Equine Development of Gut Microbiota: Difference between revisions
No edit summary |
No edit summary |
||
(27 intermediate revisions by the same user not shown) | |||
Line 1: | Line 1: | ||
[[Image:foal-horse-pony-baby-horse-preview.jpg|thumb|300px|right|A young horse, called a foal. Photo credit: [https://www.pickpik.com/foal-horse-pony-baby-horse-young-colt-62688]]] | [[Image:foal-horse-pony-baby-horse-preview.jpg|thumb|300px|right|A young horse, called a foal. Photo credit: [https://www.pickpik.com/foal-horse-pony-baby-horse-young-colt-62688]]] | ||
<b>By Mira Allen </b> <br> | <b>By Mira Allen </b> <br> | ||
<br>Topic: development of and mature gut microbiota in equine<br><br> | <br>Topic: development of and mature gut microbiota in equine<br><br> | ||
<br> | <br> | ||
==Introduction and Importance== | ==Introduction and Importance== | ||
Much like in humans, the equine gut microbiome is considered by some to be akin to an organ system due to the significant role that it plays in digestion [10]. In addition, an intact microbiota stimulates mucus production and immunity, produces short-chain fatty acids, maintains antigen tolerance, prevents colonization of harmful organisms, and improves dendrite function[ | Much like in humans, the equine gut microbiome is considered by some to be akin to an organ system due to the significant role that it plays in digestion<ref name=a>[https://journals.plos.org/plosone/article?id=10.1371/journal.pone.0216211 De La Torre et al. "Utilizing the fecal microbiota to understand foal gut transitions from birth to weaning." 2019. PLoS ONE 14(4):e0216211.]</ref>. In addition, an intact microbiota stimulates mucus production and immunity, produces short-chain fatty acids, maintains antigen tolerance, prevents colonization of harmful organisms, and improves dendrite function<ref name=b>[https://doi.org/10.3390/ani14050758 Boucher et al. "Current Understanding of Equine Gut Dysbiosis and Microbiota Manipulation Techniques: Comparison with Current Knowledge in Other Species." 2024. Animals 14(5):758.]</ref>. | ||
<br> | <br> | ||
In utero, horses' intestinal tracts are close to sterile. Soon after birth, however, microbial colonization skyrockets. Colonization of the gut with bacteria that can digest an adult diet, including roughage, lasts about 60 days [1 | In utero, horses' intestinal tracts are close to sterile. Soon after birth, however, microbial colonization skyrockets. Colonization of the gut with bacteria that can digest an adult diet, including roughage, lasts about 60 days<ref name=a/><ref name=c>[https://doi.org/10.1038%2Fs41598-019-50563-9 Lindenberg et al. "Development of the equine gut microbiota." 2019. Scientific Reports 9(1):14427.]</ref>. Proper colonization is incredibly important as improper microbial content can result in dysbiosis, which can lead to inflammation and metabolic disease<ref name=c/><ref name=d>[https://doi.org/10.1186/s42523-019-0013-3 Kauter et al. "The gut microbiome of horses: current research on equine enteral microbiota and future perspectives." 2019. Animal Microbiome 1(14).]</ref>. Equine hind-gut microbiota enable nutritional optimization from an otherwise nutrient-poor foraging diet via the fermentation of plant material<ref name=d/>. The initial colonization, stabilization, and then weaning period as a foal transfers to solid food are important milestones in establishing the microbial composition of the gut<ref name=c/>. Most equine gut bacteria live in the colon, specifically in the caecum<ref name=d/>. These bacteria degrade otherwise indigestible forage<ref name=d/>. The equine gut is composed of two main sections: <br> | ||
<b>Upper/fore gut</b><br> | <b>Upper/fore gut</b><br> | ||
This area is made up of the stomach, jejunum, and ileum | This area is made up of the stomach, jejunum, and ileum<ref name=d/>. There is increased microbiota variation and turnover as food is processed here first<ref name=d/>. <br> | ||
<b>Lower/hind gut </b><br> | <b>Lower/hind gut </b><br> | ||
This area is made up of the anaerobic caecum and colon | This area is made up of the anaerobic caecum and colon<ref name=b/><ref name=d/>. The microbiota is more stable here<ref name=d/>. In this section, bacteria ferment structural carbohydrates, allowing energy to be extracted from forage<ref name=b/>. <br> | ||
<br> | <br> | ||
==Gut Colonization: Birth to Maturity== | ==Gut Colonization: Birth to Maturity== | ||
The stages of microbiome colonization in mammals are as follows | The stages of microbiome colonization in mammals are as follows<ref name=b/><ref name=c/>:<br> | ||
- Initial colonization<br> | -Initial colonization<br> | ||
- Microbiota stabilization<br> | -Microbiota stabilization<br> | ||
- Weaning (in foals: between 4 and 10 months)<br> | -Weaning (in foals: between 4 and 10 months)<br> | ||
- Transfer to solid foods (occurs gradually)<br> | -Transfer to solid foods (occurs gradually)<br> | ||
<br> | <br> | ||
While these are generally applicable in mammals, it has been demonstrated that the foal gut microbiome closely resembles its mother's around 60 days of age | [[Image:Microbiome acquisition.jpg|thumb|500px|right|Factors affecting equine microbial colonization. Photo credit: [https://www.ncbi.nlm.nih.gov/pmc/articles/PMC4315782/]]] | ||
While these are generally applicable in mammals, it has been demonstrated that the foal gut microbiome closely resembles its mother's around 60 days of age<ref name=c/>, although weaning has been shown in some cases to have an impact (though this may be due to stress)<ref name=b/>. This means that their microbiome development occurs more rapidly than in most other mammals. A foal’s mother begins to influence its microbiome in utero by circulating microbial metabolites, but the birthing process has a larger impact on colonization<ref name=c/><ref name=e>[https://doi.org/10.1038/s41598-019-57003-8 Husso et al. "The composition of the perinatal intestinal microbiota in horse." 2020. Scientific Reports 10:441.]</ref>. Foals have bacteria present in their system immediately after birth. Their microbiome is then diversified and refined via environmental contact and the consumption of mare's milk, manure, and eventually solid food<ref name=c/>. The first week of life is a critical time as foals are very susceptible to infection and disease<ref name=e/>. Diarrhea is common in this period and can be associated with a microbial imbalance<ref name=e/>. <br> | |||
Due to the low permeability of the placenta to proteins, foals are born without circulating immunoglobulins [ | Due to the low permeability of the placenta to proteins, foals are born without circulating immunoglobulins<ref name=f>[https://doi.org/10.1093/af/vfad003 Reiter A. S. and Reed S. A. "Lactation in horses" 2023. Animal Frontiers 13(3):103-7.]</ref>. Thus, their immune system is weak until passive immunity can be transferred from the mother via immunoglobulins in the colostrum<ref name=f/>. Colostrum is the first milk produced following the birth of the foal, and its consumption is incredibly important in the initial microbial colonization stage. Colostrum is nutrient rich and provides the foal with amino acids, proteins, immunological factors, and antioxidants<ref name=f/>. This is vital to the foal’s growth, development, and immunocompetence and immune development<ref name=f/>. The transition from colostrum to mature milk production begins around 2 days post-parturition and lasts several weeks<ref name=f/>. <br> | ||
Immunoglobulins gained from colostrum protect the foal from environmental pathogens and make up nearly 60% of this milk's protein content | Immunoglobulins gained from colostrum protect the foal from environmental pathogens and make up nearly 60% of this milk's protein content<ref name=f/>. They are present in colostrum at much higher concentrations than mature milk, and immunity is transferred most significantly to the foal only in the first twelve hours of life<ref name=f/>. Immunoglobulin G occurs at high concentrations in colostrum, with immunoglobulin A at present at lower ones. Mature mare’s milk, on the other hand, contains mostly Immunoglobulin A<ref name=f/>. Also present in equine milk are lysozyme and lactoferrin proteins<ref name=a/><ref name=f/>. These possess antimicrobial properties to protect foals from bacterial infection, and may aid in proper gut microbiome establishment, including <I>lactobacillus</I> and <I>bifidobacterium </I><ref name=f/>. | ||
<br> | <br> | ||
Before 60 days old, gut microbiota composition is transient and dynamic. Research | Before 60 days old, gut microbiota composition is transient and dynamic. Research<ref name=c/><ref name=e/> has shown that change in similarity between individual foals and foals and adults occurs in these stages:<br> | ||
Day 0: relatively diverse bacterial colonization likely inherited from the foal's dam (mother) and the environment <br> | Day 0: relatively diverse bacterial colonization likely inherited from the foal's dam (mother) and the environment <br> | ||
Day 1: somewhat decreased microbiota diversity <br> | Day 1: somewhat decreased microbiota diversity <br> | ||
Line 55: | Line 49: | ||
<br> | <br> | ||
A decrease in microbiome diversity has been demonstrated as horses age, which have been speculatively attributed to changes in diet and/or deteriorating dental quality, digestion efficiency, and energy requirements | A decrease in microbiome diversity has been demonstrated as horses age, which have been speculatively attributed to changes in diet and/or deteriorating dental quality, digestion efficiency, and energy requirements<ref name=b/>. | ||
<br> | <br> | ||
==Species Composition== | ==Species Composition== | ||
Over 1,000 species of bacteria have been identified thus far in the equine GI tract | Over 1,000 species of bacteria have been identified thus far in the equine GI tract<ref name=b/>. The easiest and most common method of species identification is via fecal sample. The core adult equine microbiome that has so far been documented to include:<br> | ||
- <b>Bacteroidetes</b> (one of the most abundant phyla) | -<b>Bacteroidetes</b> (one of the most abundant phyla)<ref name=d/> including: <br> | ||
- <I>Bacteroides uniformis</I> | -<I>Bacteroides uniformis</I><ref name=c/><br> | ||
- <I>Bacteroides fragilis</I> | -<I>Bacteroides fragilis</I><ref name=c/><br> | ||
- <I>Parabacteroides</I> | -<I>Parabacteroides</I><ref name=c/><br> | ||
- <I>Butyricimonas</I> | -<I>Butyricimonas</I><ref name=c/><br> | ||
<br> | <br> | ||
<b>- Firmicutes</b> (largest and one of the most abundant phyla | <b>-Firmicutes</b> (largest and one of the most abundant phyla, including clostridia and bacilli)<ref name=d/><br> | ||
- <I>Clostridiales</I> (part of the intestinal core microbiome in all mammals) | -<I>Clostridiales</I> (part of the intestinal core microbiome in all mammals)<ref name=d/><br> | ||
-<I>Lactobacillus mucosae</I><ref name=c/><br> | |||
- <I>Lactobacillus mucosae</I> | -<I>Blautia producta</I><ref name=c/><br> | ||
- <I>Blautia producta</I> | -<I>Streptococcus</I><ref name=c/><br> | ||
- <I>Streptococcus</I> | -<I>Ruminococcaceae</I> (small percentage, hindgut)<ref name=d/><br> | ||
- <I>Ruminococcaceae</I> (small percentage, hindgut) | -<I>Fibrobacteraceae</I> (small percentage, hindgut)<ref name=d/><br> | ||
- <I>Fibrobacteraceae</I> (small percentage, hindgut) | |||
<br> | <br> | ||
<I>Ruminococcaceae</I> and <I>Fibrobacteraceae</I> are involved in fibrous plant wall degradation and are an integral species in gut health | <I>Ruminococcaceae</I> and <I>Fibrobacteraceae</I> are involved in fibrous plant wall degradation and are an integral species in gut health<ref name=a/><ref name=d/>. They also produce anti-inflammatory and immune-modulating short chain fatty acids<ref name=b/>. Some gut Clostridia are necessary for regular processes including physiology, metabolism, and immunity<ref name=g>[https://doi.org/10.1186/1757-4749-5-23 Lopetuso et al. "Commensal Clostridia: leading players in the maintenance of gut homeostasis." 2013. Sci Rep 5:23.]</ref>, but high levels may cause inflammation<ref name=b/>. <br> | ||
<br> | <br> | ||
- <b>Proteobacteria </b> (second largest phylum, driven by environment uptake)(common in upper GIT, most in ileum) may play a role in nitrogen fixation, but overabundance can cause inflammation, sometimes colic. | -<b>Proteobacteria </b> (second largest phylum, driven by environment uptake)(common in upper GIT, most in ileum) may play a role in nitrogen fixation, but overabundance can cause inflammation, sometimes colic<ref name=d/>.<br> | ||
- <I>Enterobacteriales</I> | -<I>Enterobacteriales</I><ref name=d/><ref name=e/><br> | ||
- <I>Pseudomonadales</I> | -<I>Pseudomonadales</I><ref name=d/><br> | ||
<br> | <br> | ||
- <b>Verrucomicrobia </b>(third largest phylum, present in the caecum, small colon, and rectum) | -<b>Verrucomicrobia </b>(third largest phylum, present in the caecum, small colon, and rectum)<ref name=d/>(assist in hindgut fermentation)<ref name=a/> <br> | ||
- <I>Akkermansia</I> (mucin degrading, maintains mucin layer integrity, decreases bowel inflammation) | -<I>Akkermansia</I> (mucin degrading, maintains mucin layer integrity, decreases bowel inflammation)<ref name=d/><br> | ||
<br> | <br> | ||
- <b> Spirochaetes </b><br> | -<b>Spirochaetes </b><br> | ||
- Spirochaetaceae | -<I>Spirochaetaceae</I><ref name=b/><br> | ||
- Lachnospiraceae (produce protective butyrate via fermentation) | -<I>Lachnospiraceae</I> (produce protective butyrate via fermentation, are protective of colonocytes)<ref name=b/><br> | ||
Also present in the hindgut | Also present in the hindgut<ref name=d/>:<br> | ||
- Protozoa | -Protozoa <br> | ||
- Fungi | -Fungi <br> | ||
- Yeast | -Yeast <br> | ||
- Archaea | -Archaea <br> | ||
- Methanogenic archaea (metabolize H2 and CO2 to methane; support degradation of cellulolytic bacteria) | -Methanogenic archaea (metabolize H2 and CO2 to methane; support degradation of cellulolytic bacteria)<br> | ||
<br> | <br> | ||
The foal gut microbiome has been recorded to contain mostly Firmicutes | The foal gut microbiome has been recorded to contain mostly Firmicutes<ref name=d/>. Between 2 and 30 days, Verrucomicrobia are the main species<ref name=d/>. | ||
After 24 hours, the gut contains mostly Escherichia/Shigella such as <I>E. coli</I>, Bacteroides, and Firmicutes, with species including <I>Clostridium, Streptococcus, Enterococcus, </I>and <I>Klebsiella </I>[3]. Some beneficial<I>E. coli</I> release bacteriocins which prevent the growth of other species<ref name=b/>. This is beneficial as it prevents pathogenic <I>E. coli</I> growth<ref name=b/>. Bacteria from <I>Deltaproteobacteria</I>, <I>Desulfovibrionaceae</I>, and <I>Erysipelotrichaceae</I> are more commonly seen in diets high in fat<ref name=j>[https://pubmed.ncbi.nlm.nih.gov/19706296/ Hildebrandt et al. "High-fat diet determines the composition of the murine gut microbiome independently of obesity." 2009. Gastroenterology 137(5):1716-24.e1-2.]</ref><ref name=k>[https://pubmed.ncbi.nlm.nih.gov/25835047/ Koleva et al. "The infant gut microbiome: evidence for obesity risk and dietary intervention." 2015. Nutrients 7(4):2237-60.]</ref>, as one would see in foals drinking exclusively milk<ref name=a/>. <br> | |||
[[Image:comparison graph.png|thumb|600px|left|LEfSe comparison showing relative abundance of bacterial species between 1 and 60 days of age. Photo credit: [https://doi.org/10.1371/journal.pone.0216211.g007]]] | |||
By day 7, the most abundant group is | By day 7, the most abundant group is Firmicutues, with Bacteroides and Fusobacteria having increased in abundance decreased Proteobacteria<ref name=e/>. The increase in Bacteroides specifically has been associated with the introduction of solid food<ref name=l>[https://pubmed.ncbi.nlm.nih.gov/25651996/ Rodriguez et al. "The composition of the gut microbiota throughout life, with an emphasis on early life." 2015. Microb Ecol Health Dis 26:26050.]</ref> as they play a part in complex protein and sugar digestion<ref name=m>[https://pubmed.ncbi.nlm.nih.gov/24463512/ Larsbrink et al. "A discrete genetic locus confers xyloglucan metabolism in select human gut Bacteroidetes." 2014. Nature 506(7489).]</ref>, which at this age foals have likely been increasingly exposed to<ref name=a/>. Bacteroides were most abundant, present in all foals along with <I>Fusobacterium, Tyzzerella, Streptococcus </I>and <I>Lactobacillus </I><ref name=e/>. <I>Enterobacteriaceae, Erysipelotrichaceae,</I> and <I>Peptostreptococcaceae</I> have also been documented at high concentrations in day 7 samples<ref name=a/>. The increase in <I>Enterobacteriaceae</I> at this age is likely due to repeated exposure to fecal matter<ref name=a/>. Both of the families <I>Lachnospiraceae</I> and <I>Ruminococcaceae</I> were present from day 7 until weaning, and have been hypothesized to aid in the breakdown of complex carbohydrates<ref name=a/>. | ||
<br> | <br> | ||
These findings may differ between individuals depending on their housing (ie. stall or turnout), access to adult foods from birth (ie. grain, grass), and geographic location. The factors of domestication and housing type interfere with the sharing of microbiomes between horses. Domesticated horses have lower <I> Clostridia phascolarctobacterium,</I> which produce short chain fatty acid propionate<ref name=d/>. Furthermore, non-domestic horses have higher levels of <I> Methanocorpusculum </I> archaea, which are methane producers and may increase the carbohydrate degrading activity of cellulolytic bacteria<ref name=d/>. <br> | |||
<br> | Similarly, horses fed mainly grain as opposed to forage were found to have lower levels of<I> Fibrobacter</I> and <I>Ruminococcaceae</I> and higher levels of<I> Lachnospiraceae, Bacteroidetes,</I> and members of the Bacillus-Lactobacillus-Streptococcus group<ref name=b/>. It has even been suggested that the "gut-brain" relationship discussed so often in humans may also exist in horses. High-fiber diets in horses have been associated with calmer, more investigative behavior compared to increased anxiety seen in horses consuming high-starch diets<ref name=b/>. <br> | ||
==An Unhappy Microbiome: Effects of Change and Dysbiosis== | ==An Unhappy Microbiome: Effects of Change and Dysbiosis== | ||
Harmful changes to the equine gut microbiome may occur as a result of: <br> | Harmful changes to the equine gut microbiome may occur as a result of: <br> | ||
- Stress | - Stress<ref name=b/><br> | ||
- Transport | - Transport<ref name=d/><br> | ||
- Fasting | - Fasting<ref name=d/><br> | ||
- Abrupt dietary change | - Abrupt dietary change<ref name=b/><br> | ||
- Antibiotic administration | - Antibiotic administration<ref name=b/><br> | ||
<br> | <br> | ||
An imbalance resulting in a deficiency of one or multiple species can result in the overgrowth of another. Decreased richness and diversity are common signifiers of dysbiosis | An imbalance resulting in a deficiency of one or multiple species can result in the overgrowth of another. Decreased richness and diversity are common signifiers of dysbiosis<ref name=b/>. It can take up to 25 days to bring the biome back to baseline, but effects can last longer<ref name=d/>. Often associated with dysbiosis are increased levels of Fusobacteria, Clostridia, and Proteobacteria along with decreased levels of <I>Ruminococcaceae </I> and <I>Lachnospiraceae</I><ref name=b/>. <I> Escherichia </I> and <I> Fusobacterium</I> are known to be associated with diarrhea<ref name=b/>. | ||
Often associated with dysbiosis are increased levels of Fusobacteria, Clostridia, and Proteobacteria along with decreased levels of Ruminococcaceae and Lachnospiraceae | |||
Transporting horses has shown to be associated with decreased levels several bacteria including Clostridia, Bacteroidetes, and Streptococci, but this response can also be partially attributed to stress | Transporting horses has shown to be associated with decreased levels several bacteria including Clostridia, Bacteroidetes, and Streptococci, but this response can also be partially attributed to stress<ref name=b/>. General stress has been associated with a decrease in <I> Bacteroidaceae</I> <ref name=b/> and an increase in <I> Succinivibrionaceae</I><ref name=b/>. <br> | ||
<b>Antibiotics </b><br> | <b>Antibiotics </b><br> | ||
Antibiotic administration has a significant effect on the microbiome. Improper use can lead to antibiotic-induced colitis, the overgrowth of pathogens such as <I>Clostridioides</I>, and an overall decrease in microbiome richness and diversity | Antibiotic administration has a significant effect on the microbiome. Improper use can lead to antibiotic-induced colitis, the overgrowth of pathogens such as <I>Clostridioides</I>, and an overall decrease in microbiome richness and diversity<ref name=b/>. Different drugs have different effects on specific groups of bacteria<ref name=b/>. Maintaining a diverse microbiota is important because species redundancy increases resilience, so if one species is harmed, the overall function of the system will be less impacted<ref name=b/>. <br> | ||
Although the microbiota largely returns to normal by a month post-treatment, some species may be affected for longer | Although the microbiota largely returns to normal by a month post-treatment, some species may be affected for longer<ref name=b/>. Other drugs that may impact microbiome composition include NSAID's, helminths, and anthelmintics [24]. The NSAID's phenylbutazone and firocoxib have been demonstrated to decrease concentrations of Firmicutes, <I>Clostridiaceae, Ruminococcaceae, </I>and <I>Lachnospiraceae</I><ref name=b/>. <br> | ||
<b>Foal Heat Diarrhea</b><br> | <b>Foal Heat Diarrhea</b><br> | ||
Foals between 4 and 14 days are at an increased risk for developing mild diarrhea [ | Foals between 4 and 14 days are at an increased risk for developing mild diarrhea<ref name=p>[https://www.merckvetmanual.com/digestive-system/diarrheal-diseases-in-foals/foal-heat-diarrhea Stewart A. J. "Foal Heat Diarrhea." 2022. Merck Veterinary Manual.]</ref>. Foals with a decreased microbiome diversity (specifically decreased <I>Lachnospiraceae</I> and <I>Ruminococcaceae</I>) are at a higher risk<ref name=d/>. In 7 day old foals with diarrhea, increased <I>Enterobacteriaceae</I> and <I> Alcaligenceae </I>concentrations have been found<ref name=a/>. Additionally, higher levels of lactose digesting <I> Bifidobacteriaceae</I> were found in these foals in comparison to their older counterparts, likely due to their milk consumption at this age<ref name=a/>. Heathy 7 day old foals, in comparison, had increased <I>Actinomycetales</I>, specifically in the family <I>Micrococcaceae</I><ref name=a/>. The presence of some <I>Actinobacteria</I> may aid in the maintenance of a healthy gut they aid in immune response to pathogens<ref name=q>[https://pubmed.ncbi.nlm.nih.gov/17804669/ Ventura et al. "Genomics of Actinobacteria: tracing the evolutionary history of an ancient phylum." 2007. Microbiol Mol Biol Rev 71(3):495-548.]</ref>. | ||
< | |||
<br> | <br> | ||
<b>Septicemia</b><br> | <b>Septicemia</b><br> | ||
Sepsis is a disease that occurs when the body improperly reacts to infection. Septic shock causes organ failure due to lack of blood flow and tissue oxygenation. In foals, known causes include failure to transfer immunoglobulins from colostrum | Sepsis is a disease that occurs when the body improperly reacts to infection. Septic shock causes organ failure due to lack of blood flow and tissue oxygenation. In foals, known causes include failure to transfer immunoglobulins from colostrum<ref name=e/>, issues with gestation or birth, and/or environmental contamination<ref name=r>[https://www.ncbi.nlm.nih.gov/pmc/articles/PMC9889700/ Gomez et al. "The fecal bacterial microbiota of healthy and sick newborn foals." 2023. J Vet Intern Med 37(1):315-322.]</ref>. It can take up to four weeks of intensive care to recover from sepsis, but the recovery rate is still between 50-81%<ref name=s>[https://www.merckvetmanual.com/management-and-nutrition/management-of-the-neonate/sepsis-in-foals Bedenice D. "Sepsis in Foals." 2022. Merck Veterinary Manual.]</ref>. Sepsis may be in part due to translocation of bacteria from the gut to the bloodstream, which is at increased risk in the first 24 hours of life<ref name=r/>. Healthy foals have been found to harbor greater numbers of <I>Enterococcus </I>(Firmicutes) and<I> Pasteurellaceae</I> (Proteobacteria), while septic foals had more Lactobacillus (Firmicutes), Facklamia (Firmicutes), and bacteria of the family Sphingobactarieaceae (Bacteroidetes)<ref name=r/>. | ||
Healthy foals have been found to harbor greater numbers of Enterococcus (Firmicutes) and Pasteurellaceae (Proteobacteria), while septic foals had more Lactobacillus (Firmicutes), Facklamia (Firmicutes), and bacteria of the family Sphingobactarieaceae (Bacteroidetes) | |||
<br> | <br> | ||
Line 154: | Line 141: | ||
<b> Colitis</b><br> | <b> Colitis</b><br> | ||
Colitis is a blanket term for various diseases including clostridial enterocolitis, salmonellosis, and <i> Aeromonas </i> colitis [ | Colitis is a blanket term for various diseases including clostridial enterocolitis, salmonellosis, and <i> Aeromonas </i> colitis<ref name=t>[https://www.merckvetmanual.com/digestive-system/miscellaneous-intestinal-diseases-in-horses/colitis-x-in-horses?query=enterocolitis%20horse Stewart A. J. "Colitis-X in Horses." 2022. Merck Veterinary Manual.]</ref>. These bacteria can be difficult to culture and present similarly, so they are generally all classified as colitis. The disease is characterized by acute or long-term inflammation of the gut mucosa in the large bowel (cecum, colon) and presents as a sudden onset of watery diarrhea that causes dehydration and often rapid death<ref name=d/><ref name=t/>. A general decrease in microbial diversity, specifically in Firmicutes and Lactobacillus have been observed, but some conflicting reports do exist<ref name=b/>. | ||
<br> | <br> | ||
Colitis cases can also be triggered by: <br> | Colitis cases can also be triggered by<ref name=b/><ref name=b/>: <br> | ||
- Bacterial infections (including <I>Salmonella, Clostridioides Difficile, Clostridium Perfringens,</I> and<I> Neorickettsia Risticii</I>) | - Bacterial infections (including <I>Salmonella, Clostridioides Difficile, Clostridium Perfringens,</I> and<I> Neorickettsia Risticii</I>)<br> | ||
- Equine Coronavius | - Equine Coronavius<br> | ||
- Parasite infections | - Parasite infections<br> | ||
- Antimicrobial treatment (including Penicillin, Cephalosporins, Fluoroquinolones, and Trimethoprim-sulfadiazine) | - Antimicrobial treatment (including Penicillin, Cephalosporins, Fluoroquinolones, and Trimethoprim-sulfadiazine) <br> | ||
- Stress | - Stress<ref name=t/><br> | ||
<br> | <br> | ||
<b> Colic </b><br> | <b> Colic </b><br> | ||
GI issues and stomach pain in horses are collectively known as colic. Colic cases range from mild to severe, but overall are a serious concern for horse-owners as there is only a 63% survival rate | GI issues and stomach pain in horses are collectively known as colic. Colic cases range from mild to severe, but overall are a serious concern for horse-owners as there is only a 63% survival rate<ref name=d/>. Horses colic easily because gas and fluid can only move through the digestive tract in one direction<ref name=u>[https://www.merckvetmanual.com/digestive-system/colic-in-horses/overview-of-colic-in-horses Moore J. N. "Overview of Colic in Horses." 2021. Merck Veterinary Manual.]</ref>. Thus, conditions which upset the stomach or flow of material can cause result in inflammation, severe pain, and even stomach rupture<ref name=u/>. | ||
<br> | <br> | ||
Causes of colic include: <br> | Causes of colic include<ref name=d/><ref name=u/>: <br> | ||
- Sand ingestion | - Sand ingestion<br> | ||
- Stress | - Stress<br> | ||
- Changes in feeding, which can cause a rapid change in microbiome composition | - Changes in feeding, which can cause a rapid change in microbiome composition<br> | ||
- Inflammation of the intestine, including that cause by enteritis or colitis | - Inflammation of the intestine, including that cause by enteritis or colitis<br> | ||
- Inflammation of the abdominal cavity lining, including that caused by peritonitis | - Inflammation of the abdominal cavity lining, including that caused by peritonitis<br> | ||
<br> | <br> | ||
Under normal circumstances, the equine GI tract's mucosal barrier prevents enteric bacteria and associated endotoxins from leaving the intestinal lumen | Under normal circumstances, the equine GI tract's mucosal barrier prevents enteric bacteria and associated endotoxins from leaving the intestinal lumen where they exist in high quantities<ref name=u/>. In colic cases, disruption to the mucosal lining caused by inflammation or damage can allow bacteria and associated components to migrate to the perinatal cavity, from where they can enter the bloodstream<ref name=u/>. Once circulating, these components, specifically flagellin and endotoxins, become targets of leukocytes, which initiate inflammatory responses<ref name=u/>. This can include abnormal coagulation, hypotension, reduced blood flow, depression, and fever<ref name=u/>. Collectively, this response is known as endotoxemia, and treating it is vital<ref name=u/>. Several solutions exist, including treatment with medication, antibodies, and even Polymyxin B, which at low quantities binds endotoxins without causing toxic effects itself<ref name=u/>. | ||
< | |||
<br> | <br> | ||
<b> Laminitis </b><br> | <b> Laminitis </b><br> | ||
[[Image:laminitis photo.png|thumb|300px|right|A healthy vs a laminitic hoof. Photo credit: [http://www.southwestequine.com.au/understanding-laminitis-fouunder/]]] | |||
Laminitis (also known as founder) is a disease in which the supporting tissue connected to the coffin bone (the lowermost bone in a horse's hoof) fails to secure to the hoof wall which can result in the bone twisting or dropping, sometimes completely through the bottom of the hoof. This is incredibly painful, causes severe lameness, and is incurable in severe cases. There are several causes of laminitis, one being sepsis associated [ | Laminitis (also known as founder) is a disease in which the supporting tissue connected to the coffin bone (the lowermost bone in a horse's hoof) fails to secure to the hoof wall, which can result in the bone twisting or dropping, sometimes completely through the bottom of the hoof. This is incredibly painful, causes severe lameness, and is incurable in severe cases. There are several causes of laminitis, one being sepsis associated<ref name=v>[https://www.merckvetmanual.com/musculoskeletal-system/lameness-in-horses/laminitis-in-horses?query=laminitis Belknap J. K. "Laminitis in Horses." 2015. Merck Veterinary Manual.]</ref>. Diseases causing sepsis that have also been associated with laminitis are usually caused by gram negative bacteria<ref name=v/>. Increased levels of<I> Verrucomicrobia</I> has been associated with chronic laminitis<ref name=b/>. A high starch diet can cause an increase of bacteria that produce high levels of lactic acid, such as <I> Streptococcus</I> species<ref name=b/><ref name=d/>. This changes the gut pH, allowing more lactic acid-producing species to grow, furthering dysbiosis<ref name=b/>. These changes can cause lactic acidosis, which can lead to laminitis<ref name=d/>. Other causes of sepsis related laminitis include retained fetal membranes, colic, and enterocolitis<ref name=v/><br> | ||
<b> Peritonitis </b><br> | <b> Peritonitis </b><br> | ||
Peritonitis is a condition that occurs in horses when the inner lining of the abdominal cavity, called the peritoneum, becomes inflamed [ | Peritonitis is a condition that occurs in horses when the inner lining of the abdominal cavity, called the peritoneum, becomes inflamed<ref name=x>[https://www.merckvetmanual.com/generalized-conditions/peritonitis/peritonitis-in-animals Wittek T. "Peritonitis in Animals." 2020. Merck Veterinary Manual.]</ref>. It has several causes, including infection which spreads via the bloodstream<ref name=x/>. With GI tract perforation, a mixed population of bacteria are usually found at the infection site, but other tissue perforation and infection are more often associated with <I> E. coli, Streptococcus, Staphylococcus, Klebsiella, Salmonella, Enterobacter, </I> or <I>Pseudomonas</I>, to name a few<ref name=x/>. The condition is treated with antimicrobials<ref name=x/>. | ||
<br> | <br> | ||
Line 192: | Line 179: | ||
<b> Anesthesia </b><br> | <b> Anesthesia </b><br> | ||
Anesthesia is another documented cause of sudden microbiome change, and can further cause enrichment of | Anesthesia is another documented cause of sudden microbiome change, and can further cause enrichment of<ref name=d/>: <br> | ||
- Anaerostipes <br> | -<I>Anaerostipes</I> <br> | ||
- Ethanoligenens <br> | -<I>Ethanoligenens</I> <br> | ||
- Enterococcus ( | -<I>Enterococcus</I> (Firmicutes) <br> | ||
- Ruminococcus ( | -<I>Ruminococcus</I> (Firmicutes) <br> | ||
<b>Other Diseases</b><br> | <b>Other Diseases</b><br> | ||
Asthma: The occurrence of equine asthma may be associated with dysbiosis, as the microbial adaptation of the gut has been shown to differ between horses with and without asthma | Asthma: The occurrence of equine asthma may be associated with dysbiosis, as the microbial adaptation of the gut has been shown to differ between horses with and without asthma<ref name=b/>.<br> | ||
Equine Metabolic Syndrome: The microbiota composition of horses diagnosed with metabolic syndrome has been demonstrated to differ from healthy animals, suggesting a microbial role in the disease | Equine Metabolic Syndrome: The microbiota composition of horses diagnosed with metabolic syndrome has been demonstrated to differ from healthy animals, suggesting a microbial role in the disease<ref name=b/>. | ||
Obesity: It has been suggested that the gut microbiome may play a role in equine obesity via production of inflammatory cytokines | Obesity: It has been suggested that the gut microbiome may play a role in equine obesity via production of inflammatory cytokines<ref name=b/>. | ||
<br> | <br> | ||
==Fixing Dysbiosis: Microbiome Manipulation== | ==Fixing Dysbiosis: Microbiome Manipulation== | ||
Some strategies do exist to aid in the maintenance microbiome health and stability. | Some strategies do exist to aid in the maintenance microbiome health and stability. | ||
[[Image:diet.webp|thumb|300px|right|The effects of diet on the microbiome. Photo credit: [https://doi.org/10.1186/s42523-019-0013-3]]] | |||
<b>Diet</b><br> | <b>Diet</b><br> | ||
As previously mentioned, a diet high in forage can promote beneficial bacteria and calm behavior | As previously mentioned, a diet high in forage can promote beneficial bacteria and calm behavior<ref name=b/>. Furthermore, keeping a consistent diet improves microbiome stability, but research in this area is limited due to the difficulty of experimentation<ref name=b/>. | ||
<br> | <br> | ||
<b>Probiotics</b><br> | <b>Probiotics</b><br> | ||
Probiotics are microorganisms that are given to a host with the intention of providing some benefit | Probiotics are microorganisms that are given to a host with the intention of providing some benefit<ref name=b/>. They may be given to prevent pathogen colonization, suppress inflammation, and to increase tight junction proteins to improve gut barrier function<ref name=b/>. <br> | ||
Effective probiotics must<ref name=b/><ref name=d/>: <br> | |||
-Survive in the acidic gut environment<br> | |||
-Possess some antimicrobial property against pathogens<br> | |||
- | -Adhere to mucus and epithelial cells well enough to colonize the gut <br> | ||
- | -In horses: establish in large colon (as many diseases occur here)<br> | ||
- | |||
- | |||
Putative beneficial probiotics for horses include<ref name=b/><ref name=d/>: <br> | |||
- <I>Lactobacillus</I><br> | |||
-<I> Enterococcus</I><br> | |||
-<I> Bacillus</I><br> | |||
- <I>Streptococcus</I><br> | |||
- <I>Bifidobacterium</I><br> | |||
- <I>Saccharomyces cerevisiae</i><br> | |||
- <I>Saccharomyces boulardii</i><br> | |||
There may be more potential for their use in the developmental period (0-60 days) as probiotic organisms may have an easier time establishing when there are fewer resident species | There may be more potential for their use in the developmental period (0-60 days) as probiotic organisms may have an easier time establishing when there are fewer resident species<ref name=b/><ref name=d/>. The evidence of their efficiency is weak thus far, however, in all age groups studied<ref name=b/>. | ||
<i>Saccharomyces cerevisiae</i> and <I>Saccharomyces boulardii</i> may be the most promising equine probiotic candidates thus far | <i>Saccharomyces cerevisiae</i> and <I>Saccharomyces boulardii</i> may be the most promising equine probiotic candidates thus far<ref name=b/>. These are two species of yeast that may be able to provide bacteria substrate on which to grow and to generate proteases to degrade toxins [24]. Preliminary results show that these species can likely survive in the intestinal tract, but may not be able to colonize it permanently<ref name=b/>.<br> | ||
<b>Prebiotics</b><br> | <b>Prebiotics</b><br> | ||
Prebiotics are fibers administered with the intent of them becoming substrates for beneficial microbes to colonize | Prebiotics are fibers administered with the intent of them becoming substrates for beneficial microbes to colonize<ref name=b/>. These are often oligosaccharides, including fruit-oligosaccharides or manna-oligosaccharides<ref name=b/>. They may also select against colonization of pathogens, but the effectiveness of this property is not guaranteed and may vary depending on the pre-existing microbial species composition<ref name=b/>. Once again, effectiveness is uncertain and there have been limited studies conducted to evaluate potential<ref name=b/>. | ||
<br> | <br> | ||
<b>Fecal Microbiota Transplantation</b><br> | <b>Fecal Microbiota Transplantation</b><br> | ||
Fecal Microbiota Transplantation allows healthy species of microbes to be transferred from a donor to a recipient via feces suspension | Fecal Microbiota Transplantation allows healthy species of microbes to be transferred from a donor to a recipient via feces suspension<ref name=b/>. This treatment can be effective in humans but, similarly to pre- and probiotic use, shows conflicting results in horses<ref name=b/>. | ||
<br> | <br> | ||
==Conclusion== | ==Conclusion== | ||
Overall, horses have relatively low gut microbe diversity | Overall, horses have relatively low gut microbe diversity<ref name=b/>. This could be partially due to domestication and/or housing practices, but it is a likely contributor to their susceptibility to GI upset and associated disease<ref name=b/>. Due to its observed role in maintaining beneficial microbial species in the gut, a fiber-based diet seems to be optimal for maintaining equine health<ref name=b/>. Overall, this is an emerging area of research with potential for many more studies to be conducted, both to improve baseline knowledge of the equine microbiota and to improve veterinary practices and disease management. | ||
Latest revision as of 01:02, 15 April 2024
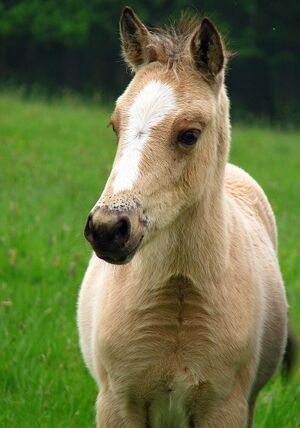
By Mira Allen
Topic: development of and mature gut microbiota in equine
Introduction and Importance
Much like in humans, the equine gut microbiome is considered by some to be akin to an organ system due to the significant role that it plays in digestion[1]. In addition, an intact microbiota stimulates mucus production and immunity, produces short-chain fatty acids, maintains antigen tolerance, prevents colonization of harmful organisms, and improves dendrite function[2].
In utero, horses' intestinal tracts are close to sterile. Soon after birth, however, microbial colonization skyrockets. Colonization of the gut with bacteria that can digest an adult diet, including roughage, lasts about 60 days[1][3]. Proper colonization is incredibly important as improper microbial content can result in dysbiosis, which can lead to inflammation and metabolic disease[3][4]. Equine hind-gut microbiota enable nutritional optimization from an otherwise nutrient-poor foraging diet via the fermentation of plant material[4]. The initial colonization, stabilization, and then weaning period as a foal transfers to solid food are important milestones in establishing the microbial composition of the gut[3]. Most equine gut bacteria live in the colon, specifically in the caecum[4]. These bacteria degrade otherwise indigestible forage[4]. The equine gut is composed of two main sections:
Upper/fore gut
This area is made up of the stomach, jejunum, and ileum[4]. There is increased microbiota variation and turnover as food is processed here first[4].
Lower/hind gut
This area is made up of the anaerobic caecum and colon[2][4]. The microbiota is more stable here[4]. In this section, bacteria ferment structural carbohydrates, allowing energy to be extracted from forage[2].
Gut Colonization: Birth to Maturity
The stages of microbiome colonization in mammals are as follows[2][3]:
-Initial colonization
-Microbiota stabilization
-Weaning (in foals: between 4 and 10 months)
-Transfer to solid foods (occurs gradually)
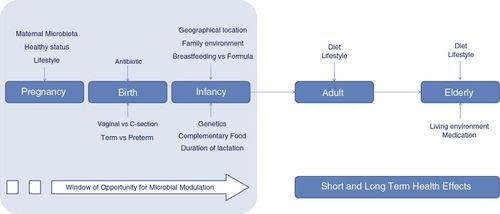
While these are generally applicable in mammals, it has been demonstrated that the foal gut microbiome closely resembles its mother's around 60 days of age[3], although weaning has been shown in some cases to have an impact (though this may be due to stress)[2]. This means that their microbiome development occurs more rapidly than in most other mammals. A foal’s mother begins to influence its microbiome in utero by circulating microbial metabolites, but the birthing process has a larger impact on colonization[3][5]. Foals have bacteria present in their system immediately after birth. Their microbiome is then diversified and refined via environmental contact and the consumption of mare's milk, manure, and eventually solid food[3]. The first week of life is a critical time as foals are very susceptible to infection and disease[5]. Diarrhea is common in this period and can be associated with a microbial imbalance[5].
Due to the low permeability of the placenta to proteins, foals are born without circulating immunoglobulins[6]. Thus, their immune system is weak until passive immunity can be transferred from the mother via immunoglobulins in the colostrum[6]. Colostrum is the first milk produced following the birth of the foal, and its consumption is incredibly important in the initial microbial colonization stage. Colostrum is nutrient rich and provides the foal with amino acids, proteins, immunological factors, and antioxidants[6]. This is vital to the foal’s growth, development, and immunocompetence and immune development[6]. The transition from colostrum to mature milk production begins around 2 days post-parturition and lasts several weeks[6].
Immunoglobulins gained from colostrum protect the foal from environmental pathogens and make up nearly 60% of this milk's protein content[6]. They are present in colostrum at much higher concentrations than mature milk, and immunity is transferred most significantly to the foal only in the first twelve hours of life[6]. Immunoglobulin G occurs at high concentrations in colostrum, with immunoglobulin A at present at lower ones. Mature mare’s milk, on the other hand, contains mostly Immunoglobulin A[6]. Also present in equine milk are lysozyme and lactoferrin proteins[1][6]. These possess antimicrobial properties to protect foals from bacterial infection, and may aid in proper gut microbiome establishment, including lactobacillus and bifidobacterium [6].
Before 60 days old, gut microbiota composition is transient and dynamic. Research[3][5] has shown that change in similarity between individual foals and foals and adults occurs in these stages:
Day 0: relatively diverse bacterial colonization likely inherited from the foal's dam (mother) and the environment
Day 1: somewhat decreased microbiota diversity
Day 7: large variation between the microbiota of individual foals
Day 20: more consistent between foals, but different from adults
Day 60: very similar to adult composition
A decrease in microbiome diversity has been demonstrated as horses age, which have been speculatively attributed to changes in diet and/or deteriorating dental quality, digestion efficiency, and energy requirements[2].
Species Composition
Over 1,000 species of bacteria have been identified thus far in the equine GI tract[2]. The easiest and most common method of species identification is via fecal sample. The core adult equine microbiome that has so far been documented to include:
-Bacteroidetes (one of the most abundant phyla)[4] including:
-Bacteroides uniformis[3]
-Bacteroides fragilis[3]
-Parabacteroides[3]
-Butyricimonas[3]
-Firmicutes (largest and one of the most abundant phyla, including clostridia and bacilli)[4]
-Clostridiales (part of the intestinal core microbiome in all mammals)[4]
-Lactobacillus mucosae[3]
-Blautia producta[3]
-Streptococcus[3]
-Ruminococcaceae (small percentage, hindgut)[4]
-Fibrobacteraceae (small percentage, hindgut)[4]
Ruminococcaceae and Fibrobacteraceae are involved in fibrous plant wall degradation and are an integral species in gut health[1][4]. They also produce anti-inflammatory and immune-modulating short chain fatty acids[2]. Some gut Clostridia are necessary for regular processes including physiology, metabolism, and immunity[7], but high levels may cause inflammation[2].
-Proteobacteria (second largest phylum, driven by environment uptake)(common in upper GIT, most in ileum) may play a role in nitrogen fixation, but overabundance can cause inflammation, sometimes colic[4].
-Enterobacteriales[4][5]
-Pseudomonadales[4]
-Verrucomicrobia (third largest phylum, present in the caecum, small colon, and rectum)[4](assist in hindgut fermentation)[1]
-Akkermansia (mucin degrading, maintains mucin layer integrity, decreases bowel inflammation)[4]
-Spirochaetes
-Spirochaetaceae[2]
-Lachnospiraceae (produce protective butyrate via fermentation, are protective of colonocytes)[2]
Also present in the hindgut[4]:
-Protozoa
-Fungi
-Yeast
-Archaea
-Methanogenic archaea (metabolize H2 and CO2 to methane; support degradation of cellulolytic bacteria)
The foal gut microbiome has been recorded to contain mostly Firmicutes[4]. Between 2 and 30 days, Verrucomicrobia are the main species[4].
After 24 hours, the gut contains mostly Escherichia/Shigella such as E. coli, Bacteroides, and Firmicutes, with species including Clostridium, Streptococcus, Enterococcus, and Klebsiella [3]. Some beneficialE. coli release bacteriocins which prevent the growth of other species[2]. This is beneficial as it prevents pathogenic E. coli growth[2]. Bacteria from Deltaproteobacteria, Desulfovibrionaceae, and Erysipelotrichaceae are more commonly seen in diets high in fat[8][9], as one would see in foals drinking exclusively milk[1].

By day 7, the most abundant group is Firmicutues, with Bacteroides and Fusobacteria having increased in abundance decreased Proteobacteria[5]. The increase in Bacteroides specifically has been associated with the introduction of solid food[10] as they play a part in complex protein and sugar digestion[11], which at this age foals have likely been increasingly exposed to[1]. Bacteroides were most abundant, present in all foals along with Fusobacterium, Tyzzerella, Streptococcus and Lactobacillus [5]. Enterobacteriaceae, Erysipelotrichaceae, and Peptostreptococcaceae have also been documented at high concentrations in day 7 samples[1]. The increase in Enterobacteriaceae at this age is likely due to repeated exposure to fecal matter[1]. Both of the families Lachnospiraceae and Ruminococcaceae were present from day 7 until weaning, and have been hypothesized to aid in the breakdown of complex carbohydrates[1].
These findings may differ between individuals depending on their housing (ie. stall or turnout), access to adult foods from birth (ie. grain, grass), and geographic location. The factors of domestication and housing type interfere with the sharing of microbiomes between horses. Domesticated horses have lower Clostridia phascolarctobacterium, which produce short chain fatty acid propionate[4]. Furthermore, non-domestic horses have higher levels of Methanocorpusculum archaea, which are methane producers and may increase the carbohydrate degrading activity of cellulolytic bacteria[4].
Similarly, horses fed mainly grain as opposed to forage were found to have lower levels of Fibrobacter and Ruminococcaceae and higher levels of Lachnospiraceae, Bacteroidetes, and members of the Bacillus-Lactobacillus-Streptococcus group[2]. It has even been suggested that the "gut-brain" relationship discussed so often in humans may also exist in horses. High-fiber diets in horses have been associated with calmer, more investigative behavior compared to increased anxiety seen in horses consuming high-starch diets[2].
An Unhappy Microbiome: Effects of Change and Dysbiosis
Harmful changes to the equine gut microbiome may occur as a result of:
- Stress[2]
- Transport[4]
- Fasting[4]
- Abrupt dietary change[2]
- Antibiotic administration[2]
An imbalance resulting in a deficiency of one or multiple species can result in the overgrowth of another. Decreased richness and diversity are common signifiers of dysbiosis[2]. It can take up to 25 days to bring the biome back to baseline, but effects can last longer[4]. Often associated with dysbiosis are increased levels of Fusobacteria, Clostridia, and Proteobacteria along with decreased levels of Ruminococcaceae and Lachnospiraceae[2]. Escherichia and Fusobacterium are known to be associated with diarrhea[2].
Transporting horses has shown to be associated with decreased levels several bacteria including Clostridia, Bacteroidetes, and Streptococci, but this response can also be partially attributed to stress[2]. General stress has been associated with a decrease in Bacteroidaceae [2] and an increase in Succinivibrionaceae[2].
Antibiotics
Antibiotic administration has a significant effect on the microbiome. Improper use can lead to antibiotic-induced colitis, the overgrowth of pathogens such as Clostridioides, and an overall decrease in microbiome richness and diversity[2]. Different drugs have different effects on specific groups of bacteria[2]. Maintaining a diverse microbiota is important because species redundancy increases resilience, so if one species is harmed, the overall function of the system will be less impacted[2].
Although the microbiota largely returns to normal by a month post-treatment, some species may be affected for longer[2]. Other drugs that may impact microbiome composition include NSAID's, helminths, and anthelmintics [24]. The NSAID's phenylbutazone and firocoxib have been demonstrated to decrease concentrations of Firmicutes, Clostridiaceae, Ruminococcaceae, and Lachnospiraceae[2].
Foal Heat Diarrhea
Foals between 4 and 14 days are at an increased risk for developing mild diarrhea[12]. Foals with a decreased microbiome diversity (specifically decreased Lachnospiraceae and Ruminococcaceae) are at a higher risk[4]. In 7 day old foals with diarrhea, increased Enterobacteriaceae and Alcaligenceae concentrations have been found[1]. Additionally, higher levels of lactose digesting Bifidobacteriaceae were found in these foals in comparison to their older counterparts, likely due to their milk consumption at this age[1]. Heathy 7 day old foals, in comparison, had increased Actinomycetales, specifically in the family Micrococcaceae[1]. The presence of some Actinobacteria may aid in the maintenance of a healthy gut they aid in immune response to pathogens[13].
Septicemia
Sepsis is a disease that occurs when the body improperly reacts to infection. Septic shock causes organ failure due to lack of blood flow and tissue oxygenation. In foals, known causes include failure to transfer immunoglobulins from colostrum[5], issues with gestation or birth, and/or environmental contamination[14]. It can take up to four weeks of intensive care to recover from sepsis, but the recovery rate is still between 50-81%[15]. Sepsis may be in part due to translocation of bacteria from the gut to the bloodstream, which is at increased risk in the first 24 hours of life[14]. Healthy foals have been found to harbor greater numbers of Enterococcus (Firmicutes) and Pasteurellaceae (Proteobacteria), while septic foals had more Lactobacillus (Firmicutes), Facklamia (Firmicutes), and bacteria of the family Sphingobactarieaceae (Bacteroidetes)[14].
Colitis
Colitis is a blanket term for various diseases including clostridial enterocolitis, salmonellosis, and Aeromonas colitis[16]. These bacteria can be difficult to culture and present similarly, so they are generally all classified as colitis. The disease is characterized by acute or long-term inflammation of the gut mucosa in the large bowel (cecum, colon) and presents as a sudden onset of watery diarrhea that causes dehydration and often rapid death[4][16]. A general decrease in microbial diversity, specifically in Firmicutes and Lactobacillus have been observed, but some conflicting reports do exist[2].
Colitis cases can also be triggered by[2][2]:
- Bacterial infections (including Salmonella, Clostridioides Difficile, Clostridium Perfringens, and Neorickettsia Risticii)
- Equine Coronavius
- Parasite infections
- Antimicrobial treatment (including Penicillin, Cephalosporins, Fluoroquinolones, and Trimethoprim-sulfadiazine)
- Stress[16]
Colic
GI issues and stomach pain in horses are collectively known as colic. Colic cases range from mild to severe, but overall are a serious concern for horse-owners as there is only a 63% survival rate[4]. Horses colic easily because gas and fluid can only move through the digestive tract in one direction[17]. Thus, conditions which upset the stomach or flow of material can cause result in inflammation, severe pain, and even stomach rupture[17].
Causes of colic include[4][17]:
- Sand ingestion
- Stress
- Changes in feeding, which can cause a rapid change in microbiome composition
- Inflammation of the intestine, including that cause by enteritis or colitis
- Inflammation of the abdominal cavity lining, including that caused by peritonitis
Under normal circumstances, the equine GI tract's mucosal barrier prevents enteric bacteria and associated endotoxins from leaving the intestinal lumen where they exist in high quantities[17]. In colic cases, disruption to the mucosal lining caused by inflammation or damage can allow bacteria and associated components to migrate to the perinatal cavity, from where they can enter the bloodstream[17]. Once circulating, these components, specifically flagellin and endotoxins, become targets of leukocytes, which initiate inflammatory responses[17]. This can include abnormal coagulation, hypotension, reduced blood flow, depression, and fever[17]. Collectively, this response is known as endotoxemia, and treating it is vital[17]. Several solutions exist, including treatment with medication, antibodies, and even Polymyxin B, which at low quantities binds endotoxins without causing toxic effects itself[17].
Laminitis
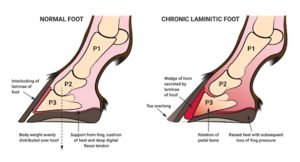
Laminitis (also known as founder) is a disease in which the supporting tissue connected to the coffin bone (the lowermost bone in a horse's hoof) fails to secure to the hoof wall, which can result in the bone twisting or dropping, sometimes completely through the bottom of the hoof. This is incredibly painful, causes severe lameness, and is incurable in severe cases. There are several causes of laminitis, one being sepsis associated[18]. Diseases causing sepsis that have also been associated with laminitis are usually caused by gram negative bacteria[18]. Increased levels of Verrucomicrobia has been associated with chronic laminitis[2]. A high starch diet can cause an increase of bacteria that produce high levels of lactic acid, such as Streptococcus species[2][4]. This changes the gut pH, allowing more lactic acid-producing species to grow, furthering dysbiosis[2]. These changes can cause lactic acidosis, which can lead to laminitis[4]. Other causes of sepsis related laminitis include retained fetal membranes, colic, and enterocolitis[18]
Peritonitis
Peritonitis is a condition that occurs in horses when the inner lining of the abdominal cavity, called the peritoneum, becomes inflamed[19]. It has several causes, including infection which spreads via the bloodstream[19]. With GI tract perforation, a mixed population of bacteria are usually found at the infection site, but other tissue perforation and infection are more often associated with E. coli, Streptococcus, Staphylococcus, Klebsiella, Salmonella, Enterobacter, or Pseudomonas, to name a few[19]. The condition is treated with antimicrobials[19].
Anesthesia
Anesthesia is another documented cause of sudden microbiome change, and can further cause enrichment of[4]:
-Anaerostipes
-Ethanoligenens
-Enterococcus (Firmicutes)
-Ruminococcus (Firmicutes)
Other Diseases
Asthma: The occurrence of equine asthma may be associated with dysbiosis, as the microbial adaptation of the gut has been shown to differ between horses with and without asthma[2].
Equine Metabolic Syndrome: The microbiota composition of horses diagnosed with metabolic syndrome has been demonstrated to differ from healthy animals, suggesting a microbial role in the disease[2].
Obesity: It has been suggested that the gut microbiome may play a role in equine obesity via production of inflammatory cytokines[2].
Fixing Dysbiosis: Microbiome Manipulation
Some strategies do exist to aid in the maintenance microbiome health and stability.
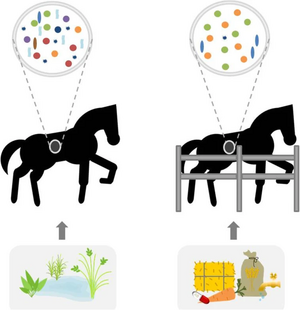
Diet
As previously mentioned, a diet high in forage can promote beneficial bacteria and calm behavior[2]. Furthermore, keeping a consistent diet improves microbiome stability, but research in this area is limited due to the difficulty of experimentation[2].
Probiotics
Probiotics are microorganisms that are given to a host with the intention of providing some benefit[2]. They may be given to prevent pathogen colonization, suppress inflammation, and to increase tight junction proteins to improve gut barrier function[2].
Effective probiotics must[2][4]:
-Survive in the acidic gut environment
-Possess some antimicrobial property against pathogens
-Adhere to mucus and epithelial cells well enough to colonize the gut
-In horses: establish in large colon (as many diseases occur here)
Putative beneficial probiotics for horses include[2][4]:
- Lactobacillus
- Enterococcus
- Bacillus
- Streptococcus
- Bifidobacterium
- Saccharomyces cerevisiae
- Saccharomyces boulardii
There may be more potential for their use in the developmental period (0-60 days) as probiotic organisms may have an easier time establishing when there are fewer resident species[2][4]. The evidence of their efficiency is weak thus far, however, in all age groups studied[2].
Saccharomyces cerevisiae and Saccharomyces boulardii may be the most promising equine probiotic candidates thus far[2]. These are two species of yeast that may be able to provide bacteria substrate on which to grow and to generate proteases to degrade toxins [24]. Preliminary results show that these species can likely survive in the intestinal tract, but may not be able to colonize it permanently[2].
Prebiotics
Prebiotics are fibers administered with the intent of them becoming substrates for beneficial microbes to colonize[2]. These are often oligosaccharides, including fruit-oligosaccharides or manna-oligosaccharides[2]. They may also select against colonization of pathogens, but the effectiveness of this property is not guaranteed and may vary depending on the pre-existing microbial species composition[2]. Once again, effectiveness is uncertain and there have been limited studies conducted to evaluate potential[2].
Fecal Microbiota Transplantation
Fecal Microbiota Transplantation allows healthy species of microbes to be transferred from a donor to a recipient via feces suspension[2]. This treatment can be effective in humans but, similarly to pre- and probiotic use, shows conflicting results in horses[2].
Conclusion
Overall, horses have relatively low gut microbe diversity[2]. This could be partially due to domestication and/or housing practices, but it is a likely contributor to their susceptibility to GI upset and associated disease[2]. Due to its observed role in maintaining beneficial microbial species in the gut, a fiber-based diet seems to be optimal for maintaining equine health[2]. Overall, this is an emerging area of research with potential for many more studies to be conducted, both to improve baseline knowledge of the equine microbiota and to improve veterinary practices and disease management.
References
- ↑ 1.00 1.01 1.02 1.03 1.04 1.05 1.06 1.07 1.08 1.09 1.10 1.11 1.12 De La Torre et al. "Utilizing the fecal microbiota to understand foal gut transitions from birth to weaning." 2019. PLoS ONE 14(4):e0216211.
- ↑ 2.00 2.01 2.02 2.03 2.04 2.05 2.06 2.07 2.08 2.09 2.10 2.11 2.12 2.13 2.14 2.15 2.16 2.17 2.18 2.19 2.20 2.21 2.22 2.23 2.24 2.25 2.26 2.27 2.28 2.29 2.30 2.31 2.32 2.33 2.34 2.35 2.36 2.37 2.38 2.39 2.40 2.41 2.42 2.43 2.44 2.45 2.46 2.47 2.48 2.49 2.50 2.51 2.52 2.53 2.54 2.55 2.56 Boucher et al. "Current Understanding of Equine Gut Dysbiosis and Microbiota Manipulation Techniques: Comparison with Current Knowledge in Other Species." 2024. Animals 14(5):758.
- ↑ 3.00 3.01 3.02 3.03 3.04 3.05 3.06 3.07 3.08 3.09 3.10 3.11 3.12 3.13 3.14 Lindenberg et al. "Development of the equine gut microbiota." 2019. Scientific Reports 9(1):14427.
- ↑ 4.00 4.01 4.02 4.03 4.04 4.05 4.06 4.07 4.08 4.09 4.10 4.11 4.12 4.13 4.14 4.15 4.16 4.17 4.18 4.19 4.20 4.21 4.22 4.23 4.24 4.25 4.26 4.27 4.28 4.29 4.30 4.31 4.32 4.33 4.34 4.35 4.36 Kauter et al. "The gut microbiome of horses: current research on equine enteral microbiota and future perspectives." 2019. Animal Microbiome 1(14).
- ↑ 5.0 5.1 5.2 5.3 5.4 5.5 5.6 5.7 Husso et al. "The composition of the perinatal intestinal microbiota in horse." 2020. Scientific Reports 10:441.
- ↑ 6.0 6.1 6.2 6.3 6.4 6.5 6.6 6.7 6.8 6.9 Reiter A. S. and Reed S. A. "Lactation in horses" 2023. Animal Frontiers 13(3):103-7.
- ↑ Lopetuso et al. "Commensal Clostridia: leading players in the maintenance of gut homeostasis." 2013. Sci Rep 5:23.
- ↑ Hildebrandt et al. "High-fat diet determines the composition of the murine gut microbiome independently of obesity." 2009. Gastroenterology 137(5):1716-24.e1-2.
- ↑ Koleva et al. "The infant gut microbiome: evidence for obesity risk and dietary intervention." 2015. Nutrients 7(4):2237-60.
- ↑ Rodriguez et al. "The composition of the gut microbiota throughout life, with an emphasis on early life." 2015. Microb Ecol Health Dis 26:26050.
- ↑ Larsbrink et al. "A discrete genetic locus confers xyloglucan metabolism in select human gut Bacteroidetes." 2014. Nature 506(7489).
- ↑ Stewart A. J. "Foal Heat Diarrhea." 2022. Merck Veterinary Manual.
- ↑ Ventura et al. "Genomics of Actinobacteria: tracing the evolutionary history of an ancient phylum." 2007. Microbiol Mol Biol Rev 71(3):495-548.
- ↑ 14.0 14.1 14.2 Gomez et al. "The fecal bacterial microbiota of healthy and sick newborn foals." 2023. J Vet Intern Med 37(1):315-322.
- ↑ Bedenice D. "Sepsis in Foals." 2022. Merck Veterinary Manual.
- ↑ 16.0 16.1 16.2 Stewart A. J. "Colitis-X in Horses." 2022. Merck Veterinary Manual.
- ↑ 17.0 17.1 17.2 17.3 17.4 17.5 17.6 17.7 17.8 Moore J. N. "Overview of Colic in Horses." 2021. Merck Veterinary Manual.
- ↑ 18.0 18.1 18.2 Belknap J. K. "Laminitis in Horses." 2015. Merck Veterinary Manual.
- ↑ 19.0 19.1 19.2 19.3 Wittek T. "Peritonitis in Animals." 2020. Merck Veterinary Manual.
Authored for BIOL 238 Microbiology, taught by Joan Slonczewski,at Kenyon College,2024