Snottites: Difference between revisions
Line 13: | Line 13: | ||
==Role in Cave Formation and Environmental Conditions== | ==Role in Cave Formation and Environmental Conditions== | ||
<br>Palmer and Palmer (1998, [http://www.caves.org/pub/journal/PDF/V60/V60N3-Abstracts.pdf 9]) propose three different mechanisms for cave enlargement (Figure 3). First, as the sulphuric acid converts the limestone to gypsum crust, the cave streams wash the crust away. Second, the sulfuric acid from the snottites and gypsum crystals drips and corrodes the limestone below; the microbes that form the snottites produce this acid through oxidation reactions. Lastly, solutional rills are created as the sulfuric acid leaks through cracks in the gypsum crust, dissolving the limestone base below. These three processes are not mutually exclusive and may all occur inside the caves at the same time. The sulfuric acid necessary for these processes is produced when hydrogen sulfide is oxidized (Equation 1). This oxidation can occur through abiotic or microbial processes. Gypsum and carbonic acid are formed upon the reaction of sulfuric acid with the carbonate from the dissolving host limestone (Equation 2).<br> | <br>Palmer and Palmer (1998, [http://www.caves.org/pub/journal/PDF/V60/V60N3-Abstracts.pdf 9]) propose three different mechanisms for cave enlargement (Figure 3). [[Image:Prr-1.gif|thumb|300px|right| [http://www.caves.org/pub/journal/PDF/V61/v61n1-Hose.pdf Figure 3.] Mechanisms for cave enlargement. (1) Conversion of limestone to gypsum crust by sulphuric acid, dissolved by the cave stream and by infiltrating freshwater. (2) Drips of sulphuric acid from the gypsum wall crust and snottites corrode limestone below. Microbial activity enhances the oxidation reactions that produce the acid. (3) Sulphuric acid seeps through the gypsum crust and dissolves the limestone at the base. Solutional rills are common. Found in Hose et al., 2000 (after Palmer and Palmer, 1998). ]]First, as the sulphuric acid converts the limestone to gypsum crust, the cave streams wash the crust away. Second, the sulfuric acid from the snottites and gypsum crystals drips and corrodes the limestone below; the microbes that form the snottites produce this acid through oxidation reactions. Lastly, solutional rills are created as the sulfuric acid leaks through cracks in the gypsum crust, dissolving the limestone base below. These three processes are not mutually exclusive and may all occur inside the caves at the same time. The sulfuric acid necessary for these processes is produced when hydrogen sulfide is oxidized (Equation 1). This oxidation can occur through abiotic or microbial processes. Gypsum and carbonic acid are formed upon the reaction of sulfuric acid with the carbonate from the dissolving host limestone (Equation 2).<br> | ||
<br>H<sub>2</sub>S + 2O<sub>2</sub> → H<sub>2</sub>SO<sub>4</sub> (1)<br> | <br>H<sub>2</sub>S + 2O<sub>2</sub> → H<sub>2</sub>SO<sub>4</sub> (1)<br> | ||
<br>CaCO<sub>3</sub> + H<sub>2</sub>SO<sub>4</sub> → CaSO<sub>4</sub> + H<sub>2</sub>CO<sub>3</sub> (2)<br> | <br>CaCO<sub>3</sub> + H<sub>2</sub>SO<sub>4</sub> → CaSO<sub>4</sub> + H<sub>2</sub>CO<sub>3</sub> (2)<br> | ||
<br>The chemolithotrophic microorganisms are active in the oxidation process of sulfide and the reduced sulfur compounds ultimately act as their energy source. | <br>The chemolithotrophic microorganisms are active in the oxidation process of sulfide and the reduced sulfur compounds ultimately act as their energy source. The cave system relies solely on the energy produced within, as there is no organic input from the outside environment.<br> | ||
<br>These microbes manifest themselves in biofilms along every surface of the caves. The classical limestone caves have carbonate speleothems, such as stalactites and stalagmites, which form as water runs into the cave and carbon dioxide degasses into the cave air. Primary minerals in the cave undergo a physicochemical reaction to form secondary mineral deposits, which in turn form speleothems. These sulfide caves have their own types of special formations. Vermiculations are often described as “discontinuous, worm-like deposits of mud and clay found on cave walls and ceilings” [http://www.caves.org/pub/journal/PDF/v70/cave-70-02-78.pdf 4], but in the microbial context they are referred to as biovermiculations. Biovermiculations are very diverse and rapidly forming arrangements of microbes. The most intensely studied microbial cave formations are stream biofilms; this thick white layer of microbes coats the entire surface of the standing water in caves. Research suggests that these biofilms are crucial components of sulfur cycling and of general cave biogeochemistry [http://aem.asm.org/cgi/content/abstract/72/8/5596 5].<br> | <br>These microbes manifest themselves in biofilms along every surface of the caves. The classical limestone caves have carbonate speleothems, such as stalactites and stalagmites, which form as water runs into the cave and carbon dioxide degasses into the cave air. Primary minerals in the cave undergo a physicochemical reaction to form secondary mineral deposits, which in turn form speleothems. These sulfide caves have their own types of special formations. Vermiculations are often described as “discontinuous, worm-like deposits of mud and clay found on cave walls and ceilings” [http://www.caves.org/pub/journal/PDF/v70/cave-70-02-78.pdf 4], but in the microbial context they are referred to as biovermiculations. Biovermiculations are very diverse and rapidly forming arrangements of microbes. The most intensely studied microbial cave formations are stream biofilms; this thick white layer of microbes coats the entire surface of the standing water in caves. Research suggests that these biofilms are crucial components of sulfur cycling and of general cave biogeochemistry [http://aem.asm.org/cgi/content/abstract/72/8/5596 5].<br> | ||
<br> | <br> |
Revision as of 16:43, 1 May 2009
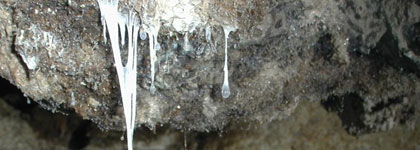
Introduction
Caves are human-accessible, underground natural spaces that extend beyond areas where sunlight can reach 8. These caverns are commonly formed within limestone or other calcareous rocks, but can emerge in basaltic rock, gypsum, granite, talus, quartzite, ice, and sandstone. They can be developed in one of three ways: classical limestone formation, lava tube formation, and sulfuric-acid driven speleogenesis. In classical limestone caves, water absorbs CO2 as it runs through the soil zone and forms a dilute solution of carbonic acid. Limestone is exposed to this water at the water table, where the calcium carbonate from the bedrock is dissolved, forming caverns. Lava tube formation, on the other hand, involves lava flow from an active volcano. The lava on the surface cools quickly, creating a solid encasement. Once the lava below drains and the eruption ceases, an empty cavern is left behind. Finally, in sulfuric-acid driven speleogenesis, hydrogen sulfide rises in crevices in the earth until it reaches an oxygenated zone. Sulfuric acid then dissolves the limestone. The extreme conditions of these sulfide-rich cave systems may reflect the early Earth environments, especially those that developed in the early Proterozoic era upon the initial rise of oxygen 4 and 5. These sulfide-rich caves are comparable to sulfur hot springs and the black smokers of deep-sea vents.
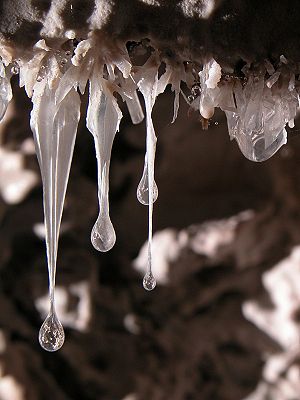
Crystals, sulfur mineral deposits, and a variety of microbial biofilms can be found in these latter, sulfide-rich cave systems. These formations include moonmilk, microbial mats in cave streams, biovermiculations, stream sediments, wall crusts and films, and snottites. Snottites have captivated cave-goers and scientists alike since the earliest publication on cave microbes by Hoeg in 1946 8. These biofilms cover the walls with a thick snot-like film, from which they derive their particularly appropriate name. A variety of cave systems, the Frasassi caves in Italy, the Cueva de Villa Luz in Tabasco, Mexico, Grotta di Rio Garrago, and Cueva Luna Azufre, are sites of intense research for these microbial populations, but these are not the only caves with these formations. The snottites are exceptionally acidic, with pH 0-2. The composition of these snottites is still unknown, they have been found include to Sulfobacillus, Aciditiobacillus, Halothiobacillus, and Acidimicrobium species, protists, filamentous fungi, and a proposed bacterial lineage TM6.
Scientists traditionally originally believed that most sulfide-rich limestone caves were formed by carbonic acid dissolution of carbonate, but recent studies now support the theory that these bacterial species have a large impact on the formation of limestone bedrock caves in the presence of sulfide-rich waters. Sulfur acid-driven speleogenesis, driven by circulation of sulfidic waters through limestone, is the common mechanism proposed by many studies. Originally this process was thought to occur through nonbiological processes, but this belief has since been modified by the discovery of the impact of bacteria, specifically Thiobacillus species, in these environments. Theses sulfur-oxidizing bacteria are now recognized as essential components in the disintegration of limestone and in the creation and expansion of caves.
These microorganisms also serve a function in the ecosystems of caves. Caves are fairly mild and predictable environments, but contain very limited resources due to the lack of sunlight necessary to support primary production of organic materials. Caves have three zones: the “entrance”, “twilight”, and “deep cave” zones. In the entrance zone, the outside environment has a large impact on the conditions. At the increased depth of the twilight zone cave conditions begin to overpower outside conditions and light becomes extremely limited. At the deep cave level, no light can penetrate, the humidity is high, and the temperature reaches MAST (Mean Annual Surface Temperature) for the region 8. At this cave depth, no phototrophic material can grow, so organic material must be produced by some other means. Microorganisms are prime candidates for this role.
Role in Cave Formation and Environmental Conditions
Palmer and Palmer (1998, 9) propose three different mechanisms for cave enlargement (Figure 3).
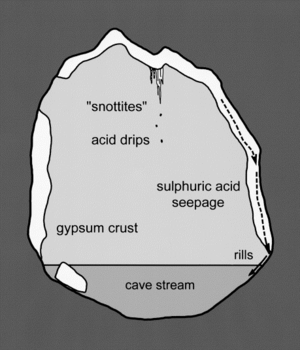
First, as the sulphuric acid converts the limestone to gypsum crust, the cave streams wash the crust away. Second, the sulfuric acid from the snottites and gypsum crystals drips and corrodes the limestone below; the microbes that form the snottites produce this acid through oxidation reactions. Lastly, solutional rills are created as the sulfuric acid leaks through cracks in the gypsum crust, dissolving the limestone base below. These three processes are not mutually exclusive and may all occur inside the caves at the same time. The sulfuric acid necessary for these processes is produced when hydrogen sulfide is oxidized (Equation 1). This oxidation can occur through abiotic or microbial processes. Gypsum and carbonic acid are formed upon the reaction of sulfuric acid with the carbonate from the dissolving host limestone (Equation 2).
H2S + 2O2 → H2SO4 (1)
CaCO3 + H2SO4 → CaSO4 + H2CO3 (2)
The chemolithotrophic microorganisms are active in the oxidation process of sulfide and the reduced sulfur compounds ultimately act as their energy source. The cave system relies solely on the energy produced within, as there is no organic input from the outside environment.
These microbes manifest themselves in biofilms along every surface of the caves. The classical limestone caves have carbonate speleothems, such as stalactites and stalagmites, which form as water runs into the cave and carbon dioxide degasses into the cave air. Primary minerals in the cave undergo a physicochemical reaction to form secondary mineral deposits, which in turn form speleothems. These sulfide caves have their own types of special formations. Vermiculations are often described as “discontinuous, worm-like deposits of mud and clay found on cave walls and ceilings” 4, but in the microbial context they are referred to as biovermiculations. Biovermiculations are very diverse and rapidly forming arrangements of microbes. The most intensely studied microbial cave formations are stream biofilms; this thick white layer of microbes coats the entire surface of the standing water in caves. Research suggests that these biofilms are crucial components of sulfur cycling and of general cave biogeochemistry 5.
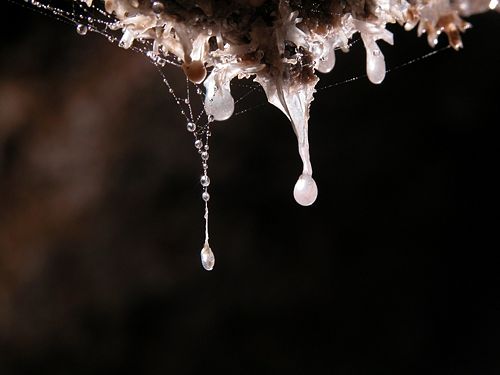
Snottites form as an extension of the microbial biofilms that coat the walls and ceilings of caves, and form around elemental sulfur deposits on the surface of sulfate crusts. These sulphate crusts, specifically gypsum (CaSO4 · 2H2O) and barite (BaSO4), are manifested in thick crusts of white, fine-grained crystals covering the walls. The biofilms accumulate on the sulfur deposits in thick layers of viscous liquid. The snottites form as gravity pulls down portions of the biofilm into mucoid drips 10. The extremely low acidity levels (between 0 and 3) in these drops have been attributed to Thiobacillus thiooxidans, which produce local environmental pH levels of less than 2 under low oxygen conditions. When oxygen is present, the oxidation is stimulated, causing the pH level to drop below 1 3. These drops not only accumulate on the snottites and gypsum crystals, but also on the plethora of spider webs that cover the cave walls (Figure 4). These acidic drips have been known to burn through clothes and cause third-degree burns on the skin of cave-goers.
In addition to these microbial communities, structures form as a result of their oxidation processes. Gypsum (CaSO4⋅6H2O) and sulfur accumulate throughout the cave in various forms. Below the water table, the gypsum is washed away, but above the water table, the gypsum accumulates in crusts on the surfaces of caves as the hydrogen sulfide degasses into condensation droplets. The gypsum coats walls, ceilings, and floors in a paste often up to depths of several meters 3. The crusts of these microcrystalline deposits are often several centimeters thick. Large blades of recrystallized gypsum, sometimes extending 10cm, jut out from ceilings and overhanging walls. Sulfur also collects on the walls on top of the gypsum where it cannot reach the limestone, or on sections of bedrock that contain insoluble materials. Usually the carbonate rocks neutralize the elemental sulfur, but on these surfaces the sulfur can accumulate and remain stable at low pH. Studies have been conducted on the composition of these gypsum and sulfur deposits to identify the origin for sulfur in caves; high percentages of the light isotope of sulfur (34S) indicate biogenic origins. Studies have found that the native sulfur and gypsum deposits in sulfide-rich caves have high enough concentrations of 34S to indicate biogenic origins, but hydrogen sulfide does not 8. These findings correlate well with the processes of cave formation and sulfide oxidation.
The drips off of the snottites are not the only aspect of the cave environment affected by the readily available sulfur. Figure 4 shows some characteristics of the area around some snottite sample sites.
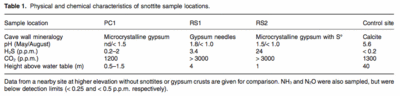
The air quality throughout the cave varies greatly due to the varying concentrations of H2S. In 1987, the American Conference of Governmental Industrial Hygienists established a threshold limit value (TLV) for H2S at10 PPM. In the late 1990s, the Environmental Protection agency tightened these parameters to a zeta tolerance limit. Near the inlets of hydrogen sulfide, outgassing of the H2S creates atmospheric levels as high as 210 ppm, creating significant health hazards for visitors to the caves. Outgassing occurs in rapid pulses, causing extreme fluctuations of atmospheric H2S concentrations in mere minutes. 2. Carbon monoxide levels can run dangerously high and oxygen levels run dangerously low (9.6% in some areas). The conditions have become so bad that teams of scientists have been forced to evacuate during data collection 2. The conditions are much less radical away from the water inlets and cave openings to the outside environments.
The slightly thermal, sulfur-rich water that runs through these caves typically registers pH values between 6.6 and 7.4, depending upon the season. The water throughout the cave is often described as milky, and 2-3cm long white filaments float along with the current. The color is suspected to be from suspended elemental sulfur and prolonged exposure of this water to the skin causes mild burning sensations 2.
Species identified

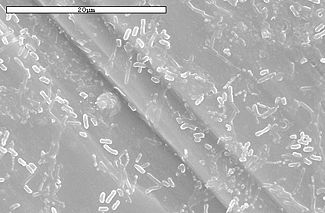

Based on the ecological findings in the caves, certain species of bacteria would be expected to comprise the variety of biofilms formed in these sulfide-rich cave systems. One would expect to find chemilithotrophic (“rock eating”) and acidophilic (“acid-loving”) species. The genus Thiobacillus contains a number of sulfur-oxidizing species with these characteristics. The autotrophic species Thiobacillus thiooxidans is perhaps the most thoroughly studied. These bacteria have the ability, in the absence of any organic carbon source, to fix carbon dioxide into biomass.
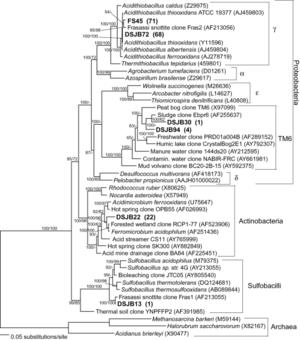
Its strongly exothermic process of producing metabolically useful energy from the oxidation of sulfur and sulfuric acid provides large amounts of energy to the living organisms around it. These are the microorganisms that may be responsible for the high pH value of the snottites. Another thoroughly studied species, Thiobacillus ferrooxidans, was originally identified in acid drainage sources and can produce copious sulfuric acid.
Sulfate-reducing bacteria (SRB) are responsible for a process called “disproportionation” or “sulfido-genesis.” These organisms transform elemental sulfur into sulfate or sulfide. They can use inorganic materials for chemoautotrophic disproportionation or organic materials for chemoorganotrophic disproportionation. Desulfolobus propionicus is a common SRB species.
Species in the genera Sulfolobus and Acidianus are also expected to appear in environments like these cave systems. These extreme acidophilic archaeobacteria are sulfur respirers. Sulfolobus species are exclusively aerobic autotrophs that oxidize elemental sulfur to sulfuric acid. The Acidianus species can utilize sulfur as either an electron acceptor or electron donor, depending upon the conditions. Under anaerobic conditions the organisms use elemental sulfur as an electron donor to oxidize H2. Under aerobic conditions, they use sulfur as an electron acceptor to oxidize S° to sulfuric acid. The various abilities of these microbial species serve the function of cycling sulfur in the cave systems discussed above.
A sampling of pink-colored, hydrophobic snottites revealed dense communities of microorganisms in banded growth rings. These rings indicate different organism types with individual oxygen preferences 3. The concentration of H2S in samples ranges between 0.2 and 24 ppm. More specific analyses of snottite samples revealed not only bacterial organisms, but archaea as well. In a study performed in the Frasassi cave system, Macalady, Jones, and Lyon (2007, 6) found a variety of species in the snottite samples. They created bacterial 16S rDNA clone libraries for the samples (Figures 8 and 10). The most common phylotypes found were of the Acidithiobacillus species, related closely to A. thiooxidans and A. albertensis, A. ferroxidans, and A. caldus. More uncommon phylotypes found were related to the Sulfobacillus species. Additionally, close relatives were found in the proposed TM6 lineage and archaea. The archaea found were identified as archaeal genera Ferroplasma and Thermoplasma. F. acidiphilum was the most abundant type found.
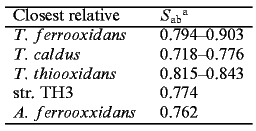
The samples collected were connected to clones from sewage sludge, marine mud volcanoes, peat bogs, groundwater, acid mine and acid rock drainage, bioleaching environments, and thermal sulfidic springs and vents. These samples were not incredibly diverse, but definitely rich with bacterial cells. Hose, et al (2000) 3 performed extensive analysis on snottite composition in Cueva de Villa Luz. They performed DNA extraction, aplification, and cloning to create bacterial clone libraries to combine with SEM studies (Figures 5, 6, and 7) and plate cultures to find the composition of the snottite formations. The table in Figure 9 shows their results from the Ribosomal Database Project. The data shows how closely related the bacterial clones from the snottites are to species in the database.
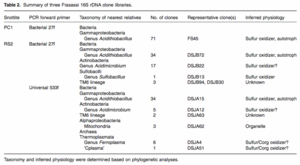
In addition to bacterial clone libraries, microbial morphologies were observed to identify bacterial types present. A variety of probes were used and three morphologies were found: short rods, thin vibrios, and thicker ovoid cells. Additionally, two archaeal morphologies were found: large, irregularly shaped cells, and coccoid cells of varying sizes. Weakly autoflourescing fungal filaments and large ovoid cells with nuclei, presumed to be protists, were identified as well.
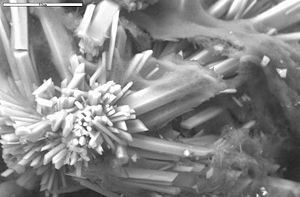
Ecosystem Characteristics
The environmental impact of these microbial species in snottites appears to spread further from just cave formation and atmospheric conditions. In such harsh conditions, these microorganisms become the primary producers of energy for the other life in the cave. Midges, spiders, and gastropods are just a few of the organisms found roaming the caves and eating the microbial colonies. A specific species of fish, Poecilia mexicana, have also been found in the Cueva de Villa Luna caves. These fish gather in densely populated groups in the streams of the caves. Obviously all of these organisms are adapted to the sulfide-rich cave environment, but all organisms must have an energy source to survive on. These snottites and microbial populations fill that need. Unfortunately, not all of the life found in the caves is well-adapted. Bats have often been found as regular residents, but large numbers of deceased and dying bats can often be found on the walls, ceilings, and floors of the caves. These individuals were undoubtedly caught in one of the degassing events described above.
Conclusion
The intense investigation of caves as microbial habitats is a fairly recent event. While the biofilms they create have always been evident and enthralling, their exact composition was, until recently, generally unknown. The dangerous atmospheric conditions don’t make these environments ideal for long term exposure, but safety measures could enable intense and rewarding data. These microbial species are obviously vital to the sulfur cycling and cave formation processes. Fortunately, there are a variety of caves that exhibit the expansion process in various stages, enabling scientists to see the long term effects.
These cave systems, while harsh in their conditions, are relatively constant compared to other environments since the deeper parts are considerably isolated from the outside world. Northup and Lavoie (2001) 8 state, “Caves should be used as experimental study systems for geomicrobiology, not because they are strange, but because they are simple and often locally abundant, allowing for replicate studies.” Scientists have already used caves as incubators to grow certain species found in these environments so as to run replicate tests on the cultures. Further investigations are necessary to identify the exact species involved in the formations as well as the extent to which the microorganisms participate in cave expansion.
References
1Galdenzi, S et al. “Sulfidic Ground-Water Chemistry in the Frasassi Caves, Italy”. Journal of Cave and Karst Studies. 2008. Volume 70(2). p. 94-107.
2Hose, L. D., and Pisarowicz, J. A. “Cueva de Villa Luz, Tabasco, Mexico: Reconnaissance study of an active sulfur spring cave and ecosystem”. Journal of Cave and Karst Studies. 1999. Volume 61(1). p. 13-21.
3Hose, L. D., Palmer, A. N., Palmer, M. V., Northup, D. E., Boston, P. J., and DuChene, H. R. "Microbiology and geochemistry in a hydrogen-sulfide-rich karst environment". Chemical Geology. 2000. Volume 169. p. 399-423.
4Jones, D, Lyon E., and Macalady, J. “Geomicrobiology of Biovermiculations from the Frasassi Cave System, Italy”. Journal of Cave and Karst Studies. 2008. Volume 70(2). p. 76-93.
5Macalady, J. et al. “Dominant Microbial Populations in Limestone-Corroding Stream Biofilms, Frasassi Cave System, Italy”. Applied and Environmental Microbiology. 2006. Volume 72(8). p. 5596-5609.
6Macalady, J. L., D. S. Jones, and E. H. Lyon. “Extremely acidic, pendulous cave wall biofilms from the Frasassi cave system, Italy”. Environmental Microbiology. 2007. Volume 9(6). p. 1402-1414.
7Mulec, Janez. “Microorganisms in hypogean: examples from Slovenian karst caves”. Acta Carologica. 2008. Volume 37(1). p. 153-160.
8Northup, D. E., and K. H. Lavoie. “Geomicrobiology of caves: a review”. Geomicrobiology Journal. 2001. Volume 18. p. 199-222.
9Palmer, A.N., Palmer, M.V. "Geochemistry of Cueva de Villa Luz, Mexico: an active H2S cave". Journal of Cave and Karst Studies. 1998. Volume 60(3). p. 188
10Vlasceanu, L, S. M. Sarbu, A. S. Engel, and B. K. Kinkle. “Acidic cave-wall biofilms located in the Frasassi Gorge, Italy”. Geomicrobiology Journal. 2000. Volume 17. p. 125-139.
Edited by Paige Roberts, student of Joan Slonczewski for BIOL 238 Microbiology, 2009, Kenyon College.