Prions and Encephalopathies: Difference between revisions
Line 15: | Line 15: | ||
==Prion Protein Structures as Interspecific Barriers for TSEs== | ==Prion Protein Structures as Interspecific Barriers for TSEs== | ||
[[Image:FISB.medium.gif|thumb|300px|right|Model of a healthy host prion protein proposed by Hagiwara et al. (2012)]] | [[Image:FISB.medium.gif|thumb|300px|right|Model of a healthy host prion protein proposed by Hagiwara et al. (2012)]] | ||
[[Image:FISB2.large.jpg|thumb|300px|right|Different models of pathogenic prion proteins proposed by Hagiwara et al. (2012)]] | [[Image:FISB2.large.jpg|thumb|300px|right|Different models of pathogenic prion proteins proposed by Hagiwara et al. (2012)]] |
Revision as of 18:49, 23 April 2013
Introduction
“Prion” is the name given to a class of proteins which can cause severe neurological diseases in a host of mammalian species, including humans. These disorders are a result of misfolding of naturally found proteins in host tissue. While the exact mechanisms of transmission from protein to protein are not completely understood, the general mechanism of infection is that existing misfolded proteins cause the same misfolding in these existing normal proteins. These misfolded proteins are extremely resilient– they are resistant to extreme temperatures, both dry and wet, as well as radiation.9 The accumulation of diseased prions in mammalian tissues, commonly in the nervous system, causes Transmissible Spongiform Encephalopathies (TSEs). This name reflects the appearance of the infected animal’s tissues after infection – the brain and related tissue resemble sponges because of the disintegration of the tissue by the misfolded protein. In general, these disorders are characterized by a long incubation period, followed by an aggressive and always fatal loss of brain and nervous system function. In addition, these diseases do not induce an inflammatory response in the diseased individual.2
Transmissible Spongiform Encephalopathies in Animals
Include some current research in each topic, with at least one figure showing data.
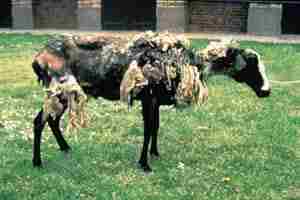
The Prion Protein and Mechanism of Infection
Include some current research in each topic, with at least one figure showing data.
Prion Protein Structures as Interspecific Barriers for TSEs
It is through this process that a TSE is spread from individual to individual within the same species. While this can also be the process between species, often there are differences between species’ normal prion protein, which can prevent infection. For example, Scrapie prions are unable to cause the disease within humans, while it is believed to have been transmitted into deer in the form of CWD. Differences between the different prion protein’s structure or folding is thought to be the main reason behind this phenomenon instead of deviations in the amino acid sequence of the protein. In the examination of host protein structure, Hagiwara et al. (2012) outlined specific features of healthy prion protein through nuclear magnetic resonance analysis. The N-terminus was found to be flexible, while the C-terminus was composed of three α-helices and two antiparallel β-pleated sheets. Of these structures on the C-terminus, the two β-pleated sheets and their connecting loop were found to be somewhat flexible as well. Through studies using different strains of TSEs and genetically engineered mice, researchers were able to make some conclusions. Mice with flexible loops and β-pleated sheets in their prion proteins were resistant to CWD prions, while mice with inflexible C-termini were resistant to Scrapie and BSE transmission.5
The analysis of disease causing protein structure has been more complicated, yet the study by Hagiwara et al. (2012) offers several different models through analysis of protein structure with electron microscopy. One model proposes that the N-terminus of the prion protein forms two loops consisting of parallel β-helices. In this model, the species barrier between mice and Syrian hamsters was thought to be the presence of a sulphur stack between Methionine amino acids of the adjacent loops of the hamster protein. A similar model suggests a four loop structure composed of β-helices at the N-terminus of the protein as well. In this model, the actual amino acid sequences within the β-helices are thought to cause some changes in the loops’ structure and shape, as the side chains of the amino acids compose the core of the helices. While no certain amino acid sequences can be ascribed to cause or prevent the transmission between species using this model, differences in the amino acid sequences of the helix regions has been noted between mice and hamsters, as well as between humans and sheep. The last model offered by Hagiwara et al. (2012) was based on nuclear magnetic resonance analysis and X-ray crystallography. This model proposes a spiral structure built of three β-pleated sheets as the main feature of the infectious prion protein. Through X-ray crystallography of synthetic peptide models of human, hamster, and mouse prion proteins, two subclasses of this model were proposed. In the mouse and human proteins, the β-pleated sheets were held together in a parallel zipper structure. The β-pleated sheets in the hamster protein, however, formed anti-parallel zipper structures. All of the models produced by this study are not exclusive, and perhaps it is a combination of these different structures that cause the interspecific barriers between proteins.5
Genetic Predictions of Susceptibility to TSEs in Carnivores
A study by Stewart et al. (2012) outlined predicted susceptibility to TSEs among carnivore species. TSEs are able to be transmitted successfully to many species through intercerebral injections, yet often only have a select number of target species to which the disease can be transferred through ingestion of the protein. While this study focused primarily on feline and canid transmission of prion diseases, its methods and results are applicable to the examination of oral transmission of the diseases through infected meat in other species. The study reinforces this applicability through proving that the digestion of prion proteins does not vary between carnivores, primates, or hooved animals.8
The large finding of the study was a difference of one codon in the prion protein coding sequence between felines and canids - the canine sequence contained either an aspartic acid or glutamic acid at codon 163. This difference is proposed to be the sole reason for the susceptibility of felines to contract TSEs. The researchers use this to explain how FSE occurred in zoos during BSE outbreaks because of infected meat, while no cases of canine prion diseases developed. The study also found the wolverine and pine marten, of all other carnivores, were the only other species to also have the aspartic or glutamic acid at codon 163, suggesting they are resistant to ingested infective BSE proteins.8
Aside from this large difference between felines and canids, prion protein polymorphisms within species were investigated as well. Within cats, the margay was found to be the most divergent, while there was absolutely no variation in prion protein sequence in mountain lions. Within feline species, individuals homozygous for a five alanine containing nonapeptide repeat were found to be the most susceptible to prion protein disease. Among canine species, the glutamic acid polymorphism at codon 163 was most common in domestic dogs, while the aspartic acid version was characteristic of gray wolves. Little variation was found in the sequences of black bears as well. This lack of genetic variation in prion protein sequence within both black bears and mountain lions could cause a uniform susceptibility to future TSEs.8
Treatment
As mentioned earlier, prions are resistant to extreme temperatures and radiation. However, Baxter et al. (2005) proved the effectiveness of radio frequency gas plasma treatment against misfolded prion proteins. In this study, both surgical instruments and stainless steel spheres were disinfected through radio frequency gas plasma treatment and then analyzed. Surgical instruments were analyzed for organic residues with scanning electron microscopy and x-ray spectroscopy, while the steel spheres were implanted into hamsters to determine the lethality of the treated prions. In the implantation section of this study, all steel spheres were contaminated with misfolded hamster prion proteins. Some of these underwent the radio frequency gas plasma treatment, while others underwent other forms of sanitizing. With these methods the researchers proved that radio frequency gas plasma treatment renders misfolded prion proteins non transmissible.1
Thus far, there have been several drugs developed which can be used to treat TSEs once the disease causing proteins enter the body. One drug has shown to have great potential – pentose polysulphate. In a study done by Farquhar et al. (1999), mice were injected with or orally consumed pentose polyphosphate before and after exposure to the Scrapie prion. The mice who received the drug prior to exposure experienced a lesser susceptibility to Scrapie, while those who received the drug after exposure had an extended incubation period and a lessening of the symptoms associated with Scrapie. In addition to this, several of the mice who were infected with Scrapie survived.4 Another form of treatment has also been developed by researchers in Switzerland and Sweden – luminescent proteins. In their study, luminescent conjugated polymers were applied to brain tissue of mice infected with a prion disease. This resulted in a significant decrease in both the number of misfolded prion proteins present in the tissue, as well as the proteins overall virulence. While these initial results are quite promising, this method of treatment has not yet been perfected for widespread use.3
References
1.^ Baxter, H., Campbell, G., Whittaker, A., Jones, C., Aitken, A., Simpson, A., Casey, M., Bountiff, L., Gibbard, L., and Baxter, R., 2005, Elimination of transmissible spongiform encephalopathy infectivity and decontamination of surgical instruments by using radio-frequency gas-plasma treatment, Journal General Virology, vol 86, pg 2393-2399
2.^ Centers for Disease Control and Prevention, 2013, Prion Diseases, http://www.cdc.gov/ncidod/dvrd/prions/index.htm
3.^ Dillow, C., 2012, First Treatment for Prion-Based Diseases Involves Glowing Polymers, Popular Science, http://www.popsci.com/science/article/2012-04/targeted-polymer-molecules-can-hunt-down-prions-brain, (4/22/2013)
4.^ Farquhar, C., Dickinson, A., and Bruce, M., 1999, Prophylactic potential of pentosan polysulphate in transmissible spongiform encephalopathies, The Lancet, vol 353, pg 117
5.^ Hagiwara, K., Hara, H., and Hanada, K., 2013, Species-barrier phenomenon in prion transmissibility from a viewpoint of protein science, Journal Biochemistry, vol 153, pg 139-145.
6.^ Hodel, L., 2004, Mad Cow Timeline, Mother Earth News,http://www.motherearthnews.com/real-food/mad-cow-timeline.aspx#axzz2REwNFBlC, (4/22/2013)
7.^ Imran, M., Mahmood, S., 2011, An overview of animal prion diseases, Virology Journal, http://www.virologyj.com/content/8/1/493, (4/22/2013)
8.^ Stewart, P., Campbell, L., Skogtvedt, S., Griffin, K., Arnemo, J., Tryland, M., Girling, S., Miller, M., Tranulis, M., and Goldmann, W., 2012, Genetic Predictions of Prion Disease Susceptibility in Carnivore Species Based on Variability of the Prion Gene Coding Region, PLoS ONE, http://www.plosone.org/article/info%3Adoi%2F10.1371%2Fjournal.pone.0050623, (4/22/2013)
9.^ Yam, P., 2011, Natural Born Prion Killers: Lichens Degrade “Mad Cow” Related Brain Pathogen, Scientific American, http://www.motherearthnews.com/real-food/mad-cow-timeline.aspx#axzz2REwNFBlC, (4/22/2013)
Edited by student of Joan Slonczewski for BIOL 238 Microbiology, 2009, Kenyon College.