Bdellovibrio exovorus: Difference between revisions
No edit summary |
|||
(7 intermediate revisions by the same user not shown) | |||
Line 2: | Line 2: | ||
[[File:Bdellovibrio_exovorus.png|400px|thumb|right|Thin sections of cells of <I>B. exovorus </I> strain JSS attached to intact (a) | [[File:Bdellovibrio_exovorus.png|400px|thumb|right|Thin sections of cells of <I>B. exovorus </I> strain JSS attached to intact (a) | ||
and empty (b) stalked cells of Caulobacter crescentus CB2A (5).]] | and empty (b) stalked cells of <I>Caulobacter crescentus</I> CB2A (5).]] | ||
==Classification== | ==Classification== | ||
Line 29: | Line 29: | ||
==Description and Significance== | ==Description and Significance== | ||
<B>Origin and Nomenclature</B> | <B>Origin and Nomenclature:</B> | ||
<I>Bdellovibrio exovorus</i> is a parasitic, predatory bacterium whose name means “Leech-like vibrating outside-devourer” (Gr. <I>Bdella</I>, leech, sucker; L. <I>vibrio</I>, vibrating; Gr. <I>exo-</I>, outside, L. <I>-vorus</I>, to eat.) (5). <I>B. exovorus</I> was first isolated from sewage in London, Ontario, Canada, and sequenced in 2013 by the Weizmann Institute of Science (1). | <I>Bdellovibrio exovorus</i> is a parasitic, predatory bacterium whose name means “Leech-like vibrating outside-devourer” (Gr. <I>Bdella</I>, leech, sucker; L. <I>vibrio</I>, vibrating; Gr. <I>exo-</I>, outside, L. <I>-vorus</I>, to eat.) (5). <I>B. exovorus</I> was first isolated from sewage in London, Ontario, Canada, and sequenced in 2013 by the Weizmann Institute of Science (1). | ||
<B>Morphological characteristics</B> | <B>Morphological characteristics:</B> | ||
<I>B. exovorus</I> appears as gram-negative, comma-shaped rods, about 0.5 | <I>B. exovorus</I> appears as gram-negative, comma-shaped rods, about 0.5 μm wide and 0.5-1.4 μm long. They have a single, polar, sheathed flagellum that is 29 nm wide. It is very motile during its attack phase, moving at speeds faster than 100 cell lengths per second (2,3). These obligate predators grow as small plaques on lawns of prey bacteria. <I>B. exovorus'</I> most common prey cell is <I>Caulobacter crescentus,</I> and is rather selective in the prey cells it attacks, as seen by its inability to prey upon <I>Escherichia coli</I>, a species that is commonly targeted by other <I>Bdellovibrio</I> (5). | ||
Line 56: | Line 56: | ||
No extra-chromosomal elements like plasmids were detected (6). | No extra-chromosomal elements like plasmids were detected (6). | ||
During initial characterization, | During initial characterization, Southern blotting was used with a DIG-labelled probe at the <I>hit</I> locus (short for "host interaction"), a gene that was suggested to be indicative of known <I>bdellovibrio</I> species. However, the results turned back negative, despite the significant genomic similarities between <I>B. exovorus</I> and <I>B. bacteriovorus</I>, suggesting that <I>B. exovorus</I> was indeed a new species of <I>Bdellovibrio</I> (5). | ||
Directed PCR reactions were also used to amplify other short regions of DNA to confirm their sequences, as well as to confirm the repeats in the CRISPR region of <I>B. exovorus</I> strain JSS (3). | Directed PCR reactions were also used to amplify other short regions of DNA to confirm their sequences, as well as to confirm the repeats in the CRISPR region of <I>B. exovorus</I> strain JSS (3). | ||
Line 71: | Line 71: | ||
[[File:Bdellovibrio_exovorus_epibiotic_life_cycle.png|400px|thumb|right|Epibiotic life cycle of <I>B. exovorus.</I> i) Motile attack phase cell (black arrow); ii) Attachment of <I>B. exovorus</I> to prey cell, <I>Stenotrophomonas maltophilia</I> (red arrow); iii) Replication of <I>B. exovorus</I> by binary fission; iv) Prey cell cytoplasmic content depleted, leaving behind an empty ghost cell (black arrowhead) (3).]] | [[File:Bdellovibrio_exovorus_epibiotic_life_cycle.png|400px|thumb|right|Epibiotic life cycle of <I>B. exovorus.</I> i) Motile attack phase cell (black arrow); ii) Attachment of <I>B. exovorus</I> to prey cell, <I>Stenotrophomonas maltophilia</I> (red arrow); iii) Replication of <I>B. exovorus</I> by binary fission; iv) Prey cell cytoplasmic content depleted, leaving behind an empty ghost cell (black arrowhead) (3).]] | ||
<I>Bdellovibrio exovorus</I> is an obligate predator, gathering nutrients by feeding on other gram-negative bacterial species like <I> | <I>Bdellovibrio exovorus</I> is an obligate predator, gathering nutrients by feeding on other gram-negative bacterial species like <I>C. crescentus</I> and <I>Stenotrophomonas maltophilia</I> (4,5). However, it is somewhat selective in the organisms it preys upon, as evidenced by its inability to use species like <I>E. coli</I> as prey, despite them also possessing gram-negative cell walls (3,4). | ||
<B>Life Cycle:</B> | <B>Life Cycle:</B> | ||
The life cycle of <I>B. exovorus</I> has two phases: a free-swimming attack phase and a stationary growth phase. In the first phase, <I>B. exovorus</I> rapidly propels itself via its flagellum to randomly attack a prey cell, attaching to the exterior of its cell wall. In this phase, <I>B. exovorus</I> has no mechanism for DNA replication or cell division, so if it does not attach to a host cell within a relatively- | The life cycle of <I>B. exovorus</I> has two phases: a free-swimming attack phase and a stationary growth phase. In the first phase, <I>B. exovorus</I> rapidly propels itself via its flagellum to randomly attack a prey cell, attaching to the exterior of its cell wall. In this phase, <I>B. exovorus</I> has no mechanism for DNA replication or cell division, so if it does not attach to a host cell within a relatively-short amount of time, it will die (6). In the second phase, <I>B. exovorus</I> becomes permanently attached to the host cell. <I>B. exovorus</I> releases hydrolytic enzymes into the host's cytoplasm, degrading its contents and transporting them back to the cell surface for <I>B. exovorus</I> to absorb. From here, <I>B. exovorus</I> can continue to grow and replicate, producing daughter cells while remaining attached to the cell. <I>B. exovorus</I> will continue elongation and binary fission until the host prey cell is completely emptied of its cytoplasmic structures and nutrients, making the prey into an empty "ghost cell" (3). | ||
All other known plaque-forming species within the family <I> Bdellovibrionaceae </I> are identified by their growth within the periplasmic space of their prey bacteria. However, <I>B. exovorus</I> has no periplasmic phase in its life cycle. Because <I>B. exovorus</I> does not inhabit the periplasmic space, it does not form the rounded bdelloplast out of the host cell that is common among members of the genus <I>Bdellovibrio</I>, or even related genera like <I>Bacteriovorax, Bacteriolyticum,</I> and <I>Predibacter</I> (3). | All other known plaque-forming species within the family <I> Bdellovibrionaceae </I> are identified by their growth within the periplasmic space of their prey bacteria. However, <I>B. exovorus</I> has no periplasmic phase in its life cycle. Because <I>B. exovorus</I> does not inhabit the periplasmic space, it does not form the rounded bdelloplast out of the host cell that is common among members of the genus <I>Bdellovibrio</I>, or even related genera like <I>Bacteriovorax, Bacteriolyticum,</I> and <I>Predibacter</I> (3). | ||
Line 85: | Line 85: | ||
<B>Metabolism and Nutrients:</B> | <B>Metabolism and Nutrients:</B> | ||
<I>B. exovorus</I> uses complete metabolic pathways like glycolysis and the TCA cycle, and can complete the pentose phosphate pathway non-oxidatively from D-ribose-5-phosphate. It undergoes complete amino acid biosynthesis for alanine, cysteine, aspartic acid, glutamic acid, glycine, glutamine, serine, threonine, tyrosine, and possibly lysine. It can undergo complete <I>de novo</I> nucleotide biosynthesis for dCTP and dTTP, and possibly for dATP and | <I>B. exovorus</I> uses complete metabolic pathways like glycolysis and the TCA cycle, and can complete the pentose phosphate pathway non-oxidatively from D-ribose-5-phosphate. It undergoes complete amino acid biosynthesis for alanine, cysteine, aspartic acid, glutamic acid, glycine, glutamine, serine, threonine, tyrosine, and possibly lysine; all other amino acids must be incorporated from prey cells. It can undergo complete <I>de novo</I> nucleotide biosynthesis for dCTP and dTTP, and possibly for dATP and dGTP using inosine as a starting compound. Fatty acids can also be completely degraded and metabolized, presumably into acetyl-coA for use in the TCA cycle (3). | ||
<I>B. exovorus</I> also contains cytochrome C within its electron transport chain, but it lacks catalase, superoxide dismutase, or peroxides needed to break down oxidative radicals (3). | <I>B. exovorus</I> also contains cytochrome C within its electron transport chain, but it lacks catalase, superoxide dismutase, or peroxides needed to break down oxidative radicals (3). | ||
Line 101: | Line 101: | ||
<B>Waste and By-Products:</B> | <B>Waste and By-Products:</B> | ||
No waste, by-products, or volatile compounds appear to have been explicitly discussed in the literature regarding <I>B. exovorus</I>, although the ghost cells left behind after consuming the cytoplasmic contents of a host cell could be considered a waste product (3). <I>B. exovorus</I> also sheds its flagellum once entering the growth phase, so perhaps that could be considered a | No waste, by-products, or volatile compounds appear to have been explicitly discussed in the literature regarding <I>B. exovorus</I>, although the ghost cells left behind after consuming the cytoplasmic contents of a host cell could be considered a waste product (3). <I>B. exovorus</I> also sheds its flagellum once entering the growth phase, so perhaps that could be considered a waste product as well (3). | ||
==Ecology / Pathology== | ==Ecology / Pathology== | ||
Line 121: | Line 121: | ||
[[File:Bdellovibrio_exovorus_on_Stenotrophomonas_maltophilia.png|300px|thumb|right|Epibiotic life cycle of <I>B. exovorus</I> FFRS-5 confirmed by thin section transmission electron microscopy. Thin section of <I>B. exovorus</I> FFRS-5 (black arrowhead) at the surface of <I>S. maltophilia</I> (black arrow) cell during predation. A small amount of cytoplasmic contents remains in the <I>S. maltophilia</I> prey cell (3).]] | [[File:Bdellovibrio_exovorus_on_Stenotrophomonas_maltophilia.png|300px|thumb|right|Epibiotic life cycle of <I>B. exovorus</I> FFRS-5 confirmed by thin section transmission electron microscopy. Thin section of <I>B. exovorus</I> FFRS-5 (black arrowhead) at the surface of <I>S. maltophilia</I> (black arrow) cell during predation. A small amount of cytoplasmic contents remains in the <I>S. maltophilia</I> prey cell (3).]] | ||
Currently, <I>B. exovorus</I> strain FFRS-5 (the first strain known to attack a bacterium other than <I>C. crescentus</I>) is being studied as an effective treatment for the newly-emerging opportunistic pathogen <I> | Currently, <I>B. exovorus</I> strain FFRS-5 (the first strain known to attack a bacterium other than <I>C. crescentus</I>) is being studied as an effective treatment for the newly-emerging opportunistic pathogen <I>S. maltophilia</I>, which although it increases plant growth and in the root nodules of other plants, and kills plant pathogens, it is inherently very resistant to antibiotics, heavy metals, and polycyclic aromatic hydrocarbons (3). Because of this, hospital patients with compromised immune systems are susceptible to infection from <I>S. maltophilia</I>. Symptoms of infection include pneumonia, bacteremia, urinary tract and soft tissue infections, eye infections, endocarditis, and meningitis. The bacterium is also being increasingly found alongside <I>P. aeruginosa</I> in cases of cystic fibrosis. While it is not very virulent, <I>S. maltophilia</I> can colonize rapidly, forming biofilms in harsh environments and thus causing infections (4). | ||
In order to treat infection, neither antibiotics nor phage therapy have proven effective (phages are too strain-specific and potentially transfer resistance genes to their host), leaving bacterial predators as a potential method of treatment. Because they are unable to attack eukaryotic cells, and have surprisingly high antibiotic levels themselves, they make an excellent candidate for use as a live antimicrobial. In combination with the antibiotic ciprofloxacin, <I>B. exovorus</I> was able to significantly lower biofilm masses produced by <I>S. maltophilia</I> in both laboratory and clinical settings as recently as 2016 (3,4). | In order to treat infection, neither antibiotics nor phage therapy have proven effective (phages are too strain-specific and potentially transfer resistance genes to their host), leaving bacterial predators as a potential method of treatment. Because they are unable to attack eukaryotic cells, and have surprisingly high antibiotic levels themselves, they make an excellent candidate for use as a live antimicrobial. In combination with the antibiotic ciprofloxacin, <I>B. exovorus</I> was able to significantly lower biofilm masses produced by <I>S. maltophilia</I> in both laboratory and clinical settings as recently as 2016 (3,4). |
Latest revision as of 19:09, 2 May 2017
Classification
Higher order taxa
Domain: Bacteria
Phylum: Proteobacteria
Class: Deltaproteobacteria
Order: Bdellovibrionales
Family: Bdellovibrionaceae
Species
NCBI: Taxonomy Bdellovibrio exovorus |
Bdellovibrio exovorus
Description and Significance
Origin and Nomenclature:
Bdellovibrio exovorus is a parasitic, predatory bacterium whose name means “Leech-like vibrating outside-devourer” (Gr. Bdella, leech, sucker; L. vibrio, vibrating; Gr. exo-, outside, L. -vorus, to eat.) (5). B. exovorus was first isolated from sewage in London, Ontario, Canada, and sequenced in 2013 by the Weizmann Institute of Science (1).
Morphological characteristics:
B. exovorus appears as gram-negative, comma-shaped rods, about 0.5 μm wide and 0.5-1.4 μm long. They have a single, polar, sheathed flagellum that is 29 nm wide. It is very motile during its attack phase, moving at speeds faster than 100 cell lengths per second (2,3). These obligate predators grow as small plaques on lawns of prey bacteria. B. exovorus' most common prey cell is Caulobacter crescentus, and is rather selective in the prey cells it attacks, as seen by its inability to prey upon Escherichia coli, a species that is commonly targeted by other Bdellovibrio (5).
Existing genus MicrobeWiki Link: https://microbewiki.kenyon.edu/index.php/Bdellovibrio
Genome and Genetics
Closest Relatives:
Bdellovibrio exovorus belongs to the major branch of prokaryotes of deltaproteobacteria. Despite many differences in life cycle and functionality, its closest relative species based on genetic similarity is Bdellovibrio bacteriovorus. Based on 16S rRNA sequences of B. bacteriovorus, the most closely related genera would therefore include Desulfomonile, Desulfuromonas, and more distantly, Bacteriovorax (2).
Extra-chromosomal Elements and Genetic Tools:
No extra-chromosomal elements like plasmids were detected (6).
During initial characterization, Southern blotting was used with a DIG-labelled probe at the hit locus (short for "host interaction"), a gene that was suggested to be indicative of known bdellovibrio species. However, the results turned back negative, despite the significant genomic similarities between B. exovorus and B. bacteriovorus, suggesting that B. exovorus was indeed a new species of Bdellovibrio (5).
Directed PCR reactions were also used to amplify other short regions of DNA to confirm their sequences, as well as to confirm the repeats in the CRISPR region of B. exovorus strain JSS (3).
Transposon mutagenesis has been used to identify genes used to attach to solid surfaces for predation. This includes the gene bd3852, which codes for the protein PilT2, an ATP-dependent motor that helps depolymerize the protein PilA, a component of type IV pili in host cells (3).
Genome Sequencing:
The genome sequence of B. exovorus was determined using whole genome sequencing (Weizmann Institute of Science, 2013), and can be found in its entirety on the Joint Genome Institute database. It has a total genome size of 2,657,893 bases, a %G+C content of 41.92%, and 2,656 genes. Of those genes, 2,619 (98.61%) are protein coding genes, 37 (1.39%) are RNA genes (of which 34 are for tRNA and 3 are for rRNA), and 1 (0.04%) is a pseudogene. Its 16S rRNA gene is 1502 bp long, and is only 90-93% similar to other strains of B. bacteriovorus. The bacterium produces a total of 2669 proteins, of which 130 are hydrolytic enzymes used to drain prey bacteria of their cytoplasmic contents (1).
Nutrition and Metabolism
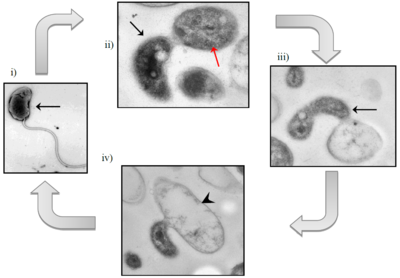
Bdellovibrio exovorus is an obligate predator, gathering nutrients by feeding on other gram-negative bacterial species like C. crescentus and Stenotrophomonas maltophilia (4,5). However, it is somewhat selective in the organisms it preys upon, as evidenced by its inability to use species like E. coli as prey, despite them also possessing gram-negative cell walls (3,4).
Life Cycle:
The life cycle of B. exovorus has two phases: a free-swimming attack phase and a stationary growth phase. In the first phase, B. exovorus rapidly propels itself via its flagellum to randomly attack a prey cell, attaching to the exterior of its cell wall. In this phase, B. exovorus has no mechanism for DNA replication or cell division, so if it does not attach to a host cell within a relatively-short amount of time, it will die (6). In the second phase, B. exovorus becomes permanently attached to the host cell. B. exovorus releases hydrolytic enzymes into the host's cytoplasm, degrading its contents and transporting them back to the cell surface for B. exovorus to absorb. From here, B. exovorus can continue to grow and replicate, producing daughter cells while remaining attached to the cell. B. exovorus will continue elongation and binary fission until the host prey cell is completely emptied of its cytoplasmic structures and nutrients, making the prey into an empty "ghost cell" (3).
All other known plaque-forming species within the family Bdellovibrionaceae are identified by their growth within the periplasmic space of their prey bacteria. However, B. exovorus has no periplasmic phase in its life cycle. Because B. exovorus does not inhabit the periplasmic space, it does not form the rounded bdelloplast out of the host cell that is common among members of the genus Bdellovibrio, or even related genera like Bacteriovorax, Bacteriolyticum, and Predibacter (3).
Replication in B. exovorus occurs via binary fission, producing two daughter cells that begin the attack phase (3).
Metabolism and Nutrients:
B. exovorus uses complete metabolic pathways like glycolysis and the TCA cycle, and can complete the pentose phosphate pathway non-oxidatively from D-ribose-5-phosphate. It undergoes complete amino acid biosynthesis for alanine, cysteine, aspartic acid, glutamic acid, glycine, glutamine, serine, threonine, tyrosine, and possibly lysine; all other amino acids must be incorporated from prey cells. It can undergo complete de novo nucleotide biosynthesis for dCTP and dTTP, and possibly for dATP and dGTP using inosine as a starting compound. Fatty acids can also be completely degraded and metabolized, presumably into acetyl-coA for use in the TCA cycle (3).
B. exovorus also contains cytochrome C within its electron transport chain, but it lacks catalase, superoxide dismutase, or peroxides needed to break down oxidative radicals (3).
Culture Conditions:
For laboratory study, one method of culturing involves growing B. exovorus on a double-layer agar with minimal medium, with lawn of prey bacteria grown for an optimal 24 hours before plating B. exovorus (1). B. exovorus will grow as plaques on that bacterial lawn in 2-6 days, leaving the visible empty husks of the prey cells behind (2). (This can be differentiated from plaques caused by bacteriophages, which appear in only 24 hours and do not typically continue growth in size.)
Another method involved growing B. exovorus in a liquid medium using 1/10-strength yeast extract-peptone medium supplemented with calcium, although a dilute nutrient broth medium can also be used. HM buffer is added to media, made of HEPES, CaCl2, and MgSO4, and adjusted to optimal pH 7.8 (6). While ideal growth occurs around 30 °C, B. exovorus can be kept in a sterile tube at 4 °C for 1 month or stored long-term with 25% (w/v) glycerol at -80 °C (5).
To purify a culture, a plaque solution can be filtered, emulsified, serially diluted, and transferred to a new plate or nutrient broth for incubation. After repeating this process several times, a culture can be considered pure, having filtered out any unwanted organisms (2).
Waste and By-Products:
No waste, by-products, or volatile compounds appear to have been explicitly discussed in the literature regarding B. exovorus, although the ghost cells left behind after consuming the cytoplasmic contents of a host cell could be considered a waste product (3). B. exovorus also sheds its flagellum once entering the growth phase, so perhaps that could be considered a waste product as well (3).
Ecology / Pathology
Ecology:
While bdellovibrios have been found ubiquitously in rivers, soil, compost, and wastewater (2), B. exovorus in particular was isolated from raw sewage, preying on other bacteria that flourish in that environment (5). Because it significantly reduces levels of bacterial populations without completely eradicating them, B. exovorus is believed to have some regulatory function within its ecosystem, keeping other bacterial growth under control (3,6).
Attempts have been made to introduce B. exovorus into living organisms as well to control the growth of potential pathogens, although they have been typically unable to establish a lasting place in an organism's microbiome without a significant and consistent amount of prey bacteria (3).
Pathology:
Like other members of the genus bdellovibrio, the species B. exovorus is not pathogenic to humans, and only predates on specific bacterial cells. It is incapable of attacking eukaryotic cells, as well as gram-positive bacterial cells. It is, however, inherently resistant to many commonly-used antibiotics, including ampicillin, chloramphenicol, ciprofloxacin, and kanamycin (3,6).
Current Research
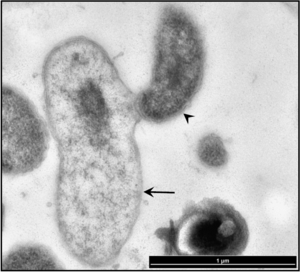
Currently, B. exovorus strain FFRS-5 (the first strain known to attack a bacterium other than C. crescentus) is being studied as an effective treatment for the newly-emerging opportunistic pathogen S. maltophilia, which although it increases plant growth and in the root nodules of other plants, and kills plant pathogens, it is inherently very resistant to antibiotics, heavy metals, and polycyclic aromatic hydrocarbons (3). Because of this, hospital patients with compromised immune systems are susceptible to infection from S. maltophilia. Symptoms of infection include pneumonia, bacteremia, urinary tract and soft tissue infections, eye infections, endocarditis, and meningitis. The bacterium is also being increasingly found alongside P. aeruginosa in cases of cystic fibrosis. While it is not very virulent, S. maltophilia can colonize rapidly, forming biofilms in harsh environments and thus causing infections (4).
In order to treat infection, neither antibiotics nor phage therapy have proven effective (phages are too strain-specific and potentially transfer resistance genes to their host), leaving bacterial predators as a potential method of treatment. Because they are unable to attack eukaryotic cells, and have surprisingly high antibiotic levels themselves, they make an excellent candidate for use as a live antimicrobial. In combination with the antibiotic ciprofloxacin, B. exovorus was able to significantly lower biofilm masses produced by S. maltophilia in both laboratory and clinical settings as recently as 2016 (3,4).
It is suggested that future studies could use a mixture of a number of Bdellovibrio and like organisms to attack and reduce infections caused by many other drug-resistant bacteria like P. aeruginosa, B. cenocepacia, E. coli, and species of Acinetobacter. This could be used to keep open wounds free of infection, and prevent the growth of biofilms on catheters and stents. Perhaps it could even be used in aerosol form to treat biofilms of pathogenic bacteria in patients with upper respiratory infections and cystic fibrosis (3)!
Another possible future study involves "tricking" B. exovorus into attacking gram-positive bacteria as well. B. exovorus has already been observed reversibly attaching to gram-positive cell walls; it could be possible to manipulate the surface receptors to send the intermediary signals necessary to begin the growth phase (3).
References
[1] Bdellovibrio exovorus JSS [internet]. University of California- Integrated Microbial Genomes & Microbial Samples. [cited 2017 Feb 13] Availible from https://img.jgi.doe.gov/cgi-bin/m/main.cgi?section=TaxonDetail&page=taxonDetail&taxon_oid=2541047022
[2] Brenner DJ. 2005. Bergey's manual of systematic bacteriology. Vol. 2. The proteobacteria. Part C. The alpha-, beta-, delta-, and epsilonproteobacteria. Staley JT, editor. New York (NY): Springer. p.1040-1053.
[3] Chanyi RM. 2014. 2285: Cell Biology of the Entry of Bdellovibrio and Like Organisms [dissertation]. [London (ON)]: University of Western Ontario.
[4] Chanyi RM, Koval SF, and Brooke JS. Stenotrophomonas maltophilia biofilm reduction by Bdellovibrio exovorus. Environmental Microbiology Reports [Internet]. 2016 [cited 31 Mar 2017]; 8:343–351.
[5] Koval SF, Hynes SH, Flannagan RS, Pasternak Z, Davidov Y, Jurkevitch E. Bdellovibrio exovorus sp. nov., a novel predator of Caulobacter crescentus. Int. J. Syst. Evol. Microbiol [Internet]. 2013 [cited 13 Feb 2017];(63):146–151.
[6] Pasternak Z, Njagi M, Shani Y, Chanyi R, Rotem O, Lurie-Weinberger MN, Koval S, Pietrokovski S, Gophna U, Jurkevitch E. In and out: an analysis of epibiotic vs periplasmic bacterial predators. International Society for Microbial Ecology [Internet]. 2014 [cited 2 Apr 2017]; 8:625-635
Authored by Kip Pierce, a student of CJ Funk at John Brown University