed in some extreme environments, including hypersaline (high salt concentration), high or low temperature, or extreme pH [10]. Archaea’s adaptation to extreme environment gives them the potential to participate in biodegradation and bioremediation in these environments; in fact, microorganisms naturally adapted to the cold environments are known to be important degraders of hydrocarbons in those environments [10]. | - Hydrocarbon-contamination is observed in some extreme environments, including hypersaline (high salt concentration), high or low temperature, or extreme pH [10]. Archaea’s adaptation to extreme environment gives them the potential to participate in biodegradation and bioremediation in these environments; in fact, microorganisms naturally adapted to the cold environments are known to be important degraders of hydrocarbons in those environments [10]. | ||
Line 148: | Line 147: | ||
- Some archaea are known to be resistant to variety of antibiotics, including penicillin, cycloheximide, streptomycin, etc, which gives them great advantage in participating in bioremediation in the presence of antibiotics [5]. | - Some archaea are known to be resistant to variety of antibiotics, including penicillin, cycloheximide, streptomycin, etc, which gives them great advantage in participating in bioremediation in the presence of antibiotics [5]. | ||
====''' | ===='''Examples of studies of Archaea involved in bioremediation'''==== | ||
Four extreme halophilic strains of archaea (belonging to genus ''[https://en.wikipedia.org/wiki/Halobacterium Halobacterium]'', ''[https://en.wikipedia.org/wiki/Haloferax Haloferax]'', and ''[https://en.wikipedia.org/wiki/Halococcus Halococcus]'') were studied to evaluate their potential to biodegrade crude oil and hydrocarbons. [5] All four strains could use various kinds of hydrocarbons as their carbon or energy sources [5]. Two strains of Haloferax grew on n-alkanes with different lengths, ranging from C8 to C34, and also benzene, toluene, biphenyl, and naphthalene. The research demonstrated the important fact that archaea have potential to carry out biodegradation at high temperatures, in the range of 40-45 °C [5], which is advantageous because hydrocarbons have higher solubility and bioavailability at these higher temperature [10]. The four strains studied were resistant to six different antibiotics, including penicillin, streptomycin, cycloheximide [5] and this gave them the potential to carry out biodegradation in conditions unfavorable for bacteria. Research suggests other genera of archaea are also capable of biodegrading in hypersaline environments [6] | |||
''[https://en.wikipedia.org/wiki/Halococcus Archaeglobus] fulgidus'', a [https://en.wikipedia.org/wiki/Hyperthermophile hyperthermophile] which can use sulfate as an electron acceptor, can also break down various aromatic hydrocarbons (Peeples, 2014). | |||
''[https://en.wikipedia.org/wiki/Halococcus Archaeglobus] fulgidus'', a [https://en.wikipedia.org/wiki/Hyperthermophile hyperthermophile] | |||
=='''Microbial Processes'''== | =='''Microbial Processes'''== | ||
Line 168: | Line 161: | ||
A greater amount of substituents will cause slower degradation in aerobic environments, but faster degradation in anaerobic ones. Chlorine makes a molecule less degradable due to steric hindrance preventing access to necessary enzymes, therefore molecules with higher chlorination are slower to degrade in aerobic conditions. High concentration of a pollutant generally results in faster rates of degradation. If the concentration drops below a threshold concentration, the enzymes may not detect it and will cease to degrade it [26]. | A greater amount of substituents will cause slower degradation in aerobic environments, but faster degradation in anaerobic ones. Chlorine makes a molecule less degradable due to steric hindrance preventing access to necessary enzymes, therefore molecules with higher chlorination are slower to degrade in aerobic conditions. High concentration of a pollutant generally results in faster rates of degradation. If the concentration drops below a threshold concentration, the enzymes may not detect it and will cease to degrade it [26]. | ||
Soil with small pores, especially clays, may cause biodegradation to take years due to the decrease in bioavailability. Chlorine makes a molecule less degradable due to steric hindrance preventing necessary enzymes from accessing the compound, therefore molecules with higher chlorination are slower to degrade. | Soil with small pores, especially clays, may cause biodegradation to take years due to the decrease in bioavailability. Chlorine makes a molecule less degradable due to steric hindrance preventing necessary enzymes from accessing the compound, therefore molecules with higher chlorination are slower to degrade. | ||
The power rate model | The rate at which a compound is transformed, as well as the curves that describe its transformation, is referred to as kinetics, and is affected by all factors listed above. First order kinetics (exponential decay) often describes biodegradation when the initial substrate concentration is low, while zero-order kinetics (linear biodegradation) is often observed when the substrate concentration is very high. In some cases if the concentration of the chemical falls below a critical threshold concentration, the microbes can no longer transform it and the chemical persists. | ||
The power rate model depicting the relationship between concentration and rate of degradation (first order decay here) is as follows: | |||
-dC/dt = kC^n | -dC/dt = kC^n | ||
Line 180: | Line 173: | ||
==='''Primary substrate utilization'''=== | ==='''Primary substrate utilization'''=== | ||
<b>Primary substrate utilization</b> occurs when a microbe both transforms a substrate and uses it as an energy or carbon source. [15] An electron acceptor is required for these transformations. It can be anaerobic or aerobic, although the presence of oxygen tends to speed up reactions. This | <b>Primary substrate utilization</b> occurs when a microbe both transforms a substrate and uses it as an energy or carbon source. [15] An electron acceptor is required for these transformations. It can be anaerobic or aerobic, although the presence of oxygen tends to speed up reactions. This type of biodegradation is common in break down of petroleum compounds and some pesticides. | ||
==='''Cometabolism (Secondary Substrate Utilization)'''=== | ==='''Cometabolism (Secondary Substrate Utilization)'''=== | ||
<b>Cometabolism</b> involves the transformation of a chemical by an organism while the organism uses a different substance as its primary energy or carbon source [14] | <b>Cometabolism</b> involves the fortuitous transformation of a chemical by an organism while the organism uses a different substance as its primary energy or carbon source [14]. During the actual reaction degrading the substance, it appears that the organism involved has no net carbon or energy gain, and may even result in a product which is toxic to the cell [14]. | ||
A key example of cometabolism is fortuitous metabolism in the degradation of trichloroethylene, shown in the diagram below. An organic growth substrate such as propane or butane is required for the enzymatic activity that transforms TCE. [14] | A key example of cometabolism is fortuitous metabolism in the degradation of trichloroethylene, shown in the diagram below. An organic growth substrate such as propane or butane is required for the enzymatic activity that transforms TCE. [14] | ||
Line 218: | Line 211: | ||
===='''Biopiling'''==== | ===='''Biopiling'''==== | ||
Excavated soils are mixed with soil amendments and placed on a treatment area. Biopiles are aerated with the use of perforated pipes and blowers in order to control the progression of biodegradation more efficiently by controlling the supply of oxygen [29], which in turn may affect other factors such as pH. This system is primarily used to remediate systems with oil and hydrocarbon contamination. The remediated soil is placed in a liner to prevent further contamination of the soil, they may also be covered with plastic to control runoff, evaporation, and volatilization. | Excavated soils are mixed with soil amendments and placed on a treatment area. Biopiles are aerated with the use of perforated pipes and blowers in order to control the progression of biodegradation more efficiently by controlling the supply of oxygen [29], which in turn may affect other factors such as pH. This system is primarily used to remediate systems with oil and hydrocarbon contamination. The remediated soil is placed in a liner to prevent further contamination of the soil, they may also be covered with plastic to control runoff, evaporation, and [https://en.wikipedia.org/wiki/Volatilisation volatilization]. | ||
===='''Composting'''==== | ===='''Composting'''==== | ||
Line 227: | Line 220: | ||
===='''Biostimulation'''==== | ===='''Biostimulation'''==== | ||
This method involves the addition of nutrients to a polluted site in order to encourage the growth of naturally occurring chemical-degrading microorganisms[31]. Biostimulation is primarily done by the addition of various nutrients that are limited in the soil | This method involves the addition of nutrients to a polluted site in order to encourage the growth of naturally occurring chemical-degrading microorganisms[31]. Biostimulation is primarily done by the addition of various nutrients that are limited in the soil as well as electron acceptors, such as phosphorus, nitrogen and oxygen, or increasing the amount of available carbon in order to increase the population or activity of naturally occurring microorganisms. Other approaches are to optimize environmental conditions such as aeration, the addition of nutrients, altering pH and temperature control [32]. The primary advantage of biostimulation is that it is done by native microorganisms that are well-suited to the environment, and are already well distributed spatially. The challenge is delivering additives so they are readily available to the subsurface microbes. | ||
===='''Metal Biosorption'''==== | ===='''Metal Biosorption'''==== | ||
Adsorption of metals and other ions of an aqueous solution by the use of microbes. The biosorption process involves a solid phase and a liquid phase containing a dissolved species to be sorbed [34]. The process continues | Adsorption of metals and other ions of an aqueous solution by the use of microbes. The biosorption process involves a solid phase and a liquid phase containing a dissolved species to be sorbed [34]. The process continues until equilibrium is established between the amount of solid-bound sorbate species and its portion remaining in the solution. The degree of affinity for the sorbate determines its distribution between the solid and liquid phases. | ||
Biosorption processes are | Biosorption processes are very important in the environment, and has been utalized for conventional biotreatment processes. Biosorption is primarily aimed at the removal or recovery of organic and inorganic substances from solution [35]. The commercialization of biosorption technologies has been limited so far. | ||
[[Image:Bioventing.png|right|upright=2.5|thumb|Bioventing is primarily used for injecting air into specific remediation zones, adding oxygen as a readily available electron acceptor where it would otherwise be anaerobic. It can also be reversed to make a more anaerobic environment. Either technique can be applied depending on the remediating microbes would thrive in [36].]] | [[Image:Bioventing.png|right|upright=2.5|thumb|Bioventing is primarily used for injecting air into specific remediation zones, adding oxygen as a readily available electron acceptor where it would otherwise be anaerobic. It can also be reversed to make a more anaerobic environment. Either technique can be applied depending on the remediating microbes would thrive in [36].]] | ||
===='''Bioventing'''==== | ===='''Bioventing'''==== | ||
Bioventing is an In | Bioventing is an In situ remediation technology that uses indigenous microorganisms to biodegrade organic constituents adsorbed to soils in the unsaturated zone[36]. The availability of oxygen generally controls the rate at which aerobic bioremediation proceeds. Bioventing is the coupling of soil venting and bioremediation. Bioventing can be successfully applied to compounds ranging from gasoline or diesel, to heavier hydrocarbons[36]. The addition of nutrients with the bioventing flow rates can achieve greater contaminant reductions than venting alone. | ||
==='''Ex-situ or In-situ'''=== | ==='''Ex-situ or In-situ'''=== | ||
Line 246: | Line 236: | ||
===='''Bioaugmentation'''==== | ===='''Bioaugmentation'''==== | ||
Bioaugmentation is the addition of non-native microorganisms | Bioaugmentation is the addition of non-native microorganisms that have the ability to degrade the contaminants that are recalcitrant to the indigenous microbiota. Bioaugmentation has been proven successful in cleaning organic pollutant, but still faces many environmental problems, such as the survival of strains introduced to soil[37]. The number of introduced microorganisms usually decreases shortly after soil inoculation. | ||
Bioaugmentation is ideal for soil: | Bioaugmentation is ideal for soil: | ||
Line 254: | Line 244: | ||
2. Containing compounds requiring multi stepped remediation. | 2. Containing compounds requiring multi stepped remediation. | ||
Augmentation techniques have a great potential for [https://en.wikipedia.org/wiki/Category:Aromatic_compounds aromatic compound] remediation. The most important step in successful bioaugmentation is selection of proper microbial strains. The success of bioaugmentation strongly depends on the ability of inoculants to survive in contaminated soil. | Augmentation techniques have a great potential for [https://en.wikipedia.org/wiki/Category:Aromatic_compounds aromatic compound] remediation. The most important step in successful bioaugmentation is selection of proper microbial strains. The success of bioaugmentation strongly depends on the ability of inoculants to survive in contaminated soil, which may vary due to predation and an environment that does provide all the conditions and nutrients that the organism needs to survive. In some cases the environment may be toxic to the added organism. | ||
===='''Land Farming'''==== | ===='''Land Farming'''==== | ||
Contaminated soil is mixed with amendments such as nutrients, and then they are tilled into the earth or | Contaminated soil is mixed with amendments such as nutrients, and then they are tilled into the earth, or the contaminated soil is applied into lined beds and periodically turned over or tilled to aerate the waste [38]. The topmost layer is the area of concentration for this method, so it is not ideal for deeper remediation. Land farming differs from composting because it actually incorporates contaminated soil into soil that is uncontaminated [38]. The higher zone of remediation will typically contain primarily lighter hydrocarbons that can be volatilized. The material is periodically tilled for aeration to hasten remediation of any nutrients and allow more oxygen to act as electron acceptors, as well as allowing volatilization to occur. Contaminants are degraded, transformed, and immobilized by microbiological processes and oxidation. Soil conditions are controlled to optimize the rate of contaminant degradation, moisture content, frequency of aeration, and pH are all conditions that may be controlled [38]. | ||
[[Image:Biofilter.png|right|upright=1.5|thumb|The application of a micro-algal/bacterial biofilter in the primary outflow of soil water [39]]] | [[Image:Biofilter.png|right|upright=1.5|thumb|The application of a micro-algal/bacterial biofilter in the primary outflow of soil water [39]]] | ||
Line 267: | Line 257: | ||
==='''Advantages'''=== | ==='''Advantages'''=== | ||
1. Bioremediation is a publicly accepted treatment of polluted soil because it is based upon natural processes. Microbes that metabolize contaminants increase in population when the contaminant is present. | 1. Bioremediation that involves natural attenuation or biostimulation is a publicly accepted treatment of polluted soil because it is based upon natural processes. Microbes that metabolize contaminants often increase in population when the contaminant is present and thus rates of biodegradation may increase over time, up to a point. If biodegradation is complete (i.e. mineralization) the products from treatment are harmless; such as carbon dioxide, water, and cellular biomass. [12] | ||
2. | 2. In situ bioremediation can result in complete degradation of pollutants into harmless products on site. This removes the risks involved with transportation for treatment and elimination of contaminated substances. [12] | ||
3. Bioremediation | 3. Bioremediation can be a cheaper alternative to other technologies used for pollution mitigation. [12] | ||
==='''Disadvantages'''=== | |||
1. Only biodegradable compounds are capable of undergoing bioremediation. Not every compound is capable of fully degrading quickly. [12] | |||
2. The products of biodegradation may potentially be even more persistent or toxic than the original contaminant. [12] | |||
3. Biological functions are usually extremely specific and require the presence of microbes that are capable of metabolizing the contaminants. In order for the correct microbes to be present, the appropriate environmental conditions, levels of nutrients, and contaminants need to be met. [12] | |||
4. Scaling up the size of studies from small initial studies to commercial-scale field operations is difficult.[12] | |||
5. The real environment contains contaminants that are mixed, unevenly distributed, and in different phases (solid, liquid, gas). More research needs to be completed to create technologies that can adapt. [12] | |||
6. Compared to other treatment technologies, bioremediation often takes more time. [12] | |||
7. Problems with ensuring adequate contact between the microbes and the contaminant. preferential pathway and soil structure can leave uncertainty in remediation dispersal.[12] | |||
=='''References'''== | =='''References'''== | ||
Line 319: | Line 307: | ||
13. "Dechloromonas Aromatica RCB." JGI Genome Portal, 16 Feb. 2016. [http://genome.jgi.doe.gov/decar/decar.home.html http://genome.jgi.doe.gov/decar/decar.home.html] | 13. "Dechloromonas Aromatica RCB." JGI Genome Portal, 16 Feb. 2016. [http://genome.jgi.doe.gov/decar/decar.home.html http://genome.jgi.doe.gov/decar/decar.home.html] | ||
14. King, R. Barry, John K. Sheldon, and GIlbert M. Long. Practical Environmental Bioremediation: The Field Guide. 2nd ed. Boca Raton: CRC, 1998. | 14. King, R. Barry, John K. Sheldon, and GIlbert M. Long. (1998). Practical Environmental Bioremediation: The Field Guide. 2nd ed. Boca Raton: CRC, 1998. | ||
15. "Manual, Bioventing Principles and Practices." United States Environmental Protection Agency I (1995) | 15. "Manual, Bioventing Principles and Practices." United States Environmental Protection Agency I (1995) | ||
Line 337: | Line 325: | ||
22. Bio-filters for Edge-of-Field Water Quality Management. (n.d.). Retrieved February 24, 2016, from [http://agronomyday.cropsci.illinois.edu/2000/bio-filters/index.html http://agronomyday.cropsci.illinois.edu/2000/bio-filters/index.html] | 22. Bio-filters for Edge-of-Field Water Quality Management. (n.d.). Retrieved February 24, 2016, from [http://agronomyday.cropsci.illinois.edu/2000/bio-filters/index.html http://agronomyday.cropsci.illinois.edu/2000/bio-filters/index.html] | ||
23. Alexander, Martin. Biodegradation and Bioremediation. San Diego: Academic | 23. Alexander, Martin. (1999). Biodegradation and Bioremediation. San Diego: Academic Print. | ||
24. Litchfield, Carol. "Thirty Years and Counting: Bioremediation in Its Prime?" BioScience 55.3 (2005): 273. | 24. Litchfield, Carol. "Thirty Years and Counting: Bioremediation in Its Prime?" BioScience 55.3 (2005): 273. | ||
Line 345: | Line 333: | ||
26. Scow, Kate. “Lectures in Soil Microbiology.” UC Davis, Winter 2016. | 26. Scow, Kate. “Lectures in Soil Microbiology.” UC Davis, Winter 2016. | ||
27 CLU-IN | Technologies Remediation About Remediation Technologies Natural Attenuation Overview. (n.d.). Retrieved February 24, 2016, from | 27 CLU-IN | Technologies Remediation About Remediation Technologies Natural Attenuation Overview. (n.d.). Retrieved February 24, 2016, from https://clu-in.org/techfocus/default.focus/sec/Natural_Attenuation/cat/Overview/ | ||
28. Chauhan, Ashok K., and A. Varma. A Textbook of Molecular Biotechnology. New Delhi: I.K. International Pub. House, 2009. Print. | 28. Chauhan, Ashok K., and A. Varma. A Textbook of Molecular Biotechnology. New Delhi: I.K. International Pub. House, 2009. Print. | ||
Line 351: | Line 339: | ||
29. Biopiles. (n.d.). Retrieved March 13, 2016, from [http://www.navfac.navy.mil/navfac_worldwide/specialty_centers/exwc/products_and_services/ev/erb/tech/rem/biopiles.html http://www.navfac.navy.mil/navfac_worldwide/specialty_centers/exwc/products_and_services/ev/erb/tech/rem/biopiles.html] | 29. Biopiles. (n.d.). Retrieved March 13, 2016, from [http://www.navfac.navy.mil/navfac_worldwide/specialty_centers/exwc/products_and_services/ev/erb/tech/rem/biopiles.html http://www.navfac.navy.mil/navfac_worldwide/specialty_centers/exwc/products_and_services/ev/erb/tech/rem/biopiles.html] | ||
30 .Bioremediation of soils contaminated with polycyclic aromatic hydrocarbons, petroleum, pesticides, chlorophenols and heavy metals by composting: Applications, microbes and future research needs. ( | 30. Chen, M., Xu, P., Zeng, G., Yang, C., Huang, D., & Zhang, J. (2015). Bioremediation of soils contaminated with polycyclic aromatic hydrocarbons, petroleum, pesticides, chlorophenols and heavy metals by composting: Applications, microbes and future research needs. Biotechnology Advances, 33(6, Part 1), 745–755. | ||
31. Bioaugmentation as a strategy for cleaning up of soils contaminated with aromatic compounds. ( | 31. Mrozik, A., & Piotrowska-Seget, Z. (2010). Bioaugmentation as a strategy for cleaning up of soils contaminated with aromatic compounds. Microbiological Research, 165(5), 363–375. Retrieved March 13, 2016, from [http://www.sciencedirect.com/science/article/pii/S0944501309000585 http://www.sciencedirect.com/science/article/pii/S0944501309000585] | ||
32. Bioremediation, Biostimulation and Bioaugmention: A Review. (n.d.). Retrieved March 13, 2016, from http://pubs.sciepub.com/ijebb/3/1/5/ | 32. Bioremediation, Biostimulation and Bioaugmention: A Review. (n.d.). Retrieved March 13, 2016, from http://pubs.sciepub.com/ijebb/3/1/5/ | ||
Line 359: | Line 347: | ||
33. Sulfur Oxides—Advances in Research and Application: 2013 Edition | 33. Sulfur Oxides—Advances in Research and Application: 2013 Edition | ||
34. Biosorption: | 34. Fomina, M., & Gadd, G. M. (2014). Biosorption: current perspectives on concept, definition and application. Bioresource Technology, 160, 3–14. Retrieved February 24, 2016, from [https://www.researchgate.net/publication/259955406_Biosorption_Current_perspectives_on_concept_definition_and_application https://www.researchgate.net/publication/259955406_Biosorption_Current_perspectives_on_concept_definition_and_application] | ||
35. Kotrba, Pavel, Martina Mackova, and Tomas Macek. (2011). Microbial Biosorption of Metals. Dordrecht: Springer Science Business Media Print. | |||
36. Bioventing » Water and Soil Bio-Remediation. (n.d.). Retrieved February 24, 2016, from [http://waterandsoilbioremediation.com/index.php/in-situ-remediation-methods/bioventing http://waterandsoilbioremediation.com/index.php/in-situ-remediation-methods/bioventing] | |||
37. Mrozik, A., & Piotrowska-Seget, Z. (2010). Bioaugmentation as a strategy for cleaning up of soils contaminated with aromatic compounds. Microbiological Research, 165(5), 363–375. | |||
38. Land Farming. (n.d.). Retrieved March 13, 2016, from http://www.cpeo.org/techtree/ttdescript/lanfarm.htm | |||
<!-- Do not edit or remove this line --> [[Category:Pages edited by students of Kate Scow at UC Davis]] |
Latest revision as of 03:47, 12 March 2018
Through agriculture, industry, and daily life, harmful chemicals have been released into the earth’s air, soil, and water. Depending on their concentrations, these substances can have destructive consequences on ecosystems, as well as cause severe damage to humans and other organisms nearby. Soil pollution is of special importance because of its impact on surface, groundwater and air contamination and can easily spread and be consumed by humans.
Biodegradation is the biologically catalyzed modification of an organic chemical's structure. However, this modification can be through different metabolic pathways and does not necessarily mean a reduction in toxicity. Mineralization, one type of biodegradation, is defined as the conversion of an organic substance to its inorganic constituents, rendering the original compound harmless. [23]. Transformation is defined as any metabolically-induced change in the chemical composition of a compound [14].
Bioremediation refers to the use of microorganisms to degrade contaminants that pose environmental and human risks. Bioremediation processes typically involve the actions of many different microbes acting in parallel or sequence to complete the degradation process. Both in situ (in place) and ex situ (removal and treatment in another place) remediation approaches are used. The versatility of microbes to degrade a vast array of pollutants makes bioremediation a technology that can be applied in different soil conditions [3]. Though it can be inexpensive and in situ approaches can reduce disruptive engineering practices, bioremediation is still not a common practice [1].
A widely used approach to bioremediation involves stimulating naturally occurring microbial communities, providing them with nutrients and other needs, to break down a contaminant. This is termed biostimulation. Biostimulation can be achieved through changes in pH, moisture, aeration, or additions of electron donors, electron acceptors or nutrients. Another bioremediation approach is termed bioaugmentation, where organisms selected for high degradation abilities are used to inoculate the contaminated site [3]. These two approaches are not mutually exclusive- they can be used simultaneously.
Recent awareness of the dangers of many chemicals used in society has led to research on formulation of products that are more easily degraded in the environment.
From an ecological point of view, bioremediation depends on the various interactions between three factors: substrate (pollutant), organisms, and environment, as shown in the figure at right [4]. The interactions of these factors affect biodegradability, bioavailability, and physiological requirements, which are important in assessing the feasibility of bioremediation [4]. Biodegradability, or whether a chemical can be degraded or not, is determined by the presence or absence of organisms that are able to degrade a chemical of interest and how widespread these organisms are in the site [4]. The substrate (pollutant) can interact with its surrounding environment to change its bioavailability, or availability to organisms that are capable of degrading it; for example, substrate has low bioavailability if it is tightly bound to soil organic matter or trapped inside aggregates [4]. Physiological requirements, or set of conditions required by organisms to carry out bioremediation in the environment, include nutrient availability, optimal pH, and availability of electron acceptors, such as oxygen and nitrate [4]. Also, the environment needs to be habitable for organisms involved in bioremediation [4].
Brief History

Microorganisms in the environment have always broken down waste, and humans have always (knowingly or unknowingly) used them in agricultural, domestic, and industrial activities [24]. As the urbanized world shifted to a more industrial system, however, people began to take an active approach in bioremediation. In the late nineteenth century, wastewater treatment plants were formed, but even so, this was not officially called bioremediation . The project considered the initial spark of the bioremediation movement was the report “Beneficial Stimulation of Bacterial Activity in Groundwater Containing Petroleum Products” by R.L. Raymond et al. in 1975. By testing the relationship between oil presence and bacterial stimulation, Raymond found that adding nutrients to soil hastened the oil removal. This led to the development of in situ bioremediation [24].
Initial bioremediation projects focused on “pump and treat” (ex situ) methods in soil around gas stations and refinery spills to get oil out of groundwater sources, but soon cleaning up chlorinated hydrocarbons became a primary concern [24]. Chlorinated compounds were commonly used in pesticides, but when people learned it was a possible carcinogen and causing ozone depletion, research into bioremediation took off [24]. This was when anaerobic bacteria started being used, as it was discovered that they dechlorinate compounds much more quickly than do aerobic bacteria, and produce fewer damaging iron compounds that precipitate from the reactions [24].
Overview of Pollutants
Pollutants found in soils present a variety of different human health risks. Soil pollutants are typically classified as organic and inorganic pollutants. The remediation of some of these pollutants will be discussed in greater depth in the following sections. Below is a link to website with a list of examples of soil pollutants and their effects on human health:
Summary of health effects of pollutants
Organic Pollutants
Industrialization resulted in increased use of organic compounds that build up and persist in the environment [11]. Main sources of organic pollutants are through anthropogenic activities, including use of solvents, pesticides, and fuels [11]. Some of these organic compounds are highly toxic and they are associated with variety of health issues around the world [11].
Table below lists some groups of contaminants, examples, and their sources.
While organic pollutants are causing both environmental and health problems, bioremediation offers an effective solution to the pollution [11]. The table below lists some of the organic pollutants and microorganisms that are found to be able to degrade them.
Inorganic Pollutants
Pollutant | Source |
---|---|
Arsenic | Pesticides, wood preservatives, biosolids, ore mining and smelting |
Cadmium | Paints and pigments, plastic stabilizers, electroplating, phosphate fertilizers |
Chromium | Tanneries, steel industries, fly ash |
Copper | Pesticides, fertilizers, biosolids, ore mining and smelting |
Mercury | Gold and Silver mining, coal combustion |
Nickel | Effluent, kitchen appliances, surgical instruments, automobile batteries |
Lead | Aerial emission from combustion of leaded fuel, batteries waste, insecticide and herbicides. |
A majority of heavy metal pollutants come from human sources that accumulate over time.
There are also natural forms of contamination from normal biological processes, which include:
1. Weathering of minerals over time
2. Erosion and volcanic activities
3. Forest fires and biogenic source
4. Particles released by vegetation
Heavy metals can be absorbed by microbes at cellular binding sites. Extracellular polymers of these microbes can complex heavy metals through various mechanisms [21]. These specialized microorganisms can mineralize the organic contaminants to metabolic intermediates, which are used as primary substrates for cell growth. The microbes prevalent in heavily metal-contaminated soil can alter the oxidation state of the heavy metals by immobilizing them [21], allowing them to be easily removed. Bioremediation of heavy metals from microbes is not heavily researched, mostly due to an incomplete understanding of the genetics of the microbes used in metal adsorption. Geomicrobiology takes a better look at the interactions between microbes and inorganic material.
Organisms
As stated previously, bioremediation involves various microorganisms that are able to degrade and reduce toxicity of environmental pollutants [12]. Therefore, the interactions of microbes with the environment and pollutants are significant in determining effectiveness of bioremediation [4]. Those microbes can be either naturally present in the site of bioremediation or isolated from other sites and inoculated artificially [12]. Biodegradation often occurs as part of microbial metabolism and in some cases, microbes are able to directly harvest carbon and energy by breaking down pollutants [12]. Sections below go over bacteria and fungi, the commonly used organisms in bioremediation, and archaea, the more recently discovered group of organisms with unique potential in bioremediation.
Bacteria
Bacteria are widely diverse organisms, and thus make excellent players in biodegradation and bioremediation. There are few universal toxins to bacteria, so there is likely an organism able to break down any given substrate, when provided with the right conditions (anaerobic versus aerobic environment, sufficient electron donors or acceptors, etc.). Below are several specific bacteria species known to participate in bioremediation.
Pseudomonas putida
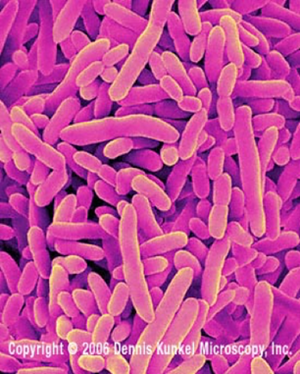
Pseudomonas putida is a gram-negative soil bacterium that is involved in the bioremediation of toluene, a component of paint thinner. It is also capable of degrading naphthalene, a product of petroleum refining, in contaminated soils. [2]
Dechloromonas aromatica
Dechloromonas aromatica is a rod-shaped bacterium which can oxidize aromatics including benzoate, chlorobenzoate, and toluene, coupling the reaction with the reduction of oxygen, chlorate, or nitrate. It is the only organism able to oxidize benzene anaerobically. Due to the high propensity of benzene contamination, especially in ground and surface water, D. aromatic is especially useful for in situ bioremediation of this substance. [13]
Nitrifiers and Denitrifiers
Industrial bioremediation is used to clean wastewater. Most treatment systems rely on microbial activity to remove unwanted mineral nitrogen compounds (i.e. ammonia, nitrite, nitrate). The removal of nitrogen is a two stage process that involves nitrification and denitrification. During nitrification, ammonium is oxidized to nitrite by organisms like Nitrosomonas europaea.Then, nitrite is further oxidized to nitrate by microbes like Nitrobacter hamburgensis.
In anaerobic conditions, nitrate produced during ammonium oxidation is used as a terminal electron acceptor by microbes like Paracoccus denitrificans [2]. The result is N2 gas. Through this process, ammonium and nitrate, two pollutants responsible for eutrophication in natural waters, are remediated.
Deinococcus radiodurans
Deinococcus radiodurans is a radiation-resistant extremophile bacterium that is genetically engineered for the bioremediation of solvents and heavy metals. An engineered strain of Deinococcus radiodurans has been shown to degrade ionic mercury and toluene in radioactive mixed waste environments [7].
In anaerobic conditions, nitrate produced during ammonium oxidation is used as a terminal electron acceptor by microbes like Paracoccus denitrificans [2]. The result is dinitrogen gas. Through this process, ammonium and nitrate, two pollutants responsible for eutrophication in natural waters, are remediated.

Methylibium petroleiphilum
Methylibium petroleiphilum (formally known as PM1 strain) is a bacterium capable of methyl tert-butyl ether (MTBE) bioremediation. PM1 degrades MTBE by using the contaminant as the sole carbon and energy source [8].
Alcanivorax borkumensis
Alcanivorax borkumensis is a marine rod-shaped bacterium which consumes hydrocarbons, such as the ones found in fuel, and produces carbon dioxide. It grows rapidly in environments damaged by oil, and has been used to aid in cleaning the more than 830,000 gallons of oil from the Deepwater Horizon oil spill in the Gulf of Mexico [25].
Fungi (Mycoremediation)
Current bioremediation applications primarily utilize bacteria, with comparatively few attempts to use fungi. Fungi have fundamentally important roles because of their participation in the cycling of elements through decomposition and transformation of organic and inorganic materials. These characteristics can be translated into applications for bioremediation which could break down organic compounds and reduce the risks of metals. In some cases, fungi have an advantage over bacteria not just in metabolic versatility but also their environmental resilience.They are able to oxidize a diverse amount of chemicals and survive in harsh environmental conditions such as low moisture and high concentrations of pollutants. Therefore, fungi are potentially an extremely powerful tool in soil bioremediation and some versatile species such as White Rot Fungi have been a hot topic of research. [16,17]
Biodegradation Capacities of White rot fungi
Using fungi as potential treatment of contaminants began in 1985 when the white rot species Phanerochaete chrysosporium was discovered to metabolize multiple key environmental pollutants. The most important feature of these fungi is their enzymatic functional ability to metabolize complex chemicals such as lignin. Similar abilities were later discovered in other white rot fungal species. In addition, white rot fungi are highly advantageous because they degrade lignin extracellularly through its hyphal extension. This allows them to access soil contaminants that other organisms are incapable of and maximize surface area for enzymatic interaction. These inexpensive fungi can tolerate extreme environmental conditions, such as pH, temperature, and moisture content. While many microbial organisms that are used for bioremediation require pre-conditioning of the environment for them to survive in, white rot fungi can directly be applied into most systems because they degrade based upon nutrient deprivation. [18]
Phanerochaete chrysosporium
P. chrysosporium was the first fungi linked to degradation of organic pollutants. Extensive research has show this it has strong potential for bioremediation in pesticides, PAHs, dioxins, carbon tetrachloride, and many other pollutants. Among fungal systems, P. chrysosporium has become the model for bioremediation. Other notable species of white rot fungi include Pleurotus ostreatus and Trametes versicolor. [18]
Bioremediation of Hydrocarbon Pollutants
Hydrocarbons are stored deep underground but are brought up to the surface to be transformed and utilized, primarily as an energy source known as fossil fuels. The majority of pollution currently comes from these byproducts in the form Polycyclic Aromatic Hydrocarbons (PAHs), which are xenobiotic environmental pollutants that form when carbon materials are incompletely combusted. Some of examples of PAHs include burning wood, fossil fuels, and cigarette smoke. [19,20] Currently, bioremediation is only effective for soils contaminated with low-molecular weight PAHs because of bacterial commercial use. However, fungi are effective at PAH degradation in comparison to bacteria for a few reasons. Firstly, they are capable degrading PAH’s that are high in molecular weight, bacteria in comparison are better at degrading smaller molecules. Secondly, fungi can function well in non-aqueous environments and low oxygen conditions, both are conditions where PAH’s can accumulate. Many fungi have evolved mechanisms that allow the to target specific PAHs. Fungi produce extracellular enzymes that degrade lignin, a process called mineralization the produces carbon dioxide as the end product. [19,20]
Remediating Metals
Toxic metals can enter the environment all life cycle stages of metal compound. For example, metal leaching can occur from the mining process till the disposal of metal wastes. However in nature, the mobility of metals comes from the geological processes that can be released into the soil and aquatic environments. The environmental largest risk from metal contamination comes from the relationship between metals and compounds that are inherently of incapable of being degraded by any natural procedures. The best solution to treating contamination is transporting the metals to location where they cannot produce negative environmental effects. Fungi have various ways of interacting with metals, some of the techniques are increasing or decreasing the mobility of metals, sorption, or even cellular uptake. After the metals have been absorbed the fungus, they can chemically altered to be stored or translocated through the hyphae and into various plants that participate in symbiosis. [17]
Pesticide Degradation
Pesticide accumulation is an issue of great concern among the public, because they are directly associated with food products and water supplies. There are number of technologies used for pesticide clean-up; however, these technologies are generally expensive and inefficient because they require contaminated soil to be excavated and sent to a separate storage location for processing. Bioremediation offers a potential solution that treats contaminated soil and groundwater without needing excavation. Studies show that White Rot Fungi has high promise for soil bioremediation application; however, most tests have been conducted in the lab rather than in the actual environment. This fungi demonstrates the ability to transform and mineralize specific pesticides in soil. [18]
Environmental Applications
Although fungi demonstrate significant biochemical and ecological useful qualities, they are hardly utilized for biotechnological purposes. Instead, bacteria are most commonly used because they usually produce superior results in their numerous advantages ranging from their highly specific biochemical reactions to their capabilities of breaking down pollutants efficiently [17]. Fungi are underused primarily because of the costs that come from providing oxygen to fungi in polluted environments. However, filamentous fungi could be highly valuable in situations where bacteria cannot perform. For example, fungi are useful in situations where contaminants are physically blockaded and bacteria cannot reach or in circumstances of environmental extremes such as high acidity or dryness prevent bacteria from functioning. [17]
Archaea
The role of archaea in bioremediation has not been studied as commonly as that of bacteria [10]. Nevertheless, numbers of researchers have shown their ability to degrade various pollutants and scientists began to discover more about their potential in participating in bioremediation. Below lists some important facts regarding archaea’s potential role in bioremediation.
- Biodegradation by extreme halophilic archaea was not recognized widely in the past, but scientists have found out that extreme halophilic archaea have greater catabolic diversity than expected [9]
- Hydrocarbon-contamination is observed in some extreme environments, including hypersaline (high salt concentration), high or low temperature, or extreme pH [10]. Archaea’s adaptation to extreme environment gives them the potential to participate in biodegradation and bioremediation in these environments; in fact, microorganisms naturally adapted to the cold environments are known to be important degraders of hydrocarbons in those environments [10].
- Extreme halophilic archaea has potential to biodegrade pollutants in hypersaline environment, in which bacteria typically used in bioremediation cannot survive or function properly. [5]
- Some archaea are known to be resistant to variety of antibiotics, including penicillin, cycloheximide, streptomycin, etc, which gives them great advantage in participating in bioremediation in the presence of antibiotics [5].
Examples of studies of Archaea involved in bioremediation
Four extreme halophilic strains of archaea (belonging to genus Halobacterium, Haloferax, and Halococcus) were studied to evaluate their potential to biodegrade crude oil and hydrocarbons. [5] All four strains could use various kinds of hydrocarbons as their carbon or energy sources [5]. Two strains of Haloferax grew on n-alkanes with different lengths, ranging from C8 to C34, and also benzene, toluene, biphenyl, and naphthalene. The research demonstrated the important fact that archaea have potential to carry out biodegradation at high temperatures, in the range of 40-45 °C [5], which is advantageous because hydrocarbons have higher solubility and bioavailability at these higher temperature [10]. The four strains studied were resistant to six different antibiotics, including penicillin, streptomycin, cycloheximide [5] and this gave them the potential to carry out biodegradation in conditions unfavorable for bacteria. Research suggests other genera of archaea are also capable of biodegrading in hypersaline environments [6]
Archaeglobus fulgidus, a hyperthermophile which can use sulfate as an electron acceptor, can also break down various aromatic hydrocarbons (Peeples, 2014).
Microbial Processes
Microorganisms use a wide range of processes to transform chemicals in their environment. In some cases, pollutants serve as the carbon and energy source for microbial growth, while in other cases, pollutants serve as the terminal electron acceptor. This manifests itself in the diverse ability of microbes to transform and degrade toxic molecules. Below, several steps and details of the microorganisms’ actions are described.
Factors Affecting Rates of Biodegradation
Biodegradation may be influenced by pH, temperature, moisture, carbon sources, soil texture, aerobic versus anaerobic conditions, the number of substituents, and the concentration of the pollutant. It is impossible, however, to make a generalization about the best universal conditions for biodegradation. What’s toxic to some microbes is a nutrient to others, what might be a damaging pH to some is beneficial to others, and so on.
A greater amount of substituents will cause slower degradation in aerobic environments, but faster degradation in anaerobic ones. Chlorine makes a molecule less degradable due to steric hindrance preventing access to necessary enzymes, therefore molecules with higher chlorination are slower to degrade in aerobic conditions. High concentration of a pollutant generally results in faster rates of degradation. If the concentration drops below a threshold concentration, the enzymes may not detect it and will cease to degrade it [26].
Soil with small pores, especially clays, may cause biodegradation to take years due to the decrease in bioavailability. Chlorine makes a molecule less degradable due to steric hindrance preventing necessary enzymes from accessing the compound, therefore molecules with higher chlorination are slower to degrade.
The rate at which a compound is transformed, as well as the curves that describe its transformation, is referred to as kinetics, and is affected by all factors listed above. First order kinetics (exponential decay) often describes biodegradation when the initial substrate concentration is low, while zero-order kinetics (linear biodegradation) is often observed when the substrate concentration is very high. In some cases if the concentration of the chemical falls below a critical threshold concentration, the microbes can no longer transform it and the chemical persists.
The power rate model depicting the relationship between concentration and rate of degradation (first order decay here) is as follows:
-dC/dt = kC^n
C is substrate concentration, t is time, k is a rate constant for the chemical in question, and n is an appropriate parameter. The values of k and n are adjusted until a line is found to match experimental data [23].
Primary substrate utilization
Primary substrate utilization occurs when a microbe both transforms a substrate and uses it as an energy or carbon source. [15] An electron acceptor is required for these transformations. It can be anaerobic or aerobic, although the presence of oxygen tends to speed up reactions. This type of biodegradation is common in break down of petroleum compounds and some pesticides.
Cometabolism (Secondary Substrate Utilization)
Cometabolism involves the fortuitous transformation of a chemical by an organism while the organism uses a different substance as its primary energy or carbon source [14]. During the actual reaction degrading the substance, it appears that the organism involved has no net carbon or energy gain, and may even result in a product which is toxic to the cell [14].
A key example of cometabolism is fortuitous metabolism in the degradation of trichloroethylene, shown in the diagram below. An organic growth substrate such as propane or butane is required for the enzymatic activity that transforms TCE. [14]
Reductive and Hydrolytic Dehalogenation
Chloride and other halogens are common components of pesticides and hazardous industrial wastes, and by removing them the toxic chemical can often be remediated [23]. If the halogen is replaced by a hydrogen (RCl -> RH), then it is reductive dehalogenation. If two halogens are replaced simultaneously, then the process is called dihaloelimination, although it still falls under reductive dehalogenation [14]. If the halogen is replaced by OH (RCl -> ROH) then it’s hydrolytic dehalogenation. In both cases, the halogen is released as its inorganic form into the environment [23].
Acclimation
An acclimation period, also called an adaptation or lag period, occurs when no destruction of a given chemical is observed [23]. It is caused by the microbes transitioning to their altered environment and shifting their metabolism to better suit it [14]. It can last for anywhere from hours (such as aromatic compounds in warm, oxygenated soils) to months (such as halobenzoates in anaerobic sediments) depending on the chemical in question and the environment [23]. Acclimation periods can be affected by temperature, the presence of oxygen, pH, and concentration of the substance. Although they are most often faster in warm, aerated, and fairly dry environments, there are few consistencies between what shortens or lengthens the period, even if the concentration is the same [23]. Insecticides including methyl parathion and azinphosmethyl; herbicides including 2, 4-D, MCPA, Mecoprop, TCA, and amitrole; the quaternary ammonium compound dodecyltrimethylammonium chloride; polycyclic aromatic hydrocarbons including naphthalene and anthracene; and other chemicals such as phenol, chlorobenzene, PCP, diphenyl-methane, and NTA have all been reported to have acclimation periods, and this can be of severe human concern [23]. The continued presence of these toxins extends human, plant, and animal exposure, and if the chemical is in water, it can allow the substance to flow further and impact environments distant to its site of origin before being degraded.
Detoxification and Activation
Detoxication, sometimes called detoxification, has been referred to as the “most important role of microorganisms in the transformation of pollutants” [23]. The process is the changing of a molecule into something less harmful to a species in question. There are a number of ways a molecule can be transformed, including hydrolysis, hydroxylation, dehalogenation, demethylation, methylation, and ether cleavage [23]. By breaking bonds, or adding or removing groups, the organism reduces its effect on the environment. Furthermore, although sometimes the resulting chemical is simply excreted as waste, the organism may also be able to use this new compound as a carbon source or further modifies it until it is released as CO2 [23].
There are instances where the initial compound is harmless, and in fact the substance produced by microorganisms, or an intermediate in the degradation process, is a toxin [23]. This process is called activation. For this reason, it is important to test all steps of a reaction when determining how a compound is degrading. The new toxins may also be more or less mobile than its predecessor, so it can either stick around one area for extended periods of time or spread to other areas and increase damage [23]. A prevalent example of this is the dechlorination of TCE, which produces DCE (50 times more hazardous than TCE) and Vinyl Chloride (a known carcinogen) [14]. Commonly used insecticides in the past, like zinophos, trichloronat, and carbofuran, were all found to increase a soil’s toxicity with extended use [23].
Bioremediation treatment methods
In order for bioremediation to be successful, it requires sufficient proof for the degradation of contaminants. However, determining the effectiveness and completeness to reach sufficient results is one of the major issues. Natural attenuation relies on natural processes to clean up or attenuate pollution in soil and groundwater [27]. This remediation is done without human interaction, and is primarily used as a monitoring technique, to make sure more aggressive cleanup strategies are not needed. Abiotic and biotic factors play a distinguishing factor of how effective bioremediation is.
Current monitoring practices determine the disappearance of contaminants and their degradation products to regulatory levels that are monitored by toxicity testing, usually on single organisms or species to ensure there are no induced changes that may result in residual toxicity. The problem with these monitoring techniques is that the assessment of contaminants may result in an inaccurate indicator of residual toxicity[28]. Rather, studying the microbial community response may be a more comprehensive indicator of residual toxicity than a single species. Once sufficient evidence is provided, human intervention may be needed for a more effective cleanup process.
There are two types of remediation that are done, ex situ: which is done by removing the contaminated soil or water and treating it outside the source, and in situ: which treatment takes place within the contaminated area. There are some treatments methods that can be either ex situ or in situ. Some techniques may deal with the mobilization of pollutants, to move them out of an area, or immobilized to keep them out of an area such as a water table.
Ex-situ
Ex-situ techniques are those that are applied to soil and groundwater which has been removed from the site via excavation or pumping [12]. The methods used include composting, biofilters, and biopiling. Ex-situ is used for smaller projects, primarily because larger excavation of soil is not prefered. The movement of the soil can be more detrimental by destroying the preestablish horizons in the soil.
Biopiling
Excavated soils are mixed with soil amendments and placed on a treatment area. Biopiles are aerated with the use of perforated pipes and blowers in order to control the progression of biodegradation more efficiently by controlling the supply of oxygen [29], which in turn may affect other factors such as pH. This system is primarily used to remediate systems with oil and hydrocarbon contamination. The remediated soil is placed in a liner to prevent further contamination of the soil, they may also be covered with plastic to control runoff, evaporation, and volatilization.
Composting
Nutrients are added to soil that is mixed to increase aeration and activation of indigenous microorganisms. Composting is done in a separate container, then when composting is complete it is incorporated into the soil. Bioremediation by the utilization of compost relies on the adsorption capabilities of organic matter and the degradation capabilities of microorganisms present[30]. Composting is recognized as as one of the most cost-effective technologies for soil bioremediation and it can be done on large and small scales. The use of composting is a very versatile technique for soil polluted by a wide range of organic pollutants and heavy metals, making it great for easier remediation involving various pollutants. The utilization of organic wastes for soil remediation is also helpful in decreasing the need for their storage and treatment. Organic matter that is generated from composting offers the benefit of improving soil quality and structure. Composting is primarily used for remediation over a longer period of time, as the nutrients for the microbes are released gradually and requrire more time compared to quicker treatments such as biostimulation.
In-situ
In-situ techniques are applied to soil and groundwater at the site with minimal disturbance[12]. These methods include biostimulation, bioleaching, biosorption, and bioventing. In-situ is preferred because it is often minimally invasive to the soil structure in comparison to ex-situ, but it can be expensive due to specialized equipment.
Biostimulation
This method involves the addition of nutrients to a polluted site in order to encourage the growth of naturally occurring chemical-degrading microorganisms[31]. Biostimulation is primarily done by the addition of various nutrients that are limited in the soil as well as electron acceptors, such as phosphorus, nitrogen and oxygen, or increasing the amount of available carbon in order to increase the population or activity of naturally occurring microorganisms. Other approaches are to optimize environmental conditions such as aeration, the addition of nutrients, altering pH and temperature control [32]. The primary advantage of biostimulation is that it is done by native microorganisms that are well-suited to the environment, and are already well distributed spatially. The challenge is delivering additives so they are readily available to the subsurface microbes.
Metal Biosorption
Adsorption of metals and other ions of an aqueous solution by the use of microbes. The biosorption process involves a solid phase and a liquid phase containing a dissolved species to be sorbed [34]. The process continues until equilibrium is established between the amount of solid-bound sorbate species and its portion remaining in the solution. The degree of affinity for the sorbate determines its distribution between the solid and liquid phases.
Biosorption processes are very important in the environment, and has been utalized for conventional biotreatment processes. Biosorption is primarily aimed at the removal or recovery of organic and inorganic substances from solution [35]. The commercialization of biosorption technologies has been limited so far.
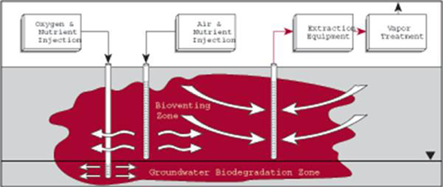
Bioventing
Bioventing is an In situ remediation technology that uses indigenous microorganisms to biodegrade organic constituents adsorbed to soils in the unsaturated zone[36]. The availability of oxygen generally controls the rate at which aerobic bioremediation proceeds. Bioventing is the coupling of soil venting and bioremediation. Bioventing can be successfully applied to compounds ranging from gasoline or diesel, to heavier hydrocarbons[36]. The addition of nutrients with the bioventing flow rates can achieve greater contaminant reductions than venting alone.
Ex-situ or In-situ
Some methods can be used by either in-situ or ex-situ methods. The soil or water can be removed from the contamination source and treated, or treated at the source, the method chosen can be based on many factors such as how expensive the project may be or how much contaminant needs to be treated. These methods include bioaugmentation, land farming and biofiltration.
Bioaugmentation
Bioaugmentation is the addition of non-native microorganisms that have the ability to degrade the contaminants that are recalcitrant to the indigenous microbiota. Bioaugmentation has been proven successful in cleaning organic pollutant, but still faces many environmental problems, such as the survival of strains introduced to soil[37]. The number of introduced microorganisms usually decreases shortly after soil inoculation.
Bioaugmentation is ideal for soil:
1. With low number of microbes that are capable of degrading targeted pollutants
2. Containing compounds requiring multi stepped remediation.
Augmentation techniques have a great potential for aromatic compound remediation. The most important step in successful bioaugmentation is selection of proper microbial strains. The success of bioaugmentation strongly depends on the ability of inoculants to survive in contaminated soil, which may vary due to predation and an environment that does provide all the conditions and nutrients that the organism needs to survive. In some cases the environment may be toxic to the added organism.
Land Farming
Contaminated soil is mixed with amendments such as nutrients, and then they are tilled into the earth, or the contaminated soil is applied into lined beds and periodically turned over or tilled to aerate the waste [38]. The topmost layer is the area of concentration for this method, so it is not ideal for deeper remediation. Land farming differs from composting because it actually incorporates contaminated soil into soil that is uncontaminated [38]. The higher zone of remediation will typically contain primarily lighter hydrocarbons that can be volatilized. The material is periodically tilled for aeration to hasten remediation of any nutrients and allow more oxygen to act as electron acceptors, as well as allowing volatilization to occur. Contaminants are degraded, transformed, and immobilized by microbiological processes and oxidation. Soil conditions are controlled to optimize the rate of contaminant degradation, moisture content, frequency of aeration, and pH are all conditions that may be controlled [38].
Biofilter
Biofilters are primarily used for the filtration of contaminated groundwater in the soil. Biofilters can be used above soil, where the water will be pumped aboveground for treatment, or a filter can be placed in the soil near an outflow. A micro-algal/bacterial biofilter can be used for the detoxification of copper and cadmium metal wastes [22]. Biofilters have been used in larger industry environments to treat contaminated outflow of water. Chromobacterium violaceum, is used to treat water and soil contaminated with silver nanoparticles, reducing its concentration.
Bioremediation Synopsis
Advantages
1. Bioremediation that involves natural attenuation or biostimulation is a publicly accepted treatment of polluted soil because it is based upon natural processes. Microbes that metabolize contaminants often increase in population when the contaminant is present and thus rates of biodegradation may increase over time, up to a point. If biodegradation is complete (i.e. mineralization) the products from treatment are harmless; such as carbon dioxide, water, and cellular biomass. [12]
2. In situ bioremediation can result in complete degradation of pollutants into harmless products on site. This removes the risks involved with transportation for treatment and elimination of contaminated substances. [12]
3. Bioremediation can be a cheaper alternative to other technologies used for pollution mitigation. [12]
Disadvantages
1. Only biodegradable compounds are capable of undergoing bioremediation. Not every compound is capable of fully degrading quickly. [12]
2. The products of biodegradation may potentially be even more persistent or toxic than the original contaminant. [12]
3. Biological functions are usually extremely specific and require the presence of microbes that are capable of metabolizing the contaminants. In order for the correct microbes to be present, the appropriate environmental conditions, levels of nutrients, and contaminants need to be met. [12]
4. Scaling up the size of studies from small initial studies to commercial-scale field operations is difficult.[12]
5. The real environment contains contaminants that are mixed, unevenly distributed, and in different phases (solid, liquid, gas). More research needs to be completed to create technologies that can adapt. [12]
6. Compared to other treatment technologies, bioremediation often takes more time. [12]
7. Problems with ensuring adequate contact between the microbes and the contaminant. preferential pathway and soil structure can leave uncertainty in remediation dispersal.[12]
References
1. United States Environmental Protection Agency, "A Citizen's Guide to Bioremediation" 2001.
2. Nitrification and Denitrification Wastewater Treatment. No. 5536407. 16 July 1996.
3. Sylvia, D. M., Fuhrmann, J.F., Hartel, P.G., and D.A Zuberer (2005). "Principles and Applications of Soil Microbiology." New Jersey, Pearson Education Inc.
4. Tiedje, J. M. (1993). Bioremediation from an ecological perspective. In situ bioremediation: When does it work, 110-120.
5. Al-Mailem, D. M., Sorkhoh, N. A., Al-Awadhi, H., Eliyas, M., & Radwan, S. S. (2010). Biodegradation of crude oil and pure hydrocarbons by extreme halophilic archaea from hypersaline coasts of the Arabian Gulf. Extremophiles, 14(3), 321-328. doi: 10.1007/s00792-010-0312-9
6. Fairley, D. J., Boyd, D. R., Sharma, N. D., Allen, C. C., Morgan, P., & Larkin, M. J. (2002). Aerobic metabolism of 4-hydroxybenzoic acid in Archaea via an unusual pathway involving an intramolecular migration (NIH shift). Appl Environ Microbiol, 68(12), 6246-6255.
7. Hassam, Sara C. McFarlan, James K. Fredrickson, Kenneth W. Minton, Min Zhai, Lawrence P. Wackett, and Michael J. Daly. "Engineering Deinococcus radiodurans for metal remediation in radioactive mixed waste environments ." biotech.nature.com 18 (2000): 85-90. 2 Mar. 2008
8. Jessica R., Corinne E. Ackerman, and Kate M. Scow. "Biodegradation of Methyl Tert-Butyl Ether by a Bacterial Pure Culture." Appl Environ Microbiol. 11 (1999): 4788-4792. 2 Mar. 2008
9. Le Borgne, S., Paniagua, D., & Vazquez-Duhalt, R. (2008). Biodegradation of organic pollutants by halophilic bacteria and archaea. J Mol Microbiol Biotechnol, 15(2-3), 74-92. doi: 10.1159/000121323
10. Margesin, R., & Schinner, F. (2001). Biodegradation and biore mediation of hydrocarbons in extreme environments. Appl Microbiol Biotechnol, 56(5-6), 650-663.
11. Kang, J. W. (2014). Removing environmental organic pollutants with bioremediation and phytoremediation. Biotechnol Lett, 36(6), 1129-1139. doi: 10.1007/s10529-014-1466-9
12. Vidali, M. (2001). Bioremediation. an overview. Pure and Applied Chemistry, 73(7), 1163-1172.
13. "Dechloromonas Aromatica RCB." JGI Genome Portal, 16 Feb. 2016. http://genome.jgi.doe.gov/decar/decar.home.html
14. King, R. Barry, John K. Sheldon, and GIlbert M. Long. (1998). Practical Environmental Bioremediation: The Field Guide. 2nd ed. Boca Raton: CRC, 1998.
15. "Manual, Bioventing Principles and Practices." United States Environmental Protection Agency I (1995)
16. Gadd, G. M. (Ed.). (2001). Fungi in bioremediation (No. 23). Cambridge University Press
17. Harms, H., Schlosser, D., & Wick, L. Y. (2011). Untapped potential: exploiting fungi in bioremediation of hazardous chemicals. Nature Reviews Microbiology, 9(3), 177-192
18. Fragoeiro, S. (2005). Use of fungi in bioremediation of pesticides. Applied Mycology Group Institute of Bioscience and Technology. Cranfield University
19. Singh, H. (2006). Mycoremediation: fungal bioremediation. John Wiley & Sons. 283-285
20. Norton, J. M. (2012). Fungi for Bioremediation of Hydrocarbon Pollutants. University of Hawai’i at Hilo. Hohonu, 10, 18-21
21. Dixit, Ruchita, Emptyyn Wasiullah, Deepti Malaviya, Kuppusamy Pandiyan, Udai Singh, Asha Sahu, Renu Shukla, Bhanu Singh, Jai Rai, Pawan Sharma, Harshad Lade, and Diby Paul. "Bioremediation of Heavy Metals from Soil and Aquatic Environment: An Overview of Principles and Criteria of Fundamental Processes." Sustainability 7.2 (2015): 2189-212. Print.
22. Bio-filters for Edge-of-Field Water Quality Management. (n.d.). Retrieved February 24, 2016, from http://agronomyday.cropsci.illinois.edu/2000/bio-filters/index.html
23. Alexander, Martin. (1999). Biodegradation and Bioremediation. San Diego: Academic Print.
24. Litchfield, Carol. "Thirty Years and Counting: Bioremediation in Its Prime?" BioScience 55.3 (2005): 273.
25. Biello, David. "Slick Solution: How Microbes Will Clean Up the Deepwater Horizon Oil Spill." Scientific American (n.d.): n. pag. 25 May 2010.
26. Scow, Kate. “Lectures in Soil Microbiology.” UC Davis, Winter 2016.
27 CLU-IN | Technologies Remediation About Remediation Technologies Natural Attenuation Overview. (n.d.). Retrieved February 24, 2016, from https://clu-in.org/techfocus/default.focus/sec/Natural_Attenuation/cat/Overview/
28. Chauhan, Ashok K., and A. Varma. A Textbook of Molecular Biotechnology. New Delhi: I.K. International Pub. House, 2009. Print.
29. Biopiles. (n.d.). Retrieved March 13, 2016, from http://www.navfac.navy.mil/navfac_worldwide/specialty_centers/exwc/products_and_services/ev/erb/tech/rem/biopiles.html
30. Chen, M., Xu, P., Zeng, G., Yang, C., Huang, D., & Zhang, J. (2015). Bioremediation of soils contaminated with polycyclic aromatic hydrocarbons, petroleum, pesticides, chlorophenols and heavy metals by composting: Applications, microbes and future research needs. Biotechnology Advances, 33(6, Part 1), 745–755.
31. Mrozik, A., & Piotrowska-Seget, Z. (2010). Bioaugmentation as a strategy for cleaning up of soils contaminated with aromatic compounds. Microbiological Research, 165(5), 363–375. Retrieved March 13, 2016, from http://www.sciencedirect.com/science/article/pii/S0944501309000585
32. Bioremediation, Biostimulation and Bioaugmention: A Review. (n.d.). Retrieved March 13, 2016, from http://pubs.sciepub.com/ijebb/3/1/5/
33. Sulfur Oxides—Advances in Research and Application: 2013 Edition
34. Fomina, M., & Gadd, G. M. (2014). Biosorption: current perspectives on concept, definition and application. Bioresource Technology, 160, 3–14. Retrieved February 24, 2016, from https://www.researchgate.net/publication/259955406_Biosorption_Current_perspectives_on_concept_definition_and_application
35. Kotrba, Pavel, Martina Mackova, and Tomas Macek. (2011). Microbial Biosorption of Metals. Dordrecht: Springer Science Business Media Print.
36. Bioventing » Water and Soil Bio-Remediation. (n.d.). Retrieved February 24, 2016, from http://waterandsoilbioremediation.com/index.php/in-situ-remediation-methods/bioventing
37. Mrozik, A., & Piotrowska-Seget, Z. (2010). Bioaugmentation as a strategy for cleaning up of soils contaminated with aromatic compounds. Microbiological Research, 165(5), 363–375.
38. Land Farming. (n.d.). Retrieved March 13, 2016, from http://www.cpeo.org/techtree/ttdescript/lanfarm.htm