Shigella dysenteriae: Difference between revisions
Line 10: | Line 10: | ||
==Description and significance== | ==Description and significance== | ||
Physical characteristics: ''S. dysenteriae'' is a small, uncapsulated, non-motile, Gram-negative, nonsporulating bacillus (rod-shaped). | Physical characteristics: ''S. dysenteriae'' is a small, uncapsulated, non-motile, Gram-negative, nonsporulating bacillus (rod-shaped). | ||
[[Image:Pic_1.jpg|frame|none|Shigella dysenteriae Image copyright Dennis Kunkel Microscopy, Inc http://denniskunkel.com]] | [[Image:Pic_1.jpg|frame|none|Shigella dysenteriae Image copyright [Dennis Kunkel Microscopy, Inc http://denniskunkel.com]]] | ||
Habitat: ''S. dysenteriae'' are found worldwide but concentrate in areas with overcrowding, malnutrition, and inadequate waste management and unsafe drinking water supplies. ''S. dysenteriae'' have caused endemic dysentery in Africa, Southeast Asia, and the Indian subcontinent. Humans are the only natural hosts for ''S. dysenteriae'', though houseflies may serve as vectors for the transmission of ''S. dysenteriae''. Although ''S. dysenteriae'' has been found in contaminated food and water, this organism generally survives poorly outside the human body. | Habitat: ''S. dysenteriae'' are found worldwide but concentrate in areas with overcrowding, malnutrition, and inadequate waste management and unsafe drinking water supplies. ''S. dysenteriae'' have caused endemic dysentery in Africa, Southeast Asia, and the Indian subcontinent. Humans are the only natural hosts for ''S. dysenteriae'', though houseflies may serve as vectors for the transmission of ''S. dysenteriae''. Although ''S. dysenteriae'' has been found in contaminated food and water, this organism generally survives poorly outside the human body. |
Revision as of 02:24, 2 May 2007
A Microbial Biorealm page on the genus Shigella dysenteriae
Classification
Higher order taxa
Bacteria (domain), Proteobacteria (phylum), Gammaproteobacteria (class), Enterobacteriales (order), Enterobacteriaceae (family), Shigella (genus), dysenteriae (species)
Other names used: “Bacillus dysentericus" (Shiga 1897), “Eberthella dysenteriae" “Bacillus dysenteriae” (Shiga 1898), "Shigella shigae" “Bacillus shigae" (Chester 1901)
Description and significance
Physical characteristics: S. dysenteriae is a small, uncapsulated, non-motile, Gram-negative, nonsporulating bacillus (rod-shaped).
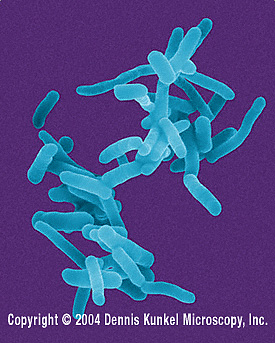
Habitat: S. dysenteriae are found worldwide but concentrate in areas with overcrowding, malnutrition, and inadequate waste management and unsafe drinking water supplies. S. dysenteriae have caused endemic dysentery in Africa, Southeast Asia, and the Indian subcontinent. Humans are the only natural hosts for S. dysenteriae, though houseflies may serve as vectors for the transmission of S. dysenteriae. Although S. dysenteriae has been found in contaminated food and water, this organism generally survives poorly outside the human body.
Significance: S. dysenteriae causes a significant threat to public health by causing shigellosis, especially in developing countries. Shigellosis is associated with 5-15% of cases of diarrhea and 30-50% of cases of dysentery worldwide. In malnourished children, shigellosis can cause a vicious cycle of further impaired nutrition absoprtion, recurrent infection, and growth retardation. Without proper care, shigellosis can become life-threatening. It is important for the genome of S. dysenteriae to be sequenced because an understanding of the genome of this organism might contribute to the successful development of a vaccine, which is still under development in laboratories (see Current Research).
Discovery: S. dysenteriae was first isolated by Kiyoshi Shiga in 1896 from stool of dysentery patients. When culture of the organism was fed to dogs, diarrhea ensued. He confirmed S. dysenteriae as the cause of the dysentery in these patients by a simple agglutination technique. Shiga demonstrated that the organism repeatedly coalesced when exposed to the serum of dysentery patients. In the years immediately following Shiga’s discovery, similar organisms were reported by other investigators, and over the next 40 years three additional related organisms were taxonomically placed in the genus Shigella and named S. dysenteriae, S. flexneri, S. boydii, and S. sonnei.
Genome structure
Strain Sd197 has been completely sequenced. The genome consists of 1 circular chromosome containing 4,369,232 nt (4664 genes). A plasmid containing 182,726 nt (224 genes) has also been sequenced.
The genome of S. dysenteriae has been shown to share many similarities with the genomes of other Shigella species and with that of E.coli K12 strain MG1655, but each has "over 200 pseudogenes, 300~700 copies of insertion sequence (IS) elements, and numerous deletions, insertions, translocations and inversions." There is great diversity of virulence genes, mostly acquired via bacteriophage-mediated lateral gene transfer. Lateral gene transfer has also been responsible for providing certain non-Shigella species the ability to produce Shiga toxins (Stx), the endotoxins that characterize the endotoxicity of the different Shigella species. For example, E. coli O157:H7 produces Stx and is also called Stx-producing E. coli (STEC). In addition, Stx-expressing Citrobacter freundii and Enterobacter cloacae strains have been described.
The plasmid has been shown to encode genes important for the virulence of all Shigella species. The invasion plasmid antigens (Ipa proteins) are required for invasion of the colonic and rectal epithelial cells and for the release of the bacteria into the host cell cytosol. One Ipa protein, IpaB, induces apoptosis in host macrophages and dendritic cells, leading to inflammatory reactions. The virulence plasmid also encodes a surface protein, IcsA, responsible for actin-based motility required for spreading of the organism in the host.
Strain 1012 is undergoing sequencing. So far the genome has been found to consist of 1 chromosome containing 3,013,140 nt (2782 genes), DNA typology is unspecified because sequencing is still incomplete.
Cell structure and metabolism
S. dysenteriae is a facultative anaerobe, i.e. it gains energy by aerobic respiration in the presence of oxygen and by anaerobic respiration or fermentation in the absence of oxygen. When it was first isolated, Shiga described that the organism fermented dextrose.
The most important molecules produced by S. dysenteriae are the Shiga toxins, toxins most responsible for the pathogenicity of this organism in humans. The prototype of Shiga toxin is found in S. dysenteriae serotype 1 and is named Stx. Various other Stx subtypes have been defined, including Stx1, Stx1c, Stx2, etc. There are also other molecules required for the virulence of S. dysenteriae, encoded on both the genomic chromosome and the plasmid (See Genome Structure).
Ecology
The most significant interaction of S. dysenteriae with other organisms is its pathogenic effects on human hosts, described in the following section.
Another significant interaction of S. dysenteriae with other organisms is its participation in lateral gene transfer with other pathogenic bacteria, “contributing” the Stx genes and endowing other bacteria with the ability to produce Shiga toxins.
Pathology
Shigella dysenteriae type 1 produces severe disease symptoms collectively called Shigellosis, which may be associated with life-threatening complications. The symptoms of shigellosis include diarrhea and dysentery, along with frequent mucoid bloody stools and abdominal cramps. S. dysenteriae causes dysentery by invading the colonic mucosa. The organism multiply within colonic epithelial cells, causing cell death, and spread laterally to infect and kill adjacent epithelial cells The infection causes mucosal ulceration, inflammation and bleeding. In most serious cases, Shigellosis can lead to acute renal failure, called the hemolytic uremic syndrome (HUS), and can also lead to CNS abnormalities.
Shiga toxin is responsible for most of the pathology of Shigellosis. Shiga toxin is neurotoxic, cytotoxic, and enterotoxic.
Enterotoxic effect: Shiga toxin binds to receptors on intestinal epithelial cells and blocks absorption (uptake) of electrolytes, glucose, and amino acids from the intestinal lumen. This enterotoxicity leads to diarrhea, dysentery, and dehydration, loss of electrolytes, and impaired nutrient absorption.
In addition, Stx damages intestinal capillaries by killing the cells of the blood vessels. Stx also triggers neutrophil-rich inflammation reactions in the intestines, causing further damage to the epithelial layers. (Stx is also able to cross the intestinal epithelial layer and enter the bloodstream. Once in the bloodstream, Stx attaches itself to circulating neutrophils and invades different cell-types, in particular, glomerular cells and CNS neurons, leading the renal failure and CNS abnormalities.)
Cytotoxic effect: B subunit of the Shiga toxin binds host cell glycolipid in large intestine, A1 domain of the Shiga toxin is internalized via receptor-mediated endocytosis and causes irreversible inactivation of the 60S ribosomal subunit, thereby inhibiting protein synthesis and thus causing cell death, damage to the intestinal blood vessels, and hemorrhage. There is also evidence that Stx can trigger apoptosis pathways in infected cells, leading to massive cell death.
Neurotoxic effect: Fever and abdominal cramping are considered signs of neurotoxicity. CNS abnormalities can also occur and include lethargy, disorientation, seizures, paralysis and coma. Neurotoxicity occurs most often in children and the elderly and is often fatal.
Application to Biotechnology
The ompA genes of Shigella dysenteriae have been used to construct a group of enterobacterial surface expression vectors for foreign genes. Foreign genes are inserted into the ompA genes and the resultant fusion proteins are expressed on cell-surface by the organism This fusion construct can be used as a vector system and has been used to transfer to the bacterial surface beta-galactosidase, foot-and-mouth disease virus (FMDV), malaria antigens, and influenza haemagglutinin. This vector system has implications in vaccine developments.
Current Research
1. Vaccine development: Human monocyte-derived dendritic cells (DC) and Balb/c mice were used as models to establish the immunogenic potential of formalin-inactivated Shigella species. Incubation of DC with inactivated or live bacteria induced DC maturation and cytokine release. Mice immunized orally or intranasally with killed S. flexneri, S. sonnei, or S. dysenteriae developed IgG and IgA to the homologous LPS. When infected with live homologous organisms, 80-100% survival was seen in all vaccinated groups compared to negligible survival in control groups.
2. Screening based on catalase: Since S. dysenteriae type 1 differs from most of the Enterobacteriaceae in that it does not produce catalase, a test for catalase may provide a useful screening method. The ability of a catalase test to provide rapid identification of S. dysenteriae type 1 was assessed using samples of S. dysenteriae, Shigella of other species, and entero-invasive (EIEC) and Shiga-toxin-producing Escherichia coli (STEC). All of the S. dysenteriae samples were found to be catalase-negative. All the other bacteria tested were positive for catalase. The catalase test is useful in the rapid identification of S. dysenteriae because of its high predictive value, simplicity and speed. It would be particularly useful during dysentery outbreaks, when other Shigella would be uncommon.
3. Characterization of iron uptake: S. dysenteriae has an outer-membrane receptor mediated pathways for the uptake and utilization of heme as an iron-source. An analysis of the outer-membrane receptor ShuA of S. dysenteriae was undertaken. Hemoglobin in the oxidized state was found to be the most likely physiological substrate for ShuA. In addition, mutation of the conserved histidines, H86A or H420A, resulted in a loss of the receptors’ ability to efficiently extract heme from hemoglobin. The double mutant H86A/H420A was unable to extract heme from hemoglobin at all. These findings taken together confirm that both His-86 and His-420 are essential for substrate recognition, heme coordination and transfer.
References
1. NCBI database entry “Shigella dysenteriae” http://www.ncbi.nlm.nih.gov/Taxonomy/Browser/wwwtax.cgi?mode=Info&id=622&lvl=3&p=mapview&p=has_linkout&p=blast_url&p=genome_blast&lin=f&keep=1&srchmode=1&unlock (retrieved on Apr 5, 2007)
2. Niyogi, S. “Shigellosis” The journal of microbiology. 2005. volume 43. p. 133 –43.
3. Dennis Kunkel Microscopy Inc. http://denniskunkel.com. (pictures retrieved on May 1, 2007)
4. NCBI Genome Database entry “Shigella dysenteriae” http://www.ncbi.nlm.nih.gov/entrez/query.fcgi?db=genome&cmd=search&term=txid622[orgn] (retrieved on Apr 5, 2007)
5. Yang, F.; Yang, J.; Zhang, X. “Genome dynamics and diversity of Shigella species, the etiologic agents of bacillary dysentery.” Nucleic Acids Research. 2005. volume 33. p.6445–6458.
6. Cherla, R.; Lee, S.; Vernon, T. “Shiga toxins and apoptosis.” FEMS microbiology letters. 2003. volume 228. p.159 –66
7. Hobom, G; Arnold, N Ruppert, A. “OmpA fusion proteins for presentation of foreign antigens on the bacterial outer membrane.” Developments in Biological Standardization. 1995. volume 84. p. 255-62.
8. Osorio, M.; Bray, M.; Walker, R. “Vaccine potential for inactivated shigellae.” Vaccine. 2007. volume 25. p.1581 –92
9. Karas, J. Pillay, P.; Sturm A. “The catalase reaction of Shigella species and its use in rapid screening for epidemic Shigella dysenteriae type 1.” Annals of tropical medicine and parasitology. 2007. volume 101. p.79 –84
10. Burkhard, K; Wilks, A. “Characterization of the outer-membrane receptor ShuA from the heme uptake system of shigella dysenteriae: Substrate specificity and identification of the heme protein ligands.” The Journal of biological chemistry. 2007, March 26. (article not yet in print as of Apr 10, 2007)
Edited by Julia Kao, student of Rachel Larsen