Evolution in Darkness: The Mexican Blind Cavefish: Difference between revisions
(40 intermediate revisions by the same user not shown) | |||
Line 1: | Line 1: | ||
== | ==Regressive Evolution== | ||
The transition from surface streams to the perpetual darkness of underwater caves has driven remarkable evolutionary changes in the Mexican blind cavefish (Astyanax mexicanus). Within the past few million years, populations migrating into caves abandoned their functional visual systems, a trait retained by their stream-dwelling counterparts. This dramatic adaptation is not unique to cavefish; troglobitic animals, including crustaceans, insects, salamanders, and spiders, have independently evolved similar traits, such as eye degeneration and heightened reliance on non-visual sensory systems. | The transition from surface streams to the perpetual darkness of underwater caves has driven remarkable evolutionary changes in the Mexican blind cavefish (Astyanax mexicanus). Within the past few million years, populations migrating into caves abandoned their functional visual systems, a trait retained by their stream-dwelling counterparts. This dramatic adaptation is not unique to cavefish; troglobitic animals, including crustaceans, insects, salamanders, and spiders, have independently evolved similar traits, such as eye degeneration and heightened reliance on non-visual sensory systems. | ||
[[Image:Mexican blind fish.jpg|thumb|300px|left|Figure 1. Mexican blind cavefish (Astyanax mexicanus)<ref name=" one ">[https://www.scienceabc.com/nature/animals/why-are-all-mexican-cavefish-blind.html Ketaki Bapat. Why Are All Mexican Cavefish Blind?.]</ref>]] | |||
Globally, over a hundred species of cave-dwelling fish exhibit varying degrees of blindness and other cave-specific adaptations, such as reduced pigmentation and enhanced mechanosensory abilities. The Mexican blind cavefish serves as a model organism for exploring how extreme habitats shape life through genetic, ecological, and microbiological influence. | |||
Biological evolution is often framed as a process of innovation, with emphasis placed on the development of new traits such as the legs of amphibians, the hair and mammary glands of mammals, or the large and complex brains of higher primates. However, this perspective overlooks an equally important evolutionary phenomenon: regressive evolution, or the loss of structures and traits that are no longer advantageous in a given environment. In many cases, evolutionary progress entails trade-offs. For a newly developed trait, an organism’s ancestors may have lost features that were no longer critical for survival. For instance, amphibians sacrificed the gills, scales, and tails that were essential to their aquatic ancestors, adapting instead to a terrestrial lifestyle. Blind cavefish exemplify regressive evolution through their loss of functional eyes and pigmentation. Living in absolute darkness, vision itself offers no survival advantage, while maintaining eyes and pigmentation would demand considerable metabolic energy. Natural selection, therefore, favors the loss of these structures. This evolutionary process illustrates that regressive changes are not failures of evolution but rather strategic responses to environmental pressures. | Biological evolution is often framed as a process of innovation, with emphasis placed on the development of new traits such as the legs of amphibians, the hair and mammary glands of mammals, or the large and complex brains of higher primates. However, this perspective overlooks an equally important evolutionary phenomenon: regressive evolution, or the loss of structures and traits that are no longer advantageous in a given environment. In many cases, evolutionary progress entails trade-offs. For a newly developed trait, an organism’s ancestors may have lost features that were no longer critical for survival. For instance, amphibians sacrificed the gills, scales, and tails that were essential to their aquatic ancestors, adapting instead to a terrestrial lifestyle. Blind cavefish exemplify regressive evolution through their loss of functional eyes and pigmentation. Living in absolute darkness, vision itself offers no survival advantage, while maintaining eyes and pigmentation would demand considerable metabolic energy. Natural selection, therefore, favors the loss of these structures. This evolutionary process illustrates that regressive changes are not failures of evolution but rather strategic responses to environmental pressures. | ||
== | ==The Role of Pleiotropy == | ||
Pleiotropy plays a significant role in the regressive evolution of eyes in cave-dwelling organisms, including mollies and Astyanax cavefish. Pleiotropy refers to a single gene influencing multiple traits, often resulting in evolutionary trade-offs. In cavefish, for example, genes associated with eye development also affect other traits critical for survival in the dark. One prominent gene implicated in this process is the Hedgehog (Hh) gene | Pleiotropy plays a significant role in the regressive evolution of eyes in cave-dwelling organisms, including mollies and Astyanax cavefish. Pleiotropy refers to a single gene influencing multiple traits, often resulting in evolutionary trade-offs. In cavefish, for example, genes associated with eye development also affect other traits critical for survival in the dark. One prominent gene implicated in this process is the Hedgehog (Hh) gene; increased Hedgehog activity leads to reduced eye size and, simultaneously, an increased density of taste buds. This adaptation boosts the fish’s ability to locate food in nutrient-scarce habitats. | ||
Gene mapping of Mexican Astyanax reveals that three distinct genes across separate chromosomes influence eye development. Notably, one of these genes is closely associated with a gene regulating metabolic rate. This genetic linkage suggests that mutations enhancing metabolism might simultaneously impair eye development, providing evidence that pleiotropy may drive the regressive evolution of cavefish eyes. | Gene mapping of Mexican Astyanax reveals that three distinct genes across separate chromosomes influence eye development. <ref name=" two ">Espinasa L, Espinasa M. Why Do Cave Fish Lose Their Eyes? Natural History. 2005;114(5):44-49. Accessed December 19, 2024. https://search-ebscohost-com.libproxy.kenyon.edu/login.aspx?direct=true&db=aph&AN=17153367&site=ehost-live]</ref> Notably, one of these genes is closely associated with a gene regulating metabolic rate. This genetic linkage suggests that mutations enhancing metabolism might simultaneously impair eye development, providing evidence that pleiotropy may drive the regressive evolution of cavefish eyes. | ||
[[Image: Olfactory pits.jpg|thumb|300px|right|Figure 2. Olfactory pits reformed in Mexican Blind Cave fish. <ref name=" two "></ref>]] | |||
Beyond eye development, pleiotropic effects extend to other morphological and sensory changes. For example, mutations that affect eye formation often enhance jaw morphology and olfactory structures. One striking adaptation is the expansion of the olfactory pit in blind cavefish, with a 13% increase in width. This broadening enlarges the surface area of the olfactory epithelium, significantly improving the fish’s sense of smell. Enhanced olfactory capabilities allow cavefish to detect chemical cues and locate food more efficiently. Moreover, the absence of eyes induces skeletal changes in the surrounding structures. The bones that would typically support the eye socket are repurposed and the broader nasal structures that result from these changes further enhance olfactory efficiency, illustrating how natural selection indirectly favors traits compensating for vision loss. | |||
==Genetic Basis of Vision Regression in Cave-Dwelling Mollies== | |||
We use Atlantic mollies who are also cave dwelling and similar to Astyanax to understand better the changes in gene expression as they have partially lost vision and hence provide more room for understanding the origin and basis of eye loss. | |||
The regression of visual structures in cave-dwelling Atlantic mollies is closely tied to changes in genetic expression, particularly in the opsin genes that regulate light sensitivity. <ref name=" three ">[https://pmc.ncbi.nlm.nih.gov/articles/PMC2817247/ Tobler M, Coleman SW, Perkins BD, Rosenthal GG. Reduced opsin gene expression in a cave-dwelling fish. Biol Lett. 2010 Feb 23;6(1):98-101. doi: 10.1098/rsbl.2009.0549. Epub 2009 Sep 9. PMID: 19740890; PMCID: PMC2817247.]</ref> These changes are evident in the reduced expression of LWS opsin in individuals from perpetually dark cave chambers, while SWS1 and Rh2 expression remain high in surface and front-cave populations. This reduction aligns with the rapid attenuation of long-wavelength light in low-light cave environments. Retinal immunohistochemistry revealed that the decrease in opsin expression is not accompanied by changes in the photoreceptor structure, as rod and cone densities remain consistent across populations. Instead, the decrease occurs at the transcriptional level, signifying a genetic mechanism underlying this adaptation. Laboratory studies further support this genetic basis, as fish from all three populations raised under identical lighting conditions displayed the same opsin expression patterns as their wild counterparts. This indicates that the observed differences in opsin gene expression are heritable and not merely a plastic response to the environment suggesting that genetic regulation is a key factor in the regression of vision in cave-dwelling mollies. <ref name=" four ">[https://www-sciencedirect-com.libproxy.kenyon.edu/science/article/pii/004269899090049Q Yokoyama R, Yokoyama S. Isolation, DNA sequence and evolution of a color visual pigment gene of the blind cave fish astyanax fasciatus. Vision research (Oxford). 1990;30(6):807-816. doi: 10.1016/0042-6989(90)90049-q.]</ref> | |||
The role of pleiotropy is also central to this process. Genes regulating opsin expression are closely linked to other traits beneficial for survival in darkness, such as enhanced taste bud density and altered jaw morphology. These pleiotropic effects highlight the interconnectedness of genetic pathways, where selection for advantageous traits like improved feeding efficiency in darkness may drive the regression of visual structures. This genetic interplay underscores how changes in gene expression contribute to the convergent evolution of troglodytic phenotypes in cave-dwelling organisms. | |||
==Microbiome== | |||
Cave-dwelling fish possess distinct microbiomes compared to their surface-dwelling relatives. These differences are likely a result of their unique habitats, which lack sunlight and have limited organic matter. For example, cavefish exhibit reduced diversity in their gut microbiota, which can affect nutrient processing and metabolic functions. The primary microbial taxa present in the cavefish microbiome include genera such as Bacteroides, Lactobacillus, and Flavobacterium, which are known for their roles in carbohydrate metabolism and fermentation. These adaptations allow cavefish to extract energy from the limited food sources available in their dark environments. | |||
The reduced availability of food in cave ecosystems has led to specific adaptations in cavefish microbiomes. The microbiota in these fish can facilitate the breakdown of complex carbohydrates, helping them to derive nutrients from otherwise indigestible sources. For instance, the symbiotic relationship between cavefish and their gut microbiota may enhance the fish's ability to digest detritus and other organic matter that accumulate in the cave environment. Such microbial contributions are crucial for the fish's survival, especially given their reduced ability to forage effectively in complete darkness. The microbiome also plays a critical role in the immune system of cave-dwelling fish. A balanced microbiome can protect against pathogens and contribute to the overall health of the host. Cavefish have been found to possess an adapted immune response, potentially influenced by their microbial communities. The absence of light and the stable, isolated environment of caves can reduce exposure to certain pathogens; however, the microbiome may still be crucial in defending against opportunistic infections. Understanding the dynamics between cavefish and their microbiomes can provide insights into how these fish maintain health in challenging environments. <ref name=" five ">[https://pubmed-ncbi-nlm-nih-gov.libproxy.kenyon.edu/30425894/ Ornelas-García P, Pajares S, Sosa-Jiménez VM, Rétaux S, Miranda-Gamboa RA. Microbiome differences between river-dwelling and cave-adapted populations of the fish Astyanax mexicanus (De Filippi, 1853). PeerJ. 2018 Nov 7;6:e5906. doi: 10.7717/peerj.5906. PMID: 30425894; PMCID: PMC6228550. ]</ref> | |||
==Neural Plasticity== | |||
[[Image: Eye process.jpg|thumb|300px|right|Figure 3. Eye Development in surface fish in comparison to eye loss in blind cave fish.<ref name=" six ">[http://doi.org/10.1098/rstb.2015.0487 Krishnan Jaya and Rohner Nicolas 2017Cavefish and the basis for eye lossPhil. Trans. R. Soc. B37220150487]</ref>]] | |||
= | Neural plasticity—the brain’s ability to reorganize itself by reallocating resources based on sensory input—is a well-documented phenomenon in many species. Blind cavefish, Astyanax mexicanus, exhibit a fascinating example of this adaptability as neurons that would typically be dedicated to visual processing are recruited to enhance tactile sensory networks, allowing them to navigate and detect objects in complete darkness. The neurons in the visual cortex of blind cavefish still respond to stimuli—though not visual in nature. Instead, these "visual" neurons become highly responsive to tactile input. This neural reallocation improves the fish’s ability to perceive its environment without relying on sight. By repurposing the visual cortex, cavefish create a more efficient neural network and the brain of the blind cavefish becomes optimized for its dark environment. <ref name=" two "></ref> | ||
<br><br> | |||
Hence, blind cavefish develop a more efficient neural network than eyed fish living in similar conditions. Without the need to process visual information, cavefish brains reallocate their neurons to more useful tasks, such as enhancing tactile perception. This process is akin to the neural plasticity seen in species like newborn kittens, where neurons in the visual cortex initially connect to both eyes. As development progresses, neurons become more specialized, with some being dedicated to processing information from one eye while others focus on the other. If one eye is removed or covered, neurons shift to the remaining functional eye, maximizing the efficiency of the brain’s sensory processing. In blind cavefish, a similar mechanism is likely at play. The absence of eyes not only reduces unnecessary brain activity but also accelerates the recruitment of neurons to support other sensory systems. | |||
==Conclusion== | ==Conclusion== | ||
The Mexican blind cavefish (Astyanax mexicanus) exemplifies the power of evolution to craft organisms perfectly suited to their environments, no matter how extreme. Living in the perpetual darkness of underwater caves, these fish have undergone regressive evolution, trading functional eyes and pigmentation for enhanced non-visual sensory systems that are finely tuned to the demands of their habitat. This evolutionary shift reallocates valuable metabolic resources, illustrating that survival in darkness is not about gaining complexity but refining functionality. Key genetic mechanisms, such as pleiotropy, demonstrate how a single genetic change can influence multiple traits, allowing adaptations like enhanced taste buds or olfactory sensitivity to arise alongside the loss of vision. Additionally, the microbiome plays a pivotal role, supporting nutrient absorption and immune function, critical in the resource-scarce cave environment. Together, these adaptations highlight the profound interplay between genetic, ecological, and microbial factors. They reveal not only how life thrives in the most unyielding conditions but also how evolution balances loss and innovation. | |||
==References== | ==References== | ||
<br>Edited by [ | <br>Edited by [Hibat Allah Gillani], student of Joan Slonczewski for BIOL 116, 2024, [http://www.kenyon.edu/index.xml Kenyon College]. | ||
<!--Do not edit or remove this line-->[[Category:Pages edited by students of Joan Slonczewski at Kenyon College]] | <!--Do not edit or remove this line-->[[Category:Pages edited by students of Joan Slonczewski at Kenyon College]] | ||
Latest revision as of 03:59, 19 December 2024
Regressive Evolution
The transition from surface streams to the perpetual darkness of underwater caves has driven remarkable evolutionary changes in the Mexican blind cavefish (Astyanax mexicanus). Within the past few million years, populations migrating into caves abandoned their functional visual systems, a trait retained by their stream-dwelling counterparts. This dramatic adaptation is not unique to cavefish; troglobitic animals, including crustaceans, insects, salamanders, and spiders, have independently evolved similar traits, such as eye degeneration and heightened reliance on non-visual sensory systems.
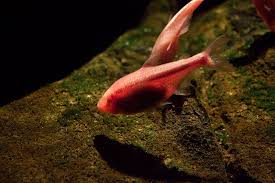
Globally, over a hundred species of cave-dwelling fish exhibit varying degrees of blindness and other cave-specific adaptations, such as reduced pigmentation and enhanced mechanosensory abilities. The Mexican blind cavefish serves as a model organism for exploring how extreme habitats shape life through genetic, ecological, and microbiological influence.
Biological evolution is often framed as a process of innovation, with emphasis placed on the development of new traits such as the legs of amphibians, the hair and mammary glands of mammals, or the large and complex brains of higher primates. However, this perspective overlooks an equally important evolutionary phenomenon: regressive evolution, or the loss of structures and traits that are no longer advantageous in a given environment. In many cases, evolutionary progress entails trade-offs. For a newly developed trait, an organism’s ancestors may have lost features that were no longer critical for survival. For instance, amphibians sacrificed the gills, scales, and tails that were essential to their aquatic ancestors, adapting instead to a terrestrial lifestyle. Blind cavefish exemplify regressive evolution through their loss of functional eyes and pigmentation. Living in absolute darkness, vision itself offers no survival advantage, while maintaining eyes and pigmentation would demand considerable metabolic energy. Natural selection, therefore, favors the loss of these structures. This evolutionary process illustrates that regressive changes are not failures of evolution but rather strategic responses to environmental pressures.
The Role of Pleiotropy
Pleiotropy plays a significant role in the regressive evolution of eyes in cave-dwelling organisms, including mollies and Astyanax cavefish. Pleiotropy refers to a single gene influencing multiple traits, often resulting in evolutionary trade-offs. In cavefish, for example, genes associated with eye development also affect other traits critical for survival in the dark. One prominent gene implicated in this process is the Hedgehog (Hh) gene; increased Hedgehog activity leads to reduced eye size and, simultaneously, an increased density of taste buds. This adaptation boosts the fish’s ability to locate food in nutrient-scarce habitats.
Gene mapping of Mexican Astyanax reveals that three distinct genes across separate chromosomes influence eye development. [2] Notably, one of these genes is closely associated with a gene regulating metabolic rate. This genetic linkage suggests that mutations enhancing metabolism might simultaneously impair eye development, providing evidence that pleiotropy may drive the regressive evolution of cavefish eyes.
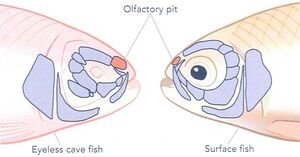
Beyond eye development, pleiotropic effects extend to other morphological and sensory changes. For example, mutations that affect eye formation often enhance jaw morphology and olfactory structures. One striking adaptation is the expansion of the olfactory pit in blind cavefish, with a 13% increase in width. This broadening enlarges the surface area of the olfactory epithelium, significantly improving the fish’s sense of smell. Enhanced olfactory capabilities allow cavefish to detect chemical cues and locate food more efficiently. Moreover, the absence of eyes induces skeletal changes in the surrounding structures. The bones that would typically support the eye socket are repurposed and the broader nasal structures that result from these changes further enhance olfactory efficiency, illustrating how natural selection indirectly favors traits compensating for vision loss.
Genetic Basis of Vision Regression in Cave-Dwelling Mollies
We use Atlantic mollies who are also cave dwelling and similar to Astyanax to understand better the changes in gene expression as they have partially lost vision and hence provide more room for understanding the origin and basis of eye loss.
The regression of visual structures in cave-dwelling Atlantic mollies is closely tied to changes in genetic expression, particularly in the opsin genes that regulate light sensitivity. [3] These changes are evident in the reduced expression of LWS opsin in individuals from perpetually dark cave chambers, while SWS1 and Rh2 expression remain high in surface and front-cave populations. This reduction aligns with the rapid attenuation of long-wavelength light in low-light cave environments. Retinal immunohistochemistry revealed that the decrease in opsin expression is not accompanied by changes in the photoreceptor structure, as rod and cone densities remain consistent across populations. Instead, the decrease occurs at the transcriptional level, signifying a genetic mechanism underlying this adaptation. Laboratory studies further support this genetic basis, as fish from all three populations raised under identical lighting conditions displayed the same opsin expression patterns as their wild counterparts. This indicates that the observed differences in opsin gene expression are heritable and not merely a plastic response to the environment suggesting that genetic regulation is a key factor in the regression of vision in cave-dwelling mollies. [4]
The role of pleiotropy is also central to this process. Genes regulating opsin expression are closely linked to other traits beneficial for survival in darkness, such as enhanced taste bud density and altered jaw morphology. These pleiotropic effects highlight the interconnectedness of genetic pathways, where selection for advantageous traits like improved feeding efficiency in darkness may drive the regression of visual structures. This genetic interplay underscores how changes in gene expression contribute to the convergent evolution of troglodytic phenotypes in cave-dwelling organisms.
Microbiome
Cave-dwelling fish possess distinct microbiomes compared to their surface-dwelling relatives. These differences are likely a result of their unique habitats, which lack sunlight and have limited organic matter. For example, cavefish exhibit reduced diversity in their gut microbiota, which can affect nutrient processing and metabolic functions. The primary microbial taxa present in the cavefish microbiome include genera such as Bacteroides, Lactobacillus, and Flavobacterium, which are known for their roles in carbohydrate metabolism and fermentation. These adaptations allow cavefish to extract energy from the limited food sources available in their dark environments.
The reduced availability of food in cave ecosystems has led to specific adaptations in cavefish microbiomes. The microbiota in these fish can facilitate the breakdown of complex carbohydrates, helping them to derive nutrients from otherwise indigestible sources. For instance, the symbiotic relationship between cavefish and their gut microbiota may enhance the fish's ability to digest detritus and other organic matter that accumulate in the cave environment. Such microbial contributions are crucial for the fish's survival, especially given their reduced ability to forage effectively in complete darkness. The microbiome also plays a critical role in the immune system of cave-dwelling fish. A balanced microbiome can protect against pathogens and contribute to the overall health of the host. Cavefish have been found to possess an adapted immune response, potentially influenced by their microbial communities. The absence of light and the stable, isolated environment of caves can reduce exposure to certain pathogens; however, the microbiome may still be crucial in defending against opportunistic infections. Understanding the dynamics between cavefish and their microbiomes can provide insights into how these fish maintain health in challenging environments. [5]
Neural Plasticity
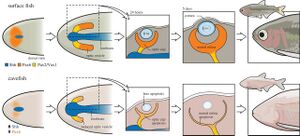
Neural plasticity—the brain’s ability to reorganize itself by reallocating resources based on sensory input—is a well-documented phenomenon in many species. Blind cavefish, Astyanax mexicanus, exhibit a fascinating example of this adaptability as neurons that would typically be dedicated to visual processing are recruited to enhance tactile sensory networks, allowing them to navigate and detect objects in complete darkness. The neurons in the visual cortex of blind cavefish still respond to stimuli—though not visual in nature. Instead, these "visual" neurons become highly responsive to tactile input. This neural reallocation improves the fish’s ability to perceive its environment without relying on sight. By repurposing the visual cortex, cavefish create a more efficient neural network and the brain of the blind cavefish becomes optimized for its dark environment. [2]
Hence, blind cavefish develop a more efficient neural network than eyed fish living in similar conditions. Without the need to process visual information, cavefish brains reallocate their neurons to more useful tasks, such as enhancing tactile perception. This process is akin to the neural plasticity seen in species like newborn kittens, where neurons in the visual cortex initially connect to both eyes. As development progresses, neurons become more specialized, with some being dedicated to processing information from one eye while others focus on the other. If one eye is removed or covered, neurons shift to the remaining functional eye, maximizing the efficiency of the brain’s sensory processing. In blind cavefish, a similar mechanism is likely at play. The absence of eyes not only reduces unnecessary brain activity but also accelerates the recruitment of neurons to support other sensory systems.
Conclusion
The Mexican blind cavefish (Astyanax mexicanus) exemplifies the power of evolution to craft organisms perfectly suited to their environments, no matter how extreme. Living in the perpetual darkness of underwater caves, these fish have undergone regressive evolution, trading functional eyes and pigmentation for enhanced non-visual sensory systems that are finely tuned to the demands of their habitat. This evolutionary shift reallocates valuable metabolic resources, illustrating that survival in darkness is not about gaining complexity but refining functionality. Key genetic mechanisms, such as pleiotropy, demonstrate how a single genetic change can influence multiple traits, allowing adaptations like enhanced taste buds or olfactory sensitivity to arise alongside the loss of vision. Additionally, the microbiome plays a pivotal role, supporting nutrient absorption and immune function, critical in the resource-scarce cave environment. Together, these adaptations highlight the profound interplay between genetic, ecological, and microbial factors. They reveal not only how life thrives in the most unyielding conditions but also how evolution balances loss and innovation.
References
Edited by [Hibat Allah Gillani], student of Joan Slonczewski for BIOL 116, 2024, Kenyon College.
- ↑ Ketaki Bapat. Why Are All Mexican Cavefish Blind?.
- ↑ Jump up to: 2.0 2.1 2.2 Espinasa L, Espinasa M. Why Do Cave Fish Lose Their Eyes? Natural History. 2005;114(5):44-49. Accessed December 19, 2024. https://search-ebscohost-com.libproxy.kenyon.edu/login.aspx?direct=true&db=aph&AN=17153367&site=ehost-live]
- ↑ Tobler M, Coleman SW, Perkins BD, Rosenthal GG. Reduced opsin gene expression in a cave-dwelling fish. Biol Lett. 2010 Feb 23;6(1):98-101. doi: 10.1098/rsbl.2009.0549. Epub 2009 Sep 9. PMID: 19740890; PMCID: PMC2817247.
- ↑ Yokoyama R, Yokoyama S. Isolation, DNA sequence and evolution of a color visual pigment gene of the blind cave fish astyanax fasciatus. Vision research (Oxford). 1990;30(6):807-816. doi: 10.1016/0042-6989(90)90049-q.
- ↑ Ornelas-García P, Pajares S, Sosa-Jiménez VM, Rétaux S, Miranda-Gamboa RA. Microbiome differences between river-dwelling and cave-adapted populations of the fish Astyanax mexicanus (De Filippi, 1853). PeerJ. 2018 Nov 7;6:e5906. doi: 10.7717/peerj.5906. PMID: 30425894; PMCID: PMC6228550.
- ↑ Krishnan Jaya and Rohner Nicolas 2017Cavefish and the basis for eye lossPhil. Trans. R. Soc. B37220150487