Prions and Encephalopathies: Difference between revisions
Line 1: | Line 1: | ||
==Introduction== | ==Introduction== | ||
By Ian Stewart-Bates | By Ian Stewart-Bates | ||
[[Image:r7_prion.jpg|thumb|300px|right|Figure 1). Digital representation of an incorrectly folded prion protein. Image created by the Mayo Clinic.]] | [[Image:r7_prion.jpg|thumb|300px|right|Figure 1). Digital representation of an incorrectly folded prion protein. Image created by the Mayo Clinic. http://www.mayoclinic.com/health/medical/IM02291]] | ||
“Prion” is the name given to a class of proteins which can cause severe neurological diseases in a host of mammalian species, including humans. These disorders are a result of misfolding of naturally found proteins in host tissue (Figure 1). While the exact mechanisms of transmission from protein to protein are not completely understood, the general mechanism of infection is that existing misfolded proteins cause the same misfolding in these existing normal proteins. These misfolded proteins are extremely resilient– they are resistant to extreme temperatures, both dry and wet, as well as radiation.<sup>9</sup> The accumulation of diseased prions in mammalian tissues, commonly in the nervous system, causes Transmissible Spongiform Encephalopathies (TSEs). This name reflects the appearance of the infected animal’s tissues after infection – the brain and related tissue resemble sponges because of the disintegration of the tissue by the misfolded protein. In general, these disorders are characterized by a long incubation period, followed by an aggressive and always fatal loss of brain and nervous system function. In addition, these diseases do not induce an inflammatory response in the diseased individual.<sup>2</sup> | “Prion” is the name given to a class of proteins which can cause severe neurological diseases in a host of mammalian species, including humans. These disorders are a result of misfolding of naturally found proteins in host tissue (Figure 1). While the exact mechanisms of transmission from protein to protein are not completely understood, the general mechanism of infection is that existing misfolded proteins cause the same misfolding in these existing normal proteins. These misfolded proteins are extremely resilient– they are resistant to extreme temperatures, both dry and wet, as well as radiation.<sup>9</sup> The accumulation of diseased prions in mammalian tissues, commonly in the nervous system, causes Transmissible Spongiform Encephalopathies (TSEs). This name reflects the appearance of the infected animal’s tissues after infection – the brain and related tissue resemble sponges because of the disintegration of the tissue by the misfolded protein. In general, these disorders are characterized by a long incubation period, followed by an aggressive and always fatal loss of brain and nervous system function. In addition, these diseases do not induce an inflammatory response in the diseased individual.<sup>2</sup> | ||
Revision as of 18:16, 3 May 2013
Introduction
By Ian Stewart-Bates
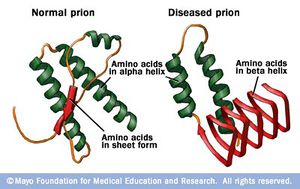
“Prion” is the name given to a class of proteins which can cause severe neurological diseases in a host of mammalian species, including humans. These disorders are a result of misfolding of naturally found proteins in host tissue (Figure 1). While the exact mechanisms of transmission from protein to protein are not completely understood, the general mechanism of infection is that existing misfolded proteins cause the same misfolding in these existing normal proteins. These misfolded proteins are extremely resilient– they are resistant to extreme temperatures, both dry and wet, as well as radiation.9 The accumulation of diseased prions in mammalian tissues, commonly in the nervous system, causes Transmissible Spongiform Encephalopathies (TSEs). This name reflects the appearance of the infected animal’s tissues after infection – the brain and related tissue resemble sponges because of the disintegration of the tissue by the misfolded protein. In general, these disorders are characterized by a long incubation period, followed by an aggressive and always fatal loss of brain and nervous system function. In addition, these diseases do not induce an inflammatory response in the diseased individual.2
Scrapie
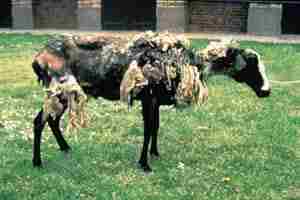
The earliest known prion disease is “Scrapie.” Found in sheep, goats, and various mountain goat species, this prion disease was first recorded in domestic sheep in 1732. The name “Scrapie” reflects a common behavior found in affected individuals – intense urges to scratch or itch are felt by the sheep, resulting in loss of wool when the sheep rubs or scrapes itself to relieve the itch (Figure 2). However, there are many other symptoms found in individuals affected by Scrapie. Often, the diseased animal has a significant change in behavior, suffers from tremors and locked muscles, and experiences a loss of movement, vision, and coordination. In goats, the disease causes several different symptoms: irritability, restlessness, posture abnormalities, grinding of teeth, and excessive salivation or regurgitation. The disease is easily diagnosed in live sheep by sampling cells from the nictating membrane of the eyelid. Dissection of the infected animal’s corpse commonly reveals the misfolded proteins presence in the nervous system, tonsils, muscles, placenta, colon and intestine, spleen and lymph nodes. The incubation period of Scrapie varies from 2-5 years, while the individual normally dies between 2 week and 6 months of the disease’s onset. Studies have found infected animals’ bodily fluids and waste products to be infectious, suggesting that the prion is commonly transmitted horizontally. The prion protein can lay dormant in soil for years, resulting in outbreaks years after infected individuals have been removed from the herd.7
Through analysis of infected individuals, several polymorphisms in sheep and goat amino acid coding genes have shown increased susceptibility to Scrapie. As outlined in Imran and Mahmood (2011), a three codon system based on these polymorphisms has been developed in sheep. This system used five alleles, which can be combined into 15 different genotypes. Analysis of these genotypes has highlighted one strongly resistant genotype, while five were identified as extremely susceptible to Scrapie. This research, in conjunction with selective breeding programs, has had success in reducing the prevalence of the prion disease in several European states. Fewer studies have been conducted to discern susceptible genotypes in goats, as the outbreaks of Scrapie in goats are less prevalent. However, six different polymorphisms have been linked to resistance to the prion disease. An interesting note is that genes carrying resistance to typical Scrapie in goats have been linked to increased susceptibility to atypical Scrapie.7
Bovine Spongiform Encephalopathy
The most widely known prion disease is Bovine Spongiform Encephalopathy (BSE), also known as “Mad Cow Disease.” The disease was first identified in 1986 in cattle herds in Great Britain. In 1993, the disease had spread to Canada, and by 2003 the United States had its first BSE case. In 1988, the British government began the banning of contaminated cattle feed and the elimination of infected cows. The USDA, on the other hand, implemented new regulations on cattle slaughter and processing seven days after the discovery of BSE in the US. This prion disease in particular is of great significance to human health: in 1996 the British government disclosed that BSE could be transmitted to humans through infected meat, resulting in variants of Creutzfeldt-Jakob disease (CJD).6 However, infected meat was also thought to be the means of transfer of the disease to other mammal species as well, resulting in Exotic Ungulate Spongiform Encephalopathy, Feline Spongiform Encephalopathy, and Non-human Primate Spongiform Encephalopathy.7
The symptoms of BSE are similar to that of Scrapie. The affected cow normally suffers from tremors, loss of hind limb mobility, excessive anxiety and overreaction to stimuli, and may display aggressive behavior. In post-mortem analysis, the misfolded protein is found to be concentrated primarily within the brain. Two atypical strains of BSE exist in addition to the typical variant – amyloid plaque buildup is a characteristic of these atypical strains. The usual incubation period of the disease is 2-8 years, with cattle aged 4-5 years the most common victims. Unlike Scrapie, there is little evidence that the disease is easily spread horizontally through bodily excretions or fluids – the misfolded proteins have not been detected in semen, milk or embryonic fluid. The disease has been detected in European cattle (Bos Taurus), Zebu, and a hybrid of the two species. In particular, .2 million cases of BSE have been documented in the Holstein-Freisian breed of European cow. While several polymorphisms of the prion protein have been identified in cattle, only three of these have been associated with susceptibility to BSE.7
Chronic Wasting Disease
Chronic wasting disease (CWD) is a prion disease similar to Scrapie and BSE, yet is found in wild populations of deer, elk, and moose. The first documented case was in 1967, ruling out the transmission of BSE from cattle to deer. However, it is suspected that the disease may have erupted from a transmission of Scrapie to deer species, as studies have shown the Scrapie prion successfully infects elk. As the name suggests, the affected individual suffers from a wasting of muscle tissue as a symptom. In addition, the misfolded protein causes a loss of fear of humans, retention of patches of winter coat during summer months, excessive salivation, loss of movement or repetitive pacing, sudden death, bacterial pneumonia, as well as other abnormal behaviors. Incubation periods of CWD range from 16 months to 5 years, with death resulting within a year of symptoms. The CWD prion is found in almost every tissue in infected individuals. In addition, analyses of carcasses often reveal lesions in regions responsible for producing anti-diuretic hormones in the hypothalamus, as a result of extreme dehydration.7
The disease is spread among wild populations through environmental exposure or contact with diseased individuals. Like in Scrapie, the prion protein is often found in soil inhabited by diseased individuals, causing the disease in herds that graze from the soil. Infected individuals deposit the misfolded protein to soil through excrement, carcasses, placenta, blood and saliva. CWD can be spread to sheep, cattle, minks, squirrel monkeys, and various rodents though intracerebral transmission. However, the CWD prion has not been found to cause disease in humans who have eaten venison or been exposed to infected animals.7
Recently, researchers at the U.S. Geological Survey of the National Wildlife Health Center have discovered an unlikely cure for CWD. Three species of lichens have been identified to secrete enzymes which naturally degrade the misfolded protein. While the testing was undertaken in a lab, the researchers believe the lichens can inactivate proteins within the soil, as well as possibly protect deer that eat the lichens.9 In addition to this, three polymorphisms of the prion protein have been identified as conferring CWD resistance to species at risk. However, as the affected species are wild population, selective breeding has limited applications for disease control.7
Exotic Ungulate Spongiform Encephalopathy, Feline Spongiform Encephalopathy, and Non-human Primate TSE
All of these prion diseases are thought to have been initially caused by interspecific transfer of BSE through bone meal or meat in animal feed: mice injected with EUE, FSE, and NHP prions formed similar lesions within nervous system tissue and had similar incubation periods as mice injected with BSE prions. In addition, these cases were concurrent with the outbreak of BSE in Great Britain, and ceased with the control of BSE. The majority of outbreaks were recorded in zoos. EUE was found in 6 elands and greater kudu, 2 Arabian Oryx and ankole cows, as well as a gemsbok, spiral horned antelope, bison, and Scimitar Horned Oryx. Symptoms of EUE vary from species to species. FSE has been documented in about 100 domestic cats, and 29 wild cats, including cheetahs, pumas, tigers, lions, ocelots, and Asian golden cats. Common symptoms in felines are increased aggression or unusual timidity, cease of grooming, restlessness, excessive hunger, loss of coordination, and loss of movement in the hind legs. Diseased domestic cats normally die within 3-8 weeks of symptoms, while larger cats within 8-10 weeks.Non-human Primate TSE has only been documented in Mayotte brown lemurs, white fronted brown lemurs, mongoose lemurs, and Rhesus macaques.7
The Prion Protein and Mechanism of Infection
The symptoms of the above diseases are thought to be direct results of the conversion of the organism’s normal prion protein into the misfolded version. The amino acid sequences of these initial normal prion proteins are well conserved in most mammal species. The most common prion protein used for studies on TSEs comes from mice, consisting of 254 amino acids. This protein contains a signal peptide at its N-terminus, two asparagines for nitrogen glycosylation, and a signal peptide at the C-terminus for attaching to an anchoring mechanism. While the exact functions of the natural prion protein are not completely understood, it may be involved with the uptake of metal ions, oxidative stress response, cell to cell recognition or communication.5
In comparison to the normal protein, disease causing prion proteins have several distinguishing features. First, the disease causing protein is unable to be broken down completely by proteinase K. Instead, mass spectrometry has revealed that C-terminus half of the original protein is left behind by the enzyme. Another difference between the healthy and disease causing prion is the percent composition of β-pleated sheets and α-helices. Through infrared spectroscopy, normal prion proteins are estimated to be composed of around 40% α-helices. In contrast, disease causing prion proteins are roughly 55% β-pleated sheets and 20% α-helices. The high amounts of β-pleated sheets are thought to give the misfolded protein its pathogenic qualities.5
The conversion of a healthy prion protein to a disease causing prion begins with the binding of the healthy protein to an original misfolded prion, normally within a plasma membrane. This suggests the necessity for there to be initial unhealthy prion proteins to be introduced to the body; researchers are unsure if misfolded prion proteins can be spontaneously created. These misfolded proteins replicate to form fiber like chains, eventually forming amyloid tissue. These prions are then spread from cell to cell within the diseased individual’s body through vesicles.5
Prion Protein Structures as Interspecific Barriers for TSEs
It is through this process that a TSE is spread from individual to individual within the same species. While this can also be the process between species, often there are differences between species’ normal prion protein, which can prevent infection. For example, Scrapie prions are unable to cause the disease within humans, while it is believed to have been transmitted into deer in the form of CWD. Differences between the different prion protein’s structure or folding is thought to be the main reason behind this phenomenon instead of deviations in the amino acid sequence of the protein. In the examination of host protein structure, Hagiwara et al. (2012) outlined specific features of healthy prion protein through nuclear magnetic resonance analysis. The N-terminus was found to be flexible, while the C-terminus was composed of three α-helices and two antiparallel β-pleated sheets (Figure 3). Of these structures on the C-terminus, the two β-pleated sheets and their connecting loop were found to be somewhat flexible as well. Through studies using different strains of TSEs and genetically engineered mice, researchers were able to make some conclusions. Mice with flexible loops and β-pleated sheets in their prion proteins were resistant to CWD prions, while mice with inflexible C-termini were resistant to Scrapie and BSE transmission.5
The analysis of disease causing protein structure has been more complicated, yet the study by Hagiwara et al. (2012) offers several different models through analysis of protein structure with electron microscopy. One model proposes that the N-terminus of the prion protein forms two loops consisting of parallel β-helices. In this model, the species barrier between mice and Syrian hamsters was thought to be the presence of a sulphur stack between Methionine amino acids of the adjacent loops of the hamster protein. A similar model suggests a four loop structure composed of β-helices at the N-terminus of the protein as well. In this model, the actual amino acid sequences within the β-helices are thought to cause some changes in the loops’ structure and shape, as the side chains of the amino acids compose the core of the helices. While no certain amino acid sequences can be ascribed to cause or prevent the transmission between species using this model, differences in the amino acid sequences of the helix regions has been noted between mice and hamsters, as well as between humans and sheep (Figure 4). The last model offered by Hagiwara et al. (2012) was based on nuclear magnetic resonance analysis and X-ray crystallography. This model proposes a spiral structure built of three β-pleated sheets as the main feature of the infectious prion protein. Through X-ray crystallography of synthetic peptide models of human, hamster, and mouse prion proteins, two subclasses of this model were proposed. In the mouse and human proteins, the β-pleated sheets were held together in a parallel zipper structure. The β-pleated sheets in the hamster protein, however, formed anti-parallel zipper structures. All of the models produced by this study are not exclusive, and perhaps it is a combination of these different structures that cause the interspecific barriers between proteins.5
Genetic Predictions of Susceptibility to TSEs in Carnivores
A study by Stewart et al. (2012) outlined predicted susceptibility to TSEs among carnivore species. TSEs are able to be transmitted successfully to many species through intercerebral injections, yet often only have a select number of target species to which the disease can be transferred through ingestion of the protein. While this study focused primarily on feline and canid transmission of prion diseases, its methods and results are applicable to the examination of oral transmission of the diseases through infected meat in other species. The study reinforces this applicability through proving that the digestion of prion proteins does not vary between carnivores, primates, or hooved animals.8
The large finding of the study was a difference of one codon in the prion protein coding sequence between felines and canids - the canine sequence contained either an aspartic acid or glutamic acid at codon 163. This difference is proposed to be the sole reason for the susceptibility of felines to contract TSEs. The researchers use this to explain how FSE occurred in zoos during BSE outbreaks because of infected meat, while no cases of canine prion diseases developed. The study also found the wolverine and pine marten, of all other carnivores, were the only other species to also have the aspartic or glutamic acid at codon 163, suggesting they are resistant to ingested infective BSE proteins.8
Aside from this large difference between felines and canids, prion protein polymorphisms within species were investigated as well. Within cats, the margay was found to be the most divergent, while there was absolutely no variation in prion protein sequence in mountain lions. Within feline species, individuals homozygous for a five alanine containing nonapeptide repeat were found to be the most susceptible to prion protein disease. Among canine species, the glutamic acid polymorphism at codon 163 was most common in domestic dogs, while the aspartic acid version was characteristic of gray wolves. Little variation was found in the sequences of black bears as well. This lack of genetic variation in prion protein sequence within both black bears and mountain lions could cause a uniform susceptibility to future TSEs.8
Prevention and Treatment
As mentioned earlier, prions are resistant to extreme temperatures and radiation. This has made prevention of the disease difficult in medical settings, as normal methods of disinfection have proven ineffective against removing prions from surgical instruments or other sanitary surfaces. However, Baxter et al. (2005) proved the effectiveness of radio frequency gas plasma treatment against misfolded prion proteins. In this study, both surgical instruments and stainless steel spheres were disinfected through radio frequency gas plasma treatment and then analyzed for pathogenicity. Surgical instruments were analyzed for organic residues with scanning electron microscopy and x-ray spectroscopy, while the steel spheres were implanted into hamsters to determine the lethality of the treated prions. In the implantation section of this study, all steel spheres were contaminated with misfolded hamster prion proteins. Some of these underwent the radio frequency gas plasma treatment, while others underwent other forms of sanitizing. With these methods the researchers proved that radio frequency gas plasma treatment renders misfolded prion proteins non transmissible.1
Thus far, there have been several drugs developed which can be used to treat TSEs once the disease causing proteins enter the body. One drug has shown to have great potential – pentosan polysulphate. In a study done by Farquhar et al. (1999), mice were injected with or orally consumed pentosan polyphosphate before and after exposure to the Scrapie prion. The mice who received the drug prior to exposure experienced a lesser susceptibility to Scrapie, while those who received the drug after exposure had an extended incubation period and a lessening of the symptoms associated with Scrapie. In addition to this, several of the mice who were infected with Scrapie survived.4 Another form of treatment has also been developed by researchers in Switzerland and Sweden – luminescent proteins. In their study, luminescent conjugated polymers were applied to brain tissue of mice infected with a prion disease. This resulted in a significant decrease in both the number of misfolded prion proteins present in the tissue, as well as the proteins overall virulence. While these initial results are quite promising, this method of treatment has not yet been perfected for widespread use.3
References
1.^ Baxter, H., Campbell, G., Whittaker, A., Jones, C., Aitken, A., Simpson, A., Casey, M., Bountiff, L., Gibbard, L., and Baxter, R., 2005, Elimination of transmissible spongiform encephalopathy infectivity and decontamination of surgical instruments by using radio-frequency gas-plasma treatment, Journal General Virology, vol 86, pg 2393-2399, http://vir.sgmjournals.org/content/86/8/2393.full.pdf+html
2.^ Centers for Disease Control and Prevention, 2013, Prion Diseases, http://www.cdc.gov/ncidod/dvrd/prions/index.htm
3.^ Dillow, C., 2012, First Treatment for Prion-Based Diseases Involves Glowing Polymers, Popular Science, http://www.popsci.com/science/article/2012-04/targeted-polymer-molecules-can-hunt-down-prions-brain, (4/22/2013)
4.^ Farquhar, C., Dickinson, A., and Bruce, M., 1999, Prophylactic potential of pentosan polysulphate in transmissible spongiform encephalopathies, The Lancet, vol 353, pg 117, http://www.sciencedirect.com/science/article/pii/S0140673698053951
5.^ Hagiwara, K., Hara, H., and Hanada, K., 2013, Species-barrier phenomenon in prion transmissibility from a viewpoint of protein science, Journal Biochemistry, vol 153, pg 139-145, http://jb.oxfordjournals.org/content/153/2/139.long
6.^ Hodel, L., 2004, Mad Cow Timeline, Mother Earth News,http://www.motherearthnews.com/real-food/mad-cow-timeline.aspx#axzz2REwNFBlC, (4/22/2013)
7.^ Imran, M., Mahmood, S., 2011, An overview of animal prion diseases, Virology Journal, http://www.virologyj.com/content/8/1/493, (4/22/2013)
8.^ Stewart, P., Campbell, L., Skogtvedt, S., Griffin, K., Arnemo, J., Tryland, M., Girling, S., Miller, M., Tranulis, M., and Goldmann, W., 2012, Genetic Predictions of Prion Disease Susceptibility in Carnivore Species Based on Variability of the Prion Gene Coding Region, PLoS ONE, http://www.plosone.org/article/info%3Adoi%2F10.1371%2Fjournal.pone.0050623, (4/22/2013)
9.^ Yam, P., 2011, Natural Born Prion Killers: Lichens Degrade “Mad Cow” Related Brain Pathogen, Scientific American, http://www.motherearthnews.com/real-food/mad-cow-timeline.aspx#axzz2REwNFBlC, (4/22/2013)
Edited by student of Joan Slonczewski for BIOL 238 Microbiology, 2013, Kenyon College.