African Trypanosomiasis: a parasitic disease of the CNS: Difference between revisions
Mclaughlin2 (talk | contribs) |
Mclaughlin2 (talk | contribs) No edit summary |
||
Line 94: | Line 94: | ||
==Current Research & Next Steps== | ==Current Research & Next Steps== | ||
[[Image:HAT mice.jpg|thumb|300px|left|Results of mice treated with both sucrose and elfornithine post <i>T.brucei</i> infection. Tissue radioactivity levels of various brain regions were measured to reflect both blood-barrier strength and permeability. https://www.ncbi.nlm.nih.gov/pmc/articles/PMC2695853/figure/fig04/ Photo credit:[https://www.ncbi.nlm.nih.gov/pmc/articles/PMC2695853/]]] | |||
Research for more effective treatments and prevention of African trypanosomiasis is still prevalent, despite the fact that there has been a recent decline in cases diagnosed each year. Less toxic treatment options for second stage HAT have been in recent development, one of them being centered around the drug fexinidazole [[#References|[8]]]. Current studies have shown oral administration of fexinidazole has succession rates ~90%, similar to the succession rates of NECT [[#References|[8]]]. Oral administration is becoming the preferred method of HAT treatment due to its overall simplicity and effectiveness. | Research for more effective treatments and prevention of African trypanosomiasis is still prevalent, despite the fact that there has been a recent decline in cases diagnosed each year. Less toxic treatment options for second stage HAT have been in recent development, one of them being centered around the drug fexinidazole [[#References|[8]]]. Current studies have shown oral administration of fexinidazole has succession rates ~90%, similar to the succession rates of NECT [[#References|[8]]]. Oral administration is becoming the preferred method of HAT treatment due to its overall simplicity and effectiveness. |
Revision as of 16:10, 14 April 2024
Introduction
By Maeve McLaughlin

Human African Trypanosomiasis (HAT) informally known as African Sleeping Sickness is an infection directly linked to a microscopic parasitic species known as Trypanosoma brucei. T. brucei are members of the Trypanosoma genus as well as the Trypanosomatidae family of unicellular parasites [3]. HAT is an extremely rare disease in the United States with fewer than 1,000 cases per year, but has a history of epidemics in Sub-Saharan regions of Africa [1]. There are a number of species of trypanosomes but only 2 have been known to infect humans, those being T.b gambiense and T.b rhodesiense [1].
Its transmission occurs through the tsetse fly, a member of the Glossina genus, only found in regions of Sub-Saharan Africa [1]. A bite from the infected species enters the human bloodstream, allowing the parasite to colonize areas up through the lymph nodes. Once infected, the spread of the disease easily develops into the central nervous system working its way to the brain. There are two main stages early and late, the late stage results in neurological defects. Early symptoms commonly consist of headache, fever, rash, or drowsiness [1].
Infected hosts may experience differences in circadian rhythm, insomnia, drowsiness throughout the day, and eventually potential death if left untreated [1]. Those in late stages of the disease experience disturbances in their circadian rhythm, insomnia, or other psychological symptoms such as dementia, depression, mania, irritability, or memory loss [1]. If left untreated, almost all patients are left in a coma, often resulting in death.
Trypanosoma Brucei
Trypanosoma brucei are an extracellular protozoan flagellate parasitic species belonging to the Trypanosoma genus, residing mainly in Sub-Saharan regions of Africa. T. brucei are frequently located extracellularly in blood and tissue regions of the mammalian host, transmitting via leech or arthropod vectors [3]. There are 3 subspecies of T. brucei, yet only 2 species, T. b. gambiense (Tbg), T. b. rhodesiense (Tbr) cause infection in humans [2]. In general, T.brucei has a larger genome than most bacteria, and is a motile bacteria.
The genome of T.brucei is 26 Mb, contains roughly 1700 variant surface glycoproteins along with around 900 pseudogenes [11]. Genome analysis has shown horizontal gene transfer allows for increased metabolic properties for trypanosome parasites [11]. T.b. gambiense is the strain mainly responsible for human infection, accounting for 98% of all diagnosed cases of african trypanosomiasis [3]. This strain is also responsible for retaining the majority of the genetic information with a smaller genome.
Virulence Factors
There are a number of cellular mechanisms, structures, and molecules that allow for full parasitic function of trypanosomes. Regarding immune response, T. brucei parasites are capable of invading a host's immune system through the use of a variable surface glycoprotein (VSG) [7]. VSGs aid in protecting these parasites from lytic factors, ultimately evading the natural host immune response. As infection is prevalent, gene conversion between VSGs occurs at the trypanosome expression site [8]. This is considered an antigenic variation which enables the parasite to avoid the body's immune response as well as continue infection throughout the host. This constant switching also has made it challenging for clinicians to develop a vaccine that trypanosomes cannot evade.
Another strategy to evade immune response is through production of serum resistance associated proteins (SRAs) [7]. SRAs are unique to T.b rhodesiense and considered members of VSGs. They are exported to the flagellar pocket of trypanosomes before getting taken up by extracellular vesicles [12]. Serum resistant forms of T.b rhodesiense express and mRNA encoding protein, associated with surface glycoproteins [12]. Presence of SRAs aid in the survival of parasites, elongating the length and path of infection in a host. SRAs have the potential to evade cell lysis, preventing the breakdown of any present trypanosomes.
An important mechanism of T. brucei parasites is their flagellum. The flagellum is essential for parasite motility, pathogenesis, transmission of infection as well as immune evasion. Their flagellum is surrounded by its own membrane and attaches by length to the trypanosome cell body [10]. This wrap-around is vital for trypanosomes to invade other tissues throughout the body, given its extracellular characteristics. Moreover, T.brucei contain a flagellar pocket which is essential for the export of VSGs to other tissues throughout the body as well as the protection of extracellular macromolecules from the host’s immune response [10]. The flagellum oversees host cell attachment through attachment plaques, allowing parasites to develop a VSG coat [10]. Once coated, parasites are released into salivary glands, unleashing infection within the host.
Epidemiology
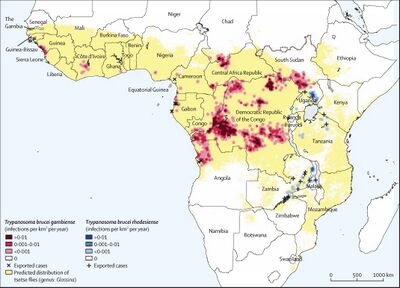
African Sleeping Sickness, or HAT infection is limited to regions of Sub-Saharan Africa specifically. There are two strains of Trypanosoma that infect humans, each infecting separate regions of Africa. T.b. gambiense, is centered in Central and West Africa while T.b. rhodiense is found in the southern and eastern portions of Africa [3]. Poor and rural communities face an increased risk due to frequent interaction with the land and water which are breeding grounds for bacteria.
Previous epidemics of Human African Trypanosomiasis have been documented in Uganda, Congo, and Cameroon, all of which are located in Central Africa [3]. As of late, most reported cases of T.b gambiense are found in the Democratic Republic of Congo and continue to decrease at a steady rate, hovering in the low thousands [8]. Popular tourist destinations such as Kenya, Malawi, Uganda, and Zambia may also notice an increase in T.b. rhodesiense cases due to constant visitation [3]. Travelers still face an increased risk of possible infection, especially those staying in areas of wildlife and nature preserves.
Symptoms

Human African Trypanosomiasis is commonly distinguished between two stages, hemolymphatic and neurological [2]. The hemolymphatic stage falls under a “stage one” diagnosis where the parasite has yet to cross the blood-brain barrier, infiltrating the CNS. The neurological stage is considered “stage two” and occurs once the parasite has colonized within the CNS. T.b gambiense is known to take longer to work its way through the host, sometimes taking months to progress into the latter stages of the disease. T.b rhodisiense, which only occurs in about 2% of HAT cases, spreads its infection much quicker and can develop into the neurological phase within 1-2 weeks [1].
Beginning symptoms to those who are infected by the Trypanosoma brucei parasite may be considered moderate such as itching of the skin, low-grade fever, facial swelling, or headache. The development of a chancre, a specific type of skin lesion, is a common initial symptom. Swollen lymph nodes are another sign that that parasite is working its way towards the central nervous system [2]. If left untreated for an extended amount of time,infected patients may begin to experience neurological symptoms such as trouble sleeping, irregularities in circadian rhythm, and nocturnal insomnia [1]. Patients in the second stage of HAT may experience cognitive troubles including, but not limited to, memory loss, dementia, depression and mania, increased irritability, and mood swings [1]. Other neurological symptoms include severe dehydration, tremors in the hands, muscle and motor weakness, along with a potential for slurred speech [3].
In regards to gender, females may experience inconsistent patterns in their menstrual cycles, while men may be more prone to erectile dysfunction [2]. Majority of those infected are unaware until they begin to experience neurological symptoms, the second stage of HAT which can range, taking anywhere from weeks to months to transpire. Rate of infection varies on the host however, if left untreated both T.b. gambiense and T.b. rhodesiense can be fatal, ending in death.
Pathogenesis
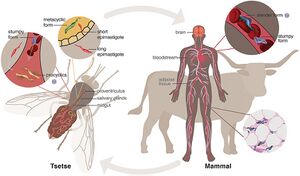
Path of Infection
African Trypanosomiasis infection first stems from a bite of the tsetse fly, a member of the Glossina genus. The T.brucei parasite lives in the salivary glands of the glossina species.
Tsetse flies only become infected with T. brucei when trypanosomes are ingested in a blood meal of another species [3]. After ingestion takes place, trypanosomes undergo a series of physical changes until they arrive at the salivary glands. The trypanosome cell develops into its infective metacyclic form which is considered to be its proper form [3]. Eventually, when the saliva of a tsetse fly comes into contact with the host epithelium, it inserts its infected trypanosome cells into the human bloodstream. This contact induces stage one symptoms of sleeping sickness.
Infection first arises in the skin giving off the appearance of a chancre. As trypanosomes multiply, they are able to extend infection via lymph and blood cells to essential organs [3]. Once the parasite is able to reach up through the lymph nodes, the infection spreads all through the host, colonizing in the central nervous system [1]. As the disease works its way through the host, it compromises the strength of the blood-brain barrier of the CNS, resulting in a decline in various neurocognitive functions. In rare cases, the transmission of the parasite may occur sexually, or through blood transfusions but only few have been reported [5].
Impact on the Central Nervous System
The central nervous system is composed of the spinal cord and the brain. The CNS contains cerebrospinal fluid acting as a barrier between these to protect the brain and cord from any major damage or potential injury. The blood-brain barrier is another semipermeable membrane between the brain and the blood, an important barrier in T.brucei parasite infection. It is still unclear as to how trypanosomes are able to penetrate the blood-brain barrier of the CNS as it has proven to be resistant to microbes. One possibility is penetrability through barrier inflammation when trypanosomes are present [7]. Trypanosomes are also thought to enter via the choroid plexus, a network of various blood vessels in the ventricles of the brain. Trypanosomes have the potential to damage the choroid plexus, allowing parasites to penetrate the ventricles, up through the blood-barrier region [1]. Entry into the blood-barrier region can induce a variety of neurological symptoms in those who have been infected by T.brucei parasites. Infection of the CNS ultimately establishes a slow demyelinating process which, unless treated, will result in death of the infected patient [1].
Diagnosis & Treatment
Diagnosis
Diagnosis can be challenging in the early stages of HAT as many of the initial corresponding symptoms such as fever and headache can be associated with a number of different diseases. Given that there are also varying neurological symptoms in later stages, diagnosing progression and stage of the infection is another challenge. In most cases, diagnosis of the late stages requires distinguishing the parasite from either tissue or blood sampling. For an initial diagnosis, patients may receive serological antibody tests in addition to blood tests to test potential trypanosome infection [1]. Test results would have to exhibit parasite antigens that result from the host’s immune response.
Samples of cerebrospinal fluid may be analyzed via lumbar puncture for biological changes to diagnose a specific stage in an HAT patient [1]. If no trypanosomes are detected in the cerebrospinal fluid, they are deemed to be in the first stage while trypanosomes detected would instigate a second stage diagnosis [3]. Given the long term effects and toxicity of current treatment plans, it is essential for HAT to be diagnosed as early as possible.
Treatment
Treatment of Human African Trypanosomiasis varies by stage and is most commonly done by the administration of drugs. Early diagnosis is essential to the health of the overall patient as the later the stage, the more toxic the drug is to the body [3]. Earlier treatment also increases the patient's likelihood of survival of sleeping sickness. The most frequently used drugs for treatment of HAT in the first stage consist of pentamidine and suramin while those in the second stage are typically administered melarsoprol.
First Stage Treatment:
Pentamidine is one of the first drugs used to cure HAT patients who are not experiencing any symptoms related to the central nervous system. Pentamidine is an injection typically given via intramuscular injection but could also be delivered through intravenous infusion [3]. Patients may experience swelling around the injection site, a common symptom of intravenous puncture. Common side effects of pentamidine treatment lie in the gastrointestinal regions but may also cause hypotension [8]. Another drug, suramin is used for treatment of T.b. rhodesiense and tends to be more extensive than pentamidine. Similarly, suramin is administered intravenously and may bring about adverse effects such as anemia, neuropathy, pyrexia, and a decrease in blood cell and platelet counts [3].
Second Stage Treatment:
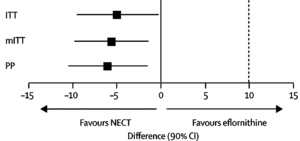
Regardless of strain, second stage treatment is dangerous and can result in long-term harmful effects to the human body. Melarsoprol, first discovered in 1949, is an injection administered intravenously, accumulating in the cerebrospinal fluid, killing trypanosomes in its vicinity [1]. Parasites located elsewhere throughout the body may not be affected by the administration of melarsoprol, giving rise to its 10% failure rate [1]. Melarsoprol is typically administered to second stage patients with the T.b. rhodesiense parasite as it tends to develop rapidly in the host. Melarsoprol causes several adverse effects and is considered to be extremely toxic to the body. One of its more severe effects is encephalopathy, which develops in 5-10% of HAT patients, often resulting in death [8]. Encephalopathy produces neurological symptoms such as memory loss, personality changes, and often stems from infection. In HAT, the result is often coma, seizures, and cerebral edema [8]. Others may experience heart complications or rash and skin infections. Although melarsoprol has proven to be effective for treatment of second stage HAT, its high mortality rate has encouraged researchers to look elsewhere.
As of 2009, another treatment, NECT (nifurtimox-eflornithine combination therapy) has been approved by the World Health Organization as treatment for second stage T.b. gambiense HAT [6]. Studies have shown nifurtimox has significantly higher cure rates (~95%), little to no fatality rates as well as less side effects [3]. Rather than injection, it is given orally which is much easier to administer. NECT administration is less time consuming with fewer infusions, and has since been the preferred method of treatment [6]. Side effects consist of nausea, headache, and abdominal pain. Nifurtimox has not been approved for use in African trypanosomiasis, only American; however, it can be administered to patients off label if approved [3]. Melarsoprol is considered to have the highest fatality risk hence it is only administered when deemed absolutely necessary.
Prevention
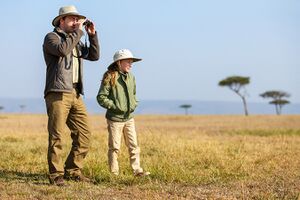
As of late, there are limited options for clinical prevention of African trypanosomiasis. There is currently no approved prevention through the use of drugs or vaccines, majority is done through self-prevention. Individuals who feel they may be at risk of infection can reduce their chances by protecting their skin through long pants and shirts, as well as visit areas that do not have tsetse fly infestation. When risk of infection is high, prevention of T.b. gambiense is achieved through early diagnosis and treatment, decreasing the likelihood of transmission and further infection.
A time consuming but effective method of preventing T.b. gambiense is through case screening individuals who live in “high risk” areas. Some regions have implemented the use of the Card Agglutination Test for Trypanosomiasis (CATT) which allows for screening of the presence of trypanosomes [8]. There are some drawbacks to this method as it quite frequently delivers false positives, which could initiate a “false” epidemic [8]. In addition, screening requires intensive training therefore it is considered to be not effective in areas where the disease is not currently prevalent [3]. Regardless, constant screening in communities that may be at risk may slowly eliminate the disease as those infected are identified early on.
Researchers are continuing to find reliable and cost effective methods to identify hosts infected with trypanosomiasis. A far-fetched but more permanent solution would be complete elimination of the tsetse fly, however researchers are confident that alternative prevention methods are attainable in eliminating HAT [3].
Current Research & Next Steps
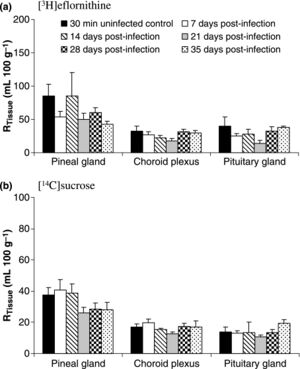
Research for more effective treatments and prevention of African trypanosomiasis is still prevalent, despite the fact that there has been a recent decline in cases diagnosed each year. Less toxic treatment options for second stage HAT have been in recent development, one of them being centered around the drug fexinidazole [8]. Current studies have shown oral administration of fexinidazole has succession rates ~90%, similar to the succession rates of NECT [8]. Oral administration is becoming the preferred method of HAT treatment due to its overall simplicity and effectiveness.
Animal models of late stage HAT have also played a crucial role in understanding the effects drugs have on African trypanosomiasis. Animals have allowed researchers to evaluate the neuropathogenesis of HAT as well as ways to counteract the disease upon entering into the central nervous system [9]. Rodents are used in discovering less toxic drugs, and alternative treatments that are able to cross the blood brain barrier (BBB) which is essential in late stage HAT treatment [9]. Researchers have acknowledged that further studies need to be conducted on assessment of the BBB in order to develop drugs strong enough to penetrate it without inflicting harm on the host.
Conclusion
Although numbers have shown to decrease over recent years, Human African Trypanosomiasis still poses a risk to African communities. Considering mechanisms of HAT are still not fully understood, communities may be severely underprepared for disease control if there were to be a break out in cases. Researchers continue to work on understanding the mechanisms behind the trypanosoma brucei species that allow it to pass through various tissues throughout the human body. Specifically, future research is needed to evaluate how the T.brucei parasite can infiltrate the blood-brain barrier and other CNS involvement.
Americans who plan visits to Sub-Saharan African countries also still face a significant risk of contracting the disease, potentially carrying it back into the country. Until the disease has a more permanent and less harmful cure or is completely eliminated, there are still possibilities for an infection to break out anywhere in the country. As this disease tends to target lower-income communities, it is essential for clinicians to continue to work towards antibiotic resistance for HAT and continue to provide medical assistance until there is no longer a risk of trypanosome infection.
References
[1] Enanga, B., Burchmore, R. J., Stewart, M. L., & Barrett, M. P. (2002). Sleeping sickness and the brain. Cellular and molecular life sciences, 59(5) pp. 845–858. From: https://doi.org/10.1007/s00018-002-8472-0
[2] Crilly, N.P, & Mugnier, M.R. (2021). Thinking outside the blood: Perspectives on tissue-resident Trypanosoma brucei, PLOS, 17(9). From: https://doi.org/10.1371/journal.ppat.1009866
[3] Büscher, P, Cecchi, G, Jamonneau, V, Priotto, G. (2017). Human African trypanosomiasis,
Lancet, 390 (10110) pp. 2397-2409. From: https://doi.org/10.1016/s0140-6736(17)31510-6
[4] Priotto G, Kasparian S, Mutombo W, et al.(2009). Nifurtimox-eflornithine combination therapy for second-stage AfricanTrypanosoma brucei gambiense trypanosomiasis: a multicentre, randomised, phase III, non-inferiority trial. Lancet, 374 pp. 56–64. From: https://doi.org/10.1016/s0140-6736(09)61117-x
[5] Herwaldt B. L. (2001). Laboratory-acquired parasitic infections from accidental exposures. Clinical microbiology reviews, 14(4) pp. 659–688. From: https://doi.org/10.1128/CMR.14.3.659-688.2001
[6] Sudarshi, D., Lawrence, S., Pickrell, W. O., Eligar, V., Walters, R., Quaderi, S., Walker, A., Capewell, P., Clucas, C., Vincent, A., Checchi, F., MacLeod, A., & Brown, M. (2014). Human African trypanosomiasis presenting at least 29 years after infection--what can this teach us about the pathogenesis and control of this neglected tropical disease?. PLoS neglected tropical diseases, 8(12), pp. 3349. From: https://doi.org/10.1371/journal.pntd.0003349
[7] Bisser, S., Ayed, Z., Bouteille, B., Stanghellini, A., Breton, J. C., Dumas, M., & Jauberteau, M. O. (2000). Central nervous system involvement in African trypanosomiasis: presence of anti-galactocerebroside antibodies in patients' cerebrospinal fluid. Transactions of the Royal Society of Tropical Medicine and Hygiene, 94(2), pp. 225–226. From: https://doi.org/10.1016/s0035-9203(00)90285-2
[8] Kennedy, P. G. E., & Rodgers, J. (2019). Clinical and Neuropathogenetic Aspects of Human African Trypanosomiasis. Frontiers in immunology, 10 (39). From: https://doi.org/10.3389/fimmu.2019.00039
[9] Kennedy P. G. (2006). Diagnostic and neuropathogenesis issues in human African trypanosomiasis, International journal for parasitology, 36(5) pp. 505–512. From: https://doi.org/10.1016/j.ijpara.2006.01.012
[10] Ralson, K.S., Kabututu, Z.P., Melehani, J.H., Oberholzer, M, & Hill, K.L. (2009). The Trypanosoma brucei Flagellum: Moving Parasites in New Directions, Annual Review of Microbiology, 63, pp. 335-362. From: https://doi-org.libproxy.kenyon.edu/10.1146/annurev.micro.091208.073353
[11] Berriman, M., Ghedin, E., Hertz-Fowler, C., Blandin, G., Renauld, H., Bartholomeu, D. C., Lennard, N. J., Caler, E., Hamlin, N. E., Haas, B., Böhme, U., Hannick, L., Aslett, M. A., Shallom, J., Marcello, L., Hou, L., Wickstead, B., Alsmark, U. C., Arrowsmith, C., Atkin, R. J., El-Sayed, N. M. (2005). The genome of the African trypanosome Trypanosoma brucei. Science (New York, N.Y.), 309(5733) pp. 416–422. From: https://doi.org/10.1126/science.1112642
[12] De Greef, C., & Hamers, R. (1994). The serum resistance-associated (SRA) gene of Trypanosoma brucei rhodesiense encodes a variant surface glycoprotein-like protein. Molecular and biochemical parasitology, 68(2) pp. 277–284. From: https://doi.org/10.1016/0166-6851(94)90172-4