Beta lactam antibiotics: Difference between revisions
Greischar1 (talk | contribs) No edit summary |
Greischar1 (talk | contribs) No edit summary |
||
(11 intermediate revisions by the same user not shown) | |||
Line 1: | Line 1: | ||
==Introduction== | ==Introduction== | ||
β-Lactam antibiotics are the most commonly used class of antibiotics and the first class of these drugs discovered. Alexander Fleming isolated the first β-lactam antibiotic, penicillin, in 1929 from a strain of Penicillium rubens | β-Lactam antibiotics are the most commonly used class of antibiotics and the first class of these drugs discovered. Alexander Fleming isolated the first β-lactam antibiotic, penicillin, in 1929 from a strain of Penicillium rubens (fig. 1). His accidental discovery of the drug revolutionized the treatment of bacterial infections; this class is now the most commonly prescribed injectable antibiotic in the US <ref>[doi: 10.1101/cshperspect.a025247 Bush K and Bradford PA. β-Lactams and β-Lactamase Inhibitors: An Overview. Cold Spring Harb Perspect Med. 2016 Aug 1;6(8):a025247.]</ref>. This family of drugs is named for their central 4-membered cyclic amide also called a lactam (fig. 2). Many investigations have converged upon the thesis that this ring, due to susceptibility for nucleophilic attack, irreversibly binds a serine residue on penicillin binding proteins, preventing complete synthesis of the bacterial cell wall<ref>[doi: 10.1021/cr030102i Fisher JF, Meroueh SO, and Mobashery S. Bacterial Resistance to β-Lactam Antibiotics: Compelling Opportunism, Compelling Opportunity Chemical Reviews 2005 105 (2), 395-424]</ref>. Due to the widespread use of this class of drugs, bacteria have evolved to resist them by producing the enzyme β-lactamase. | ||
[[Image:penicillium_rubens.jpg|thumb|300px|left|Figure 1. Macroscopic and microscopic appearance of <br><i>Penicillium rubens</i>.[https://doi.org/10.5598/imafungus.2011.02.01.12].]] | [[Image:penicillium_rubens.jpg|thumb|300px|left|Figure 1. Macroscopic and microscopic appearance of <br><i>Penicillium rubens</i>.[https://doi.org/10.5598/imafungus.2011.02.01.12].]] | ||
[[Image:lactams.png|thumb|300px|right|Figure 2. Lactams of various sizes. Made by the author in ChemDraw.]] | |||
==Historical Overview== | ==Historical Overview== | ||
In September 1928, Alexander Fleming returned to his laboratory after a vacation to find mold growing alongside bacteria in some petri dishes he had left out. A clear ring had formed around the blue-green mold where no bacteria was growing ( | In September 1928, Alexander Fleming returned to his laboratory after a vacation to find mold growing alongside bacteria in some petri dishes he had left out. A clear ring had formed around the blue-green mold where no bacteria was growing <ref name=" Bennett ">[https://doi.org/10.1016/S0065-2164(01)49013-7 Bennett JW and Chung KT. Alexander Fleming and the discovery of penicillin. Advances in Applied Microbiology. 2001;49:163-184.]</ref>. Fleming was interested in this result because several years prior, he discovered that his nasal mucus inhibited bacterial growth on a plate. This result was later found to be caused by an enzyme, rather than a small molecule, which Fleming named lysozyme <ref name=" Bennett "></ref>. Nevertheless, this discovery made Fleming perceptive enough to notice the effects of the mold on bacterial growth. | ||
In 1929, Fleming reported his findings in the British Journal of Experimental Pathology. In the article, Fleming made some attempts to characterize the antibacterial agent such as documenting the effects of boiling and filtering its concentrated solutions. He found that neither of these processes affected the antibacterial nature of the solution <ref>[http://www.jstor.org/stable/4452419. Flemming A.On the Antibacterial Action of Cultures of a Penicillium, with Special Reference to Their Use in the Isolation of B. Influenzae. Reviews of Infectious Diseases. 1980; 2(1):129–39.]</ref>. He tested varying dilutions of the stock solution and found that even when the mold juice was diluted 800 times, the growth of a staphylococcus species was still inhibited. Fleming used rabbits to test the toxicity of the concentrated solutions and found it to be well tolerated, suggesting that the drug could be useful for combating bacterial infections in humans. | |||
<ref name=" | In 1940, clinical experiments began at Oxford University to formulate penicillin as a drug for human medicine <ref name=" Bennett "></ref>. While Flemming initially thought penicillin was only effective as a topical anesthetic, the Oxford studies quickly found that it could also be injected safely or taken as an oral medication. The English biochemist Edward Abraham was the first to propose a chemical structure of penicillin, but Dorothy Hodgkin was able to determine its precise structure using x-ray crystallography. In 1941 Howard Florey and Norman Heatley of the Oxford research group travelled to the US to persuade the USDA to begin manufacturing penicillin at a large scale. Production started and the drug became available to the public by 1945. Current production methods are essentially the same as the first industrial processes of the 1940s–the fungus Penicillium rubens is grown in large tanks of liquid culture containing sugars and the penicillin produced by biosynthesis is then purified. | ||
==Mechanism of action== | |||
β-Lactam antibiotics are able to disrupt cell wall synthesis in both Gram-negative and Gram-positive bacteria. This effect is the result of covalent binding to penicillin-binding proteins, enzymes which mediate the final steps of peptidoglycan cross-linking. Peptidoglycan is a protective exoskeleton which is found in Gram-negative and Gram-positive bacteria (fig.3)[[Image:peptido.jpg|thumb|300px|right|Figure 3. Peptidoglycan structure.[https://doi.org/10.1128/ecosalplus.ESP-0010-2020].]]. It is composed of glycan strands with repeating disaccharide units and peptide chains of two to five amino acid residues. The glycan strands and peptide chains cross link to form a mesh-like structure that surrounds the cytoplasmic membrane, which prevents lysis, maintains cell shape, and protects the cell interior from environmental conditions. This final cross-linking step is mediated by DD-transpeptidases or penicillin-binding proteins, which, as their name suggests, are susceptible to inhibition by penicillin and other β-lactam-containing antibiotics. | |||
The structure of penicillin is analogous to the acyl-D-alanyl-D-alanine residues found at the terminus of linear glycopeptides in the bacterial cell wall <ref>[doi: 10.1073/pnas.54.4.1133 Tipper DJ and Strominger JL. Mechanism of action of penicillins: a proposal based on their structural similarity to acyl-D-alanyl-D-alanine. Proc Natl Acad Sci U S A. 1965 Oct;54(4):1133-41.]</ref>. It is this structural similarity which enables penicillin-like antibiotics to mimic the natural ligand for DD-transpeptidases. Studies in the 1940s and 50s determined that penicillin did not impact the formation of linear glycopeptides, only the cross-linking between them; without cross-linking the cell wall falls apart and the cell is lysed <ref name=" Garde ">[doi: 10.1128/ecosalplus.ESP-0010-2020. Garde S, Chodisetti PK, Reddy M. Peptidoglycan: Structure, Synthesis, and Regulation. EcoSal Plus. 2021 Jan;9(2):eESP-0010-2020.]</ref>. Due to ring strain, β-lactams are prone to ring opening upon nucleophilic attack. This is what happens when the antibiotic reaches the active site of a penicillin binding protein: non-covalent interactions stabilize the molecule while also positioning it for nucleophilic attack by a serine residue on the protein. This process acylates the residue, changing the structure of the protein and denaturing the protein rendering it useless. | |||
There are two parameters related to the molecular structure of β-lactam antibiotics which predict their potency. Both of these parameters influence the lability of the β-lactam ring, making it even less stable and prone to attack from nucleophilic amino acid residues. The first parameter is the height (in Å) of the pyramid formed by the β-lactam N and the two adjacent C atoms <ref name=" Garde "></ref>. This measures the extent to which the four-membered ring is bent out of planarity. It is unclear whether the increased potency of antibiotics possessing a greater height for this measurement arises from improved binding at the penicillin-binding protein active site or greater instability in the lactam ring. The second parameter, called Cohen’s parameter, is the distance (in Å) between the oxygen of the carboxylic acid to the amide moiety of the lactam ring [[Image:Lactamcore.png|thumb|300px|left|Figure 4. General structure of penicillins (left) and cephalosporins (right) the most common β-lactam antibiotics. The β-lactam ring is shown in blue. Made by the author in ChemDraw.]]. This parameter not only determines the potency of a β-lactam antibiotic but also if it will be active at all: the biological active members of this class of molecules feature the carboxylic acid close to the amide in a pseudo axial conformation <ref>[http://doi.org/10.1098/rstb.1980.0042 Woodward RB. Penems and related substances. Phil. Trans. R. Soc. Lond. B 289:239–250.]</ref>]. | |||
== | ==Bacteria Resistance to β-lactam Antibiotics== | ||
The widespread use of antibiotics has allowed millions of generations of bacteria to encounter them and develop advantageous mutations against them. There are several mechanisms by which bacteria can resist these antibiotics: producing β-lactamase enzymes, altering the active site of penicillin-binding proteins, lowering expression of outer membrane proteins, and developing efflux pumps in the plasma membrane to pump out unwanted compounds <ref name=" Drawz ">[doi: 10.1128/CMR.00037-09. Drawz SM and Bonomo RA. Three decades of beta-lactamase inhibitors. Clin Microbiol Rev. 2010 Jan;23(1):160-201.]</ref>. Of these strategies, the production of β-lactamase enzymes is the most important and common route of resistance–particularly in Gram-negative bacteria. Interestingly, the first β-lactamase was identified by E. P. Abraham and E. Chain in E. Coli before penicillin was developed for clinical use <ref name=" Drawz "></ref>. Although the human use of these antibiotics has rapidly accelerated the process of the bacterial resistance, these compounds were originally produced by microorganisms in nature so this naturally occurring resistance is somewhat unsurprising. | |||
The serine class of these enzymes possess a similar active site to penicillin-binding proteins, meaning they compete for binding by β-lactam antibiotics. Once the antibiotic is in the active site, the enzyme acylates it then hydrolyzes it, rendering it inactive (fig 5).[[Image:Lactamasemech.png|thumb|400px|left|Figure 5. Proposed mechanism of the reaction between β-lactam antibiotic substrate and a serine β-lactamase enzyme. [https://doi.org/10.1128/CMR.00037-09].]]. To counter this problem β-lactamase inhibitors have been developed to prevent the enzyme from destroying an antibiotic. | |||
==Conclusion== | ==Conclusion== | ||
β-lactam antibiotics have shaped the treatment of bacterial infections, saving countless lives since their introduction in the 1940s. The inhibition of penicillin-binding proteins and disruption of cell wall synthesis, remains the cornerstone of their efficacy. However, the rapid evolution of bacterial resistance, particularly through the production of β-lactamase enzymes, presents an ongoing challenge to medicine. Understanding the molecular dynamics of β-lactam activity and resistance is crucial for developing new strategies to combat resistant strains. Despite these challenges, β-lactam antibiotics continue to be a vital tool in modern medicine. | |||
==References== | ==References== | ||
<references /> | <references /> | ||
<br>Edited by [ | <br>Edited by [Greyson Greischar], student of Joan Slonczewski for BIOL 116, 2024, [http://www.kenyon.edu/index.xml Kenyon College]. | ||
<!--Do not edit or remove this line-->[[Category:Pages edited by students of Joan Slonczewski at Kenyon College]] | <!--Do not edit or remove this line-->[[Category:Pages edited by students of Joan Slonczewski at Kenyon College]] |
Latest revision as of 00:45, 13 December 2024
Introduction
β-Lactam antibiotics are the most commonly used class of antibiotics and the first class of these drugs discovered. Alexander Fleming isolated the first β-lactam antibiotic, penicillin, in 1929 from a strain of Penicillium rubens (fig. 1). His accidental discovery of the drug revolutionized the treatment of bacterial infections; this class is now the most commonly prescribed injectable antibiotic in the US [1]. This family of drugs is named for their central 4-membered cyclic amide also called a lactam (fig. 2). Many investigations have converged upon the thesis that this ring, due to susceptibility for nucleophilic attack, irreversibly binds a serine residue on penicillin binding proteins, preventing complete synthesis of the bacterial cell wall[2]. Due to the widespread use of this class of drugs, bacteria have evolved to resist them by producing the enzyme β-lactamase.
Historical Overview
In September 1928, Alexander Fleming returned to his laboratory after a vacation to find mold growing alongside bacteria in some petri dishes he had left out. A clear ring had formed around the blue-green mold where no bacteria was growing [3]. Fleming was interested in this result because several years prior, he discovered that his nasal mucus inhibited bacterial growth on a plate. This result was later found to be caused by an enzyme, rather than a small molecule, which Fleming named lysozyme [3]. Nevertheless, this discovery made Fleming perceptive enough to notice the effects of the mold on bacterial growth.
In 1929, Fleming reported his findings in the British Journal of Experimental Pathology. In the article, Fleming made some attempts to characterize the antibacterial agent such as documenting the effects of boiling and filtering its concentrated solutions. He found that neither of these processes affected the antibacterial nature of the solution [4]. He tested varying dilutions of the stock solution and found that even when the mold juice was diluted 800 times, the growth of a staphylococcus species was still inhibited. Fleming used rabbits to test the toxicity of the concentrated solutions and found it to be well tolerated, suggesting that the drug could be useful for combating bacterial infections in humans.
In 1940, clinical experiments began at Oxford University to formulate penicillin as a drug for human medicine [3]. While Flemming initially thought penicillin was only effective as a topical anesthetic, the Oxford studies quickly found that it could also be injected safely or taken as an oral medication. The English biochemist Edward Abraham was the first to propose a chemical structure of penicillin, but Dorothy Hodgkin was able to determine its precise structure using x-ray crystallography. In 1941 Howard Florey and Norman Heatley of the Oxford research group travelled to the US to persuade the USDA to begin manufacturing penicillin at a large scale. Production started and the drug became available to the public by 1945. Current production methods are essentially the same as the first industrial processes of the 1940s–the fungus Penicillium rubens is grown in large tanks of liquid culture containing sugars and the penicillin produced by biosynthesis is then purified.
Mechanism of action
β-Lactam antibiotics are able to disrupt cell wall synthesis in both Gram-negative and Gram-positive bacteria. This effect is the result of covalent binding to penicillin-binding proteins, enzymes which mediate the final steps of peptidoglycan cross-linking. Peptidoglycan is a protective exoskeleton which is found in Gram-negative and Gram-positive bacteria (fig.3)
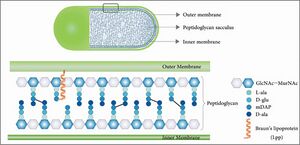
. It is composed of glycan strands with repeating disaccharide units and peptide chains of two to five amino acid residues. The glycan strands and peptide chains cross link to form a mesh-like structure that surrounds the cytoplasmic membrane, which prevents lysis, maintains cell shape, and protects the cell interior from environmental conditions. This final cross-linking step is mediated by DD-transpeptidases or penicillin-binding proteins, which, as their name suggests, are susceptible to inhibition by penicillin and other β-lactam-containing antibiotics.
The structure of penicillin is analogous to the acyl-D-alanyl-D-alanine residues found at the terminus of linear glycopeptides in the bacterial cell wall [5]. It is this structural similarity which enables penicillin-like antibiotics to mimic the natural ligand for DD-transpeptidases. Studies in the 1940s and 50s determined that penicillin did not impact the formation of linear glycopeptides, only the cross-linking between them; without cross-linking the cell wall falls apart and the cell is lysed [6]. Due to ring strain, β-lactams are prone to ring opening upon nucleophilic attack. This is what happens when the antibiotic reaches the active site of a penicillin binding protein: non-covalent interactions stabilize the molecule while also positioning it for nucleophilic attack by a serine residue on the protein. This process acylates the residue, changing the structure of the protein and denaturing the protein rendering it useless.
There are two parameters related to the molecular structure of β-lactam antibiotics which predict their potency. Both of these parameters influence the lability of the β-lactam ring, making it even less stable and prone to attack from nucleophilic amino acid residues. The first parameter is the height (in Å) of the pyramid formed by the β-lactam N and the two adjacent C atoms [6]. This measures the extent to which the four-membered ring is bent out of planarity. It is unclear whether the increased potency of antibiotics possessing a greater height for this measurement arises from improved binding at the penicillin-binding protein active site or greater instability in the lactam ring. The second parameter, called Cohen’s parameter, is the distance (in Å) between the oxygen of the carboxylic acid to the amide moiety of the lactam ring
. This parameter not only determines the potency of a β-lactam antibiotic but also if it will be active at all: the biological active members of this class of molecules feature the carboxylic acid close to the amide in a pseudo axial conformation [7]].
Bacteria Resistance to β-lactam Antibiotics
The widespread use of antibiotics has allowed millions of generations of bacteria to encounter them and develop advantageous mutations against them. There are several mechanisms by which bacteria can resist these antibiotics: producing β-lactamase enzymes, altering the active site of penicillin-binding proteins, lowering expression of outer membrane proteins, and developing efflux pumps in the plasma membrane to pump out unwanted compounds [8]. Of these strategies, the production of β-lactamase enzymes is the most important and common route of resistance–particularly in Gram-negative bacteria. Interestingly, the first β-lactamase was identified by E. P. Abraham and E. Chain in E. Coli before penicillin was developed for clinical use [8]. Although the human use of these antibiotics has rapidly accelerated the process of the bacterial resistance, these compounds were originally produced by microorganisms in nature so this naturally occurring resistance is somewhat unsurprising.
The serine class of these enzymes possess a similar active site to penicillin-binding proteins, meaning they compete for binding by β-lactam antibiotics. Once the antibiotic is in the active site, the enzyme acylates it then hydrolyzes it, rendering it inactive (fig 5).
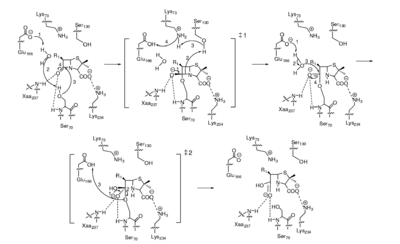
. To counter this problem β-lactamase inhibitors have been developed to prevent the enzyme from destroying an antibiotic.
Conclusion
β-lactam antibiotics have shaped the treatment of bacterial infections, saving countless lives since their introduction in the 1940s. The inhibition of penicillin-binding proteins and disruption of cell wall synthesis, remains the cornerstone of their efficacy. However, the rapid evolution of bacterial resistance, particularly through the production of β-lactamase enzymes, presents an ongoing challenge to medicine. Understanding the molecular dynamics of β-lactam activity and resistance is crucial for developing new strategies to combat resistant strains. Despite these challenges, β-lactam antibiotics continue to be a vital tool in modern medicine.
References
- ↑ [doi: 10.1101/cshperspect.a025247 Bush K and Bradford PA. β-Lactams and β-Lactamase Inhibitors: An Overview. Cold Spring Harb Perspect Med. 2016 Aug 1;6(8):a025247.]
- ↑ [doi: 10.1021/cr030102i Fisher JF, Meroueh SO, and Mobashery S. Bacterial Resistance to β-Lactam Antibiotics: Compelling Opportunism, Compelling Opportunity Chemical Reviews 2005 105 (2), 395-424]
- ↑ 3.0 3.1 3.2 Bennett JW and Chung KT. Alexander Fleming and the discovery of penicillin. Advances in Applied Microbiology. 2001;49:163-184.
- ↑ Flemming A.On the Antibacterial Action of Cultures of a Penicillium, with Special Reference to Their Use in the Isolation of B. Influenzae. Reviews of Infectious Diseases. 1980; 2(1):129–39.
- ↑ [doi: 10.1073/pnas.54.4.1133 Tipper DJ and Strominger JL. Mechanism of action of penicillins: a proposal based on their structural similarity to acyl-D-alanyl-D-alanine. Proc Natl Acad Sci U S A. 1965 Oct;54(4):1133-41.]
- ↑ 6.0 6.1 [doi: 10.1128/ecosalplus.ESP-0010-2020. Garde S, Chodisetti PK, Reddy M. Peptidoglycan: Structure, Synthesis, and Regulation. EcoSal Plus. 2021 Jan;9(2):eESP-0010-2020.]
- ↑ Woodward RB. Penems and related substances. Phil. Trans. R. Soc. Lond. B 289:239–250.
- ↑ 8.0 8.1 [doi: 10.1128/CMR.00037-09. Drawz SM and Bonomo RA. Three decades of beta-lactamase inhibitors. Clin Microbiol Rev. 2010 Jan;23(1):160-201.]
Edited by [Greyson Greischar], student of Joan Slonczewski for BIOL 116, 2024, Kenyon College.