Ophiocordyceps Fungal Invasion of Ants: Difference between revisions
Tag: Manual revert |
|||
(47 intermediate revisions by the same user not shown) | |||
Line 1: | Line 1: | ||
==Introduction== | ==Introduction== | ||
Parasitic ant-fungal relationships have been present since the Eocene epoch 45 million years | [[Image:Cyclefig1.webp|thumb|300px|left|Figure 1. Cycle of infection of <i>Ophiocordyceps</i> fungi on ants as hosts. [https://www.the-scientist.com/zombie-fungi-hijack-hosts-brains-72271].]] | ||
[[Image:Fig6b.jpg|thumb|200px|right|Figure 2. <i>O. kimflemingiae</i> infected <I>C. castaneus</i> ant. Fruiting body visible and ant displays biting and grasping behaviors. [https://www.the-scientist.com/zombie-fungi-hijack-hosts-brains-72271].]] | |||
Parasitic ant-fungal relationships have been present since the Eocene epoch 45 million years ago<ref name=" bdefense "></ref>. These relationships, while similar in nature and spanning the order Hymenoptera, are the result of a coevolution between parasite and host resulting in highly specific mechanisms of action. The genus <i>Ophiocordyceps</i> falls into this category of entomopathogens, fungi that parasitize insects, and are commonly identified as the "Zombie-ant fungus". While each individual species of <i>Ophiocordyceps</i> infects a different host ant species, the method of fungal reproduction and spreading of spores remains the same (Fig. 1). Fungal spores penetrate the protected cuticle of the ant and begin to reproduce within internal ant tissues. After a certain period of time, usually after a few days, the infected ant will leave the colony, abandon its tasks, climb a vegetative structure, and bite onto the leaf, stem, or branch with its mandibles. Here, the ant will die, remaining latched on, as the fungus completes its life cycle by growing a fruiting body from the ant cadaver to disperse spores (Fig. 2).<br> | |||
<br><br> | |||
<br><br> | |||
<br><br> | |||
<br><br> | |||
<br><br> | |||
<br><br> | <br><br> | ||
==Fungal Microbiome== | |||
< | [[Image:Sclerotiamicrobiome.jpeg|thumb|300px|right|Figure 3. Identified bacteria with sclerotia of <I>C. militaris</i>[https://pmc.ncbi.nlm.nih.gov/articles/PMC8627653/].]] | ||
Parasitic fungi are often hosts to their "gut microbiome" in the form of a sclerotia. Many microorganisms can be identified in fungal sclerotia, a hardened fungal body that stores nutrients and other resources when the fungus encounters non-ideal survival conditions. A study conducted on <I>Cordyceps militaris</i> sclerotia, another ant-infecting entomopathogen closely related to <i>Ophiocordyceps</i>, measured bacteria and their function present<ref name=" cordybact ">[https://pmc.ncbi.nlm.nih.gov/articles/PMC8627653/ Luo L, Zhou J, Xu Z, Guan J, Gao Y, Zou X. Identification and functional analysis of bacteria in sclerotia of Cordyceps militaris. PeerJ. 2021 Nov 25;9:e12511.]</ref>. In completing a transcriptomic analysis of 16S rRNA and creating a phylogenetic tree of bacteria present in fungal sclerotia, researchers identified two focal strains, <i>Stenotrophomonas maltophilia</i> and <I>Pseudomonas baetica</i>, among a wealth of others (Fig. 3). It was identified through co-culturing techniques that some bacteria were also parasitic to the insect hosts themselves or promoted fungal digestion through enzyme secretion, while others exhibited symbiosis with insect hosts as they function to inhibit fungal growth. In housing a microbiome that produces antimicrobial compounds, parasitic fungi are able to ensure an effective infection of their host while also monitoring their own growth and virulence in order to keep their host alive for proper development. It can be inferred that the genus <i>Ophiocordyceps</i> displays a similar microbiome in order to regulate infection of a host, due to the evolutionary proximity of the two genera and shared trait of entomopathogenicity.<br> | |||
< | <br><br> | ||
< | <br><br> | ||
< | <br><br> | ||
< | <br><br> | ||
< | <br><br> | ||
<br><br> | |||
<br>< | |||
<br>< | |||
<br>< | |||
<br>< | |||
<br> <br> | ==Host Defenses== | ||
Ants belong to the order Hymenoptera along with several other parasitic or eusocial insects. Eusocial insects have evolved to live in populous colonies and complete certain tasks as a part of a caste system. The colonies these insects inhabit are prime breeding grounds for parasites because of the high population density and temperature within a nest<ref name=" gdefense ">[https://www-sciencedirect-com.libproxy.kenyon.edu/science/article/pii/S0889159108000755 Stowe A, Beattie A. Chemical and genetic defenses against disease in insect societies. ScienceDirect. 2008 Oct;22(7):1009–13.]</ref>.<br> | |||
[[Image:Phylofig5.jpg|thumb|300px|right|Figure 4. Phylogeny of Hymenoptera. Green branches represent parasitoidism and orange branches represent nest construction, both ways Hymenoptera feed, live, and reproduce. Asterisks mark eusocial insects, though only some species of <I>Apoidea</i> and <I>Vespidae</i> exhibit this behavior, opposed to <I>Formicidae</I> that all exhibit this behavior. [https://www.sciencedirect.com/science/article/pii/S0960982213010567].]] | |||
As a form of evolutionary defense, Hymenoptera have formed a symbiotic relationship with Actinobacteria that produce antimicrobial metabolites<ref name=" actino ">[https://pmc.ncbi.nlm.nih.gov/articles/PMC5651009/ Matarrita-Carranza B, Moreira-Soto RD, Murillo-Cruz C, Mora M, Currie CR, Pinto-Tomas AA. Evidence for Widespread Associations between Neotropical Hymenopteran Insects and Actinobacteria. Frontiers in Microbiology. 2017 Oct 17;8.]</ref>. Worker ants are most exposed to entomopathogens because of the tasks they perform traveling into and out of the nest<ref name=" gdefense "></ref>. As a result, worker ants have evolved to secrete antimicrobial compounds from metapleural glands at the posterior of the thorax. These glands are present only among worker ants and not male ants that reside within the nest, which demonstrates the coevolution of entomopathogens and their hosts over time. Leaf-cutter ants exhibit another form of antimicrobial bacterial symbiosis. Leaf-cutter ants return to their nest with vegetation to add to the fungal garden within the nest (Fig. 5). Leaf-cutter ants cannot digest the leaves so they employ a symbiotic fungus which transforms the leaves into a glycogen-rich digestible compound. The mycoparasite, <i>Escovopsis</i>, poses a threat to these leaf-cutter ants as they parasitize the fungi that create this fungal garden. In another example of coevolution between parasites and hosts, leaf-cutter ants house a symbiotic bacteria that produces antifungal metabolites to combat <I>Escovopsis</i>.<br> | |||
[[Image:Antscanfig3.jpg|thumb|600px|left|Figure 5. Scanning electron microscopy of Leaf-cutter Ant. Identification of symbiotic <I>Pseudonocardia</i> filamentous bacteria present in ant cuticle. [https://www.frontiersin.org/journals/microbiology/articles/10.3389/fmicb.2016.02073/full].]] | |||
<br><br> | |||
<br><br> | |||
<br><br> | |||
<br><br> | |||
<br><br> | |||
<br><br> | |||
<br><br> | |||
<br><br> | |||
Hymenoptera have also developed behavioral and social defenses against entomopathogenic infection<ref name=" gdefense "></ref> (Fig. 4). Eusocial insects have adopted multimating, colony intermating, and kin avoidance behaviors in order to boost genetic diversity within colonies to increase the difficulty of entomopathogenic reproduction and infection. While these behaviors result in a net positive for the colony, multimating behaviors have shown to increase rates of sexually transmitted infection. These evolutionary advantages that increase genetic diversity are selected despite the negative implication of increased sexual transmission of pathogens.<br> | |||
It is possible the trait of eusociality evolved as functions to limit entomopathogenic infection in conjunction with the evolution of individual antimicrobial strength<ref name=" gdefense "></ref>. | |||
== | ==Genetic Basis and Possible Methods of Function== | ||
[[Image:Fig6a.jpg|thumb|300px|left|Figure 6. <I>O. subramanianii</i> infects ants and induces biting behaviors on vertical stems on vegetation close to the ground. [https://www.nature.com/articles/s41598-017-12863-w].]] | |||
<ref name=" | Once within a host, fungal spores secrete enterotoxins and active secondary metabolites that vary by fungal and host species. However, what chemically occurs and the cause is generally unknown during the fungal development, alteration of ant behavior, and attachment and fungal spore growth stages of infection. To further understand the methods of infection, researchers have performed comparative genomics tests on species of entomopathogens, pathogenic fungi, and non-pathogenic fungi in order to identify genes upregulated during biting events<ref name=" gcordy ">[https://www.nature.com/articles/s41598-017-12863-w de Bekker C, Ohm RA, Evans HC, Brachmann A, Hughes DP. Ant-infecting Ophiocordyceps genomes reveal a high diversity of potential behavioral manipulation genes and a possible major role for enterotoxins. Scientific Reports. 2017 Oct 2;7(1):1–13.]</ref>. By also comparing the transcriptomes of <i>Ophiocordyceps</i> pre, post, and during infection, researchers identified a gene of interest that functions in the production of secondary metabolites. Results identified that, although entomopathogens alone secrete enterotoxins, 78% of the <i>Ophiocordyceps</i> genome was shared with non-pathogenic fungi and no novel genes were present in entomopathogens. Gene sequence between entomopathogens and species within <i>Ophiocordyceps</i> also varied greatly despite occupying and utilizing similar secondary metabolites and pathways. This suggests that entomopathogens have evolved to co-opt evolutionarily-present fungal genes for parasitization and host biting mechanics. This research aligns with a theory within the field that <i>Ophiocordyceps</i> induce a change in ant behavior by secreting active metabolites that affect the host’s chemo-signaling pathway.<br> | ||
Another theory postulates that fungal-induced behavior changes are caused by disruption of host circadian clock genes, implementation of fungal synchronization genes, or both<ref name=" bdefense ">[https://academic.oup.com/icb/article/54/2/166/2797855 de Bekker C, Merrow M, Hughes DP. From Behavior to Mechanisms: An Integrative Approach to the Manipulation by a Parasitic Fungus (Ophiocordyceps unilateralis s.l.) of Its Host Ants (Camponotus spp.). Integrative and Comparative Biology. 2014 Jun 6;54(2):166–76.]</ref>. The different tasks and castes within a eusocial insect colony are governed by circadian clock genes that regulate where and what an insect is doing at any given time. By changing these genes, how they are coded, or even implementing fungal circadian clock genes would alter the inherent schedule of the host and may explain how and why ants abandon their nests and climb vegetation to latch onto (Fig. 6). | |||
<br><br> | |||
<br><br> | |||
==Conclusion== | |||
< | [[Image:Coculturing.jpg|thumb|500px|right|Figure 7. Results of co-culturing <I>C. militaris</i> with bacterial strains present in sclerotia. Slide A is cultured with <I>S. maltophilia</i>. Slide B is cultured with <I>P. baetica</i>. Slide C is control, only <I>C. militaris</i>.[https://pmc.ncbi.nlm.nih.gov/articles/PMC8627653/].]] | ||
< | Though the mechanism of infection by <i>Ophiocordyceps</i> is not fully understood, further research has implications for commercial production of antimicrobial compounds. Parasitic fungi and Hymenoptera host species have demonstrated abilities to produce antibacterial and antifungal compounds. As referenced before, Leaf-cutter ants house a symbiotic bacteria that produce and secrete an antifungal compound that destroys the mycoparasite, <i>Escovopsis</i>, that parasitizes the fungi within the fungal garden<ref name=" gdefense "></ref>. Despite being exposed to this mechanism of defense for millions of years, <i>Escovopsis</i> has not developed any sort of resistance to these compounds, unlike the increase of abundance of antibiotic-resistance in bacteria. Using insects as vectors for commercial antimicrobials such as antibiotics and antifungals, instead of bacteria, is a promising concept and may aid in production of such compounds. Entomopathogens also produce antimicrobial compounds, which, when cultured properly, can also be produced for commercial use. By co-culturing a fungus with a bacterial strain, the properties of that bacteria in the sclerotia of the fungus can affect the growth and metabolite production of the fungus<ref name=" cordybact "></ref> (Fig. 7). This allows for controlled mass production of commercial medical compounds. In addition, further research into the chemical basis of <i>Ophiocordyceps</i> fungal invasion of its host species may illuminate widespread methods of infection employed by entomopathogens on Hymenoptera as an order. | ||
<ref> | |||
< | |||
==References== | ==References== | ||
<references /> | <references /> | ||
<br>Edited by [ | <br>Edited by [Josie DeBruer], student of Joan Slonczewski for BIOL 116, 2024, [http://www.kenyon.edu/index.xml Kenyon College]. | ||
<!--Do not edit or remove this line-->[[Category:Pages edited by students of Joan Slonczewski at Kenyon College]] | <!--Do not edit or remove this line-->[[Category:Pages edited by students of Joan Slonczewski at Kenyon College]] |
Latest revision as of 16:55, 13 December 2024
Introduction
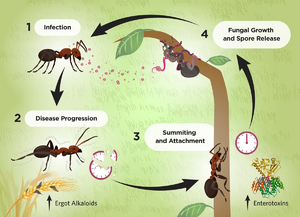
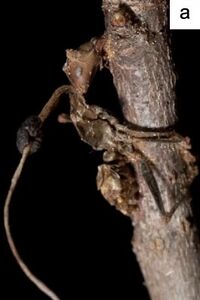
Parasitic ant-fungal relationships have been present since the Eocene epoch 45 million years ago[1]. These relationships, while similar in nature and spanning the order Hymenoptera, are the result of a coevolution between parasite and host resulting in highly specific mechanisms of action. The genus Ophiocordyceps falls into this category of entomopathogens, fungi that parasitize insects, and are commonly identified as the "Zombie-ant fungus". While each individual species of Ophiocordyceps infects a different host ant species, the method of fungal reproduction and spreading of spores remains the same (Fig. 1). Fungal spores penetrate the protected cuticle of the ant and begin to reproduce within internal ant tissues. After a certain period of time, usually after a few days, the infected ant will leave the colony, abandon its tasks, climb a vegetative structure, and bite onto the leaf, stem, or branch with its mandibles. Here, the ant will die, remaining latched on, as the fungus completes its life cycle by growing a fruiting body from the ant cadaver to disperse spores (Fig. 2).
Fungal Microbiome
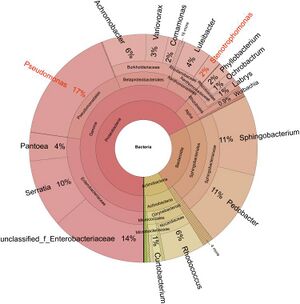
Parasitic fungi are often hosts to their "gut microbiome" in the form of a sclerotia. Many microorganisms can be identified in fungal sclerotia, a hardened fungal body that stores nutrients and other resources when the fungus encounters non-ideal survival conditions. A study conducted on Cordyceps militaris sclerotia, another ant-infecting entomopathogen closely related to Ophiocordyceps, measured bacteria and their function present[2]. In completing a transcriptomic analysis of 16S rRNA and creating a phylogenetic tree of bacteria present in fungal sclerotia, researchers identified two focal strains, Stenotrophomonas maltophilia and Pseudomonas baetica, among a wealth of others (Fig. 3). It was identified through co-culturing techniques that some bacteria were also parasitic to the insect hosts themselves or promoted fungal digestion through enzyme secretion, while others exhibited symbiosis with insect hosts as they function to inhibit fungal growth. In housing a microbiome that produces antimicrobial compounds, parasitic fungi are able to ensure an effective infection of their host while also monitoring their own growth and virulence in order to keep their host alive for proper development. It can be inferred that the genus Ophiocordyceps displays a similar microbiome in order to regulate infection of a host, due to the evolutionary proximity of the two genera and shared trait of entomopathogenicity.
Host Defenses
Ants belong to the order Hymenoptera along with several other parasitic or eusocial insects. Eusocial insects have evolved to live in populous colonies and complete certain tasks as a part of a caste system. The colonies these insects inhabit are prime breeding grounds for parasites because of the high population density and temperature within a nest[3].
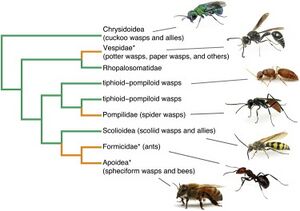
As a form of evolutionary defense, Hymenoptera have formed a symbiotic relationship with Actinobacteria that produce antimicrobial metabolites[4]. Worker ants are most exposed to entomopathogens because of the tasks they perform traveling into and out of the nest[3]. As a result, worker ants have evolved to secrete antimicrobial compounds from metapleural glands at the posterior of the thorax. These glands are present only among worker ants and not male ants that reside within the nest, which demonstrates the coevolution of entomopathogens and their hosts over time. Leaf-cutter ants exhibit another form of antimicrobial bacterial symbiosis. Leaf-cutter ants return to their nest with vegetation to add to the fungal garden within the nest (Fig. 5). Leaf-cutter ants cannot digest the leaves so they employ a symbiotic fungus which transforms the leaves into a glycogen-rich digestible compound. The mycoparasite, Escovopsis, poses a threat to these leaf-cutter ants as they parasitize the fungi that create this fungal garden. In another example of coevolution between parasites and hosts, leaf-cutter ants house a symbiotic bacteria that produces antifungal metabolites to combat Escovopsis.
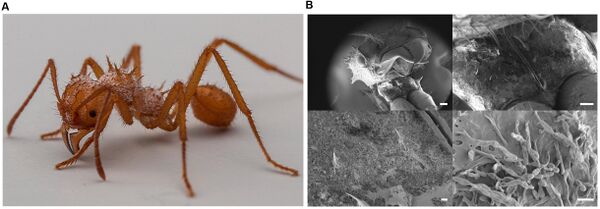
Hymenoptera have also developed behavioral and social defenses against entomopathogenic infection[3] (Fig. 4). Eusocial insects have adopted multimating, colony intermating, and kin avoidance behaviors in order to boost genetic diversity within colonies to increase the difficulty of entomopathogenic reproduction and infection. While these behaviors result in a net positive for the colony, multimating behaviors have shown to increase rates of sexually transmitted infection. These evolutionary advantages that increase genetic diversity are selected despite the negative implication of increased sexual transmission of pathogens.
It is possible the trait of eusociality evolved as functions to limit entomopathogenic infection in conjunction with the evolution of individual antimicrobial strength[3].
Genetic Basis and Possible Methods of Function
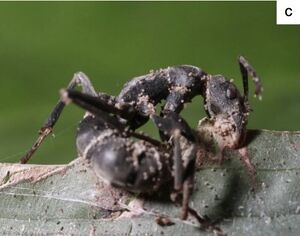
Once within a host, fungal spores secrete enterotoxins and active secondary metabolites that vary by fungal and host species. However, what chemically occurs and the cause is generally unknown during the fungal development, alteration of ant behavior, and attachment and fungal spore growth stages of infection. To further understand the methods of infection, researchers have performed comparative genomics tests on species of entomopathogens, pathogenic fungi, and non-pathogenic fungi in order to identify genes upregulated during biting events[5]. By also comparing the transcriptomes of Ophiocordyceps pre, post, and during infection, researchers identified a gene of interest that functions in the production of secondary metabolites. Results identified that, although entomopathogens alone secrete enterotoxins, 78% of the Ophiocordyceps genome was shared with non-pathogenic fungi and no novel genes were present in entomopathogens. Gene sequence between entomopathogens and species within Ophiocordyceps also varied greatly despite occupying and utilizing similar secondary metabolites and pathways. This suggests that entomopathogens have evolved to co-opt evolutionarily-present fungal genes for parasitization and host biting mechanics. This research aligns with a theory within the field that Ophiocordyceps induce a change in ant behavior by secreting active metabolites that affect the host’s chemo-signaling pathway.
Another theory postulates that fungal-induced behavior changes are caused by disruption of host circadian clock genes, implementation of fungal synchronization genes, or both[1]. The different tasks and castes within a eusocial insect colony are governed by circadian clock genes that regulate where and what an insect is doing at any given time. By changing these genes, how they are coded, or even implementing fungal circadian clock genes would alter the inherent schedule of the host and may explain how and why ants abandon their nests and climb vegetation to latch onto (Fig. 6).
Conclusion
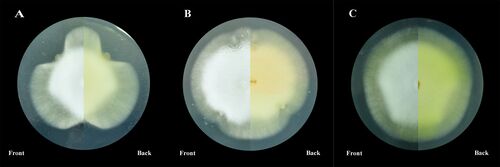
Though the mechanism of infection by Ophiocordyceps is not fully understood, further research has implications for commercial production of antimicrobial compounds. Parasitic fungi and Hymenoptera host species have demonstrated abilities to produce antibacterial and antifungal compounds. As referenced before, Leaf-cutter ants house a symbiotic bacteria that produce and secrete an antifungal compound that destroys the mycoparasite, Escovopsis, that parasitizes the fungi within the fungal garden[3]. Despite being exposed to this mechanism of defense for millions of years, Escovopsis has not developed any sort of resistance to these compounds, unlike the increase of abundance of antibiotic-resistance in bacteria. Using insects as vectors for commercial antimicrobials such as antibiotics and antifungals, instead of bacteria, is a promising concept and may aid in production of such compounds. Entomopathogens also produce antimicrobial compounds, which, when cultured properly, can also be produced for commercial use. By co-culturing a fungus with a bacterial strain, the properties of that bacteria in the sclerotia of the fungus can affect the growth and metabolite production of the fungus[2] (Fig. 7). This allows for controlled mass production of commercial medical compounds. In addition, further research into the chemical basis of Ophiocordyceps fungal invasion of its host species may illuminate widespread methods of infection employed by entomopathogens on Hymenoptera as an order.
References
- ↑ 1.0 1.1 de Bekker C, Merrow M, Hughes DP. From Behavior to Mechanisms: An Integrative Approach to the Manipulation by a Parasitic Fungus (Ophiocordyceps unilateralis s.l.) of Its Host Ants (Camponotus spp.). Integrative and Comparative Biology. 2014 Jun 6;54(2):166–76.
- ↑ 2.0 2.1 Luo L, Zhou J, Xu Z, Guan J, Gao Y, Zou X. Identification and functional analysis of bacteria in sclerotia of Cordyceps militaris. PeerJ. 2021 Nov 25;9:e12511.
- ↑ 3.0 3.1 3.2 3.3 3.4 Stowe A, Beattie A. Chemical and genetic defenses against disease in insect societies. ScienceDirect. 2008 Oct;22(7):1009–13.
- ↑ Matarrita-Carranza B, Moreira-Soto RD, Murillo-Cruz C, Mora M, Currie CR, Pinto-Tomas AA. Evidence for Widespread Associations between Neotropical Hymenopteran Insects and Actinobacteria. Frontiers in Microbiology. 2017 Oct 17;8.
- ↑ de Bekker C, Ohm RA, Evans HC, Brachmann A, Hughes DP. Ant-infecting Ophiocordyceps genomes reveal a high diversity of potential behavioral manipulation genes and a possible major role for enterotoxins. Scientific Reports. 2017 Oct 2;7(1):1–13.
Edited by [Josie DeBruer], student of Joan Slonczewski for BIOL 116, 2024, Kenyon College.