Gas gangrene (Clostridial myonecrosis): Difference between revisions
No edit summary |
No edit summary |
||
Line 27: | Line 27: | ||
==Theta-Toxin== | ==Theta-Toxin== | ||
Theta (θ) toxin, also known as perfringolysin O (PFO) is a toxin secreted by Clostridium perfringens that is a pore-forming chlosterol-dependent cytolysin. PFO is expressed in almost all Clostridium perfrigens strains and plays a role in gas gangrene advancement. The genes for producing PFO and alpha-toxin are located on the chromosome rather than on the plasmid (such as beta, epsilon and iota). Although PFO is widely encoded by most C. perfrigens strains, some food-poisoning strains do not have the PFO gene.<ref name=ver>1. Verherstraeten S, Goossens E, Valgaeren B, et al.<i> Perfringolysin O: The Underrated Clostridium perfringens Toxin?</i> Popoff MR, ed. Toxins. 2015;7(5):1702-1721. doi:10.3390/toxins7051702. | Theta (θ) toxin, also known as perfringolysin O (PFO) is a toxin secreted by Clostridium perfringens that is a pore-forming chlosterol-dependent cytolysin. PFO is expressed in almost all Clostridium perfrigens strains and plays a role in gas gangrene advancement. The genes for producing PFO and alpha-toxin are located on the chromosome rather than on the plasmid (such as beta, epsilon and iota). Although PFO is widely encoded by most C. perfrigens strains, some food-poisoning strains do not have the PFO gene.<ref name=ver>1. Verherstraeten S, Goossens E, Valgaeren B, et al.<i> Perfringolysin O: The Underrated Clostridium perfringens Toxin?</i> Popoff MR, ed. Toxins. 2015;7(5):1702-1721. doi:10.3390/toxins7051702. | ||
</ref> PFO is an average sized protein (500 amino acids) that varies in protein structure and gene location. This effects virulence of the bacteria and is likely explained by recombination.<ref name=ver></ref> PFO also contains a signal peptide that helps secretion through general secretory pathway (GSP). When traversing the cell membrane, it is cleaved. In solution at high concentrations, PFO likely forms high concentrations with a head-to-tail structure. PFO monomers contain four domains that are mostly β-barrels. PFO is dependent on cholesterol as a cellular receptor for pore formation. Cholesterol is ubiquitous in mammalian membranes. | </ref> | ||
[[Image:pore.png|thumb|300px|right|Mechanism of pore formation by PFO on eukaryotic lipid bilayer. Association between lipid bilayer and PFO is greatest on edges of the membrane, where cholesterol is most accessible. [http://www.mdpi.com/2072-6651/7/5/1702/htm].]] | |||
PFO is an average sized protein (500 amino acids) that varies in protein structure and gene location. This effects virulence of the bacteria and is likely explained by recombination.<ref name=ver></ref> PFO also contains a signal peptide that helps secretion through general secretory pathway (GSP). When traversing the cell membrane, it is cleaved. In solution at high concentrations, PFO likely forms high concentrations with a head-to-tail structure. PFO monomers contain four domains that are mostly β-barrels. PFO is dependent on cholesterol as a cellular receptor for pore formation. Cholesterol is ubiquitous in mammalian membranes. | |||
The mechanism of PFO cytolysis of mammalian cells begins with the loops of domain four attaching to the plasma membrane of the eukaryotic cell. One of the loops contains a conserved undecapeptide which helps anchor the PFO to the membrane of the cell and initiate necessary conformational changes to allow the β-barrel to enter the membrane of the eukaryotic cells. Binding capacity of the PFO may be dependent on the accessibility of the cholesterol in the cellular membrane, which is dependent on the spacing of the phospholipid groups and the saturation level of the cholesterol-phospholipid acyl chain.<ref name=ver></ref> Thickness of the phospholipid bilayer may affect the efficiency of pore formation by PFO. When domain four binds to the eukaryotic cellular membrane, the PFO molecule undergoes structural changes and allows association with another monomer. The prepore structure then forms. The prepore structure is made of oligomerized monomers that have not inserted the β-barrel into the membrane yet. Two amphipathic transmembrane β-hairpins are inserted into the membrane by each monomer. Every single monomer does same action in synchronization. | The mechanism of PFO cytolysis of mammalian cells begins with the loops of domain four attaching to the plasma membrane of the eukaryotic cell. One of the loops contains a conserved undecapeptide which helps anchor the PFO to the membrane of the cell and initiate necessary conformational changes to allow the β-barrel to enter the membrane of the eukaryotic cells. Binding capacity of the PFO may be dependent on the accessibility of the cholesterol in the cellular membrane, which is dependent on the spacing of the phospholipid groups and the saturation level of the cholesterol-phospholipid acyl chain.<ref name=ver></ref> Thickness of the phospholipid bilayer may affect the efficiency of pore formation by PFO. When domain four binds to the eukaryotic cellular membrane, the PFO molecule undergoes structural changes and allows association with another monomer. The prepore structure then forms. The prepore structure is made of oligomerized monomers that have not inserted the β-barrel into the membrane yet. Two amphipathic transmembrane β-hairpins are inserted into the membrane by each monomer. Every single monomer does same action in synchronization. | ||
==Alpha and Theta-Toxin Synergy in Immune Response== | ==Alpha and Theta-Toxin Synergy in Immune Response== | ||
Line 36: | Line 39: | ||
==Alpha-Toxin== | ==Alpha-Toxin== | ||
Alpha-toxin, or phospholipase C (PLC), degrades lecithin and sphingomyelin in eukaryotic cell membranes. Lecithin is any sort of yellow-brown fatty acid substances which are amphiphilic and sphingomyelin, a type of sphingolipid found in eukaryotic membranes.<ref name=obrien></ref> Alpha-toxin is uniquely an enzyme and also has contains two Zn2+ ions that are essential for catalysis.<ref name=titball></ref> The structure of alpha-toxin contains two domains with the N-terminal composed of α-helices and the C-terminal composed of β-sheets. The N-domain contains the phospholipase C active site.<ref name=titball></ref> The function of the C-domain is not fully understood, however recent studies suggest that the C-domain may be important for the interaction between alpha-toxin and membrane phospholipids through calcium ions that are coordinated by the amino acid residues.<ref name=titball></ref> There are structural variants of the alpha-toxin, individual to the bacterium. Structural differences usually do not affect the phospholipase C activity but may have biological significance. Some variations are more resistant to degradation.<ref name=titball></ref> | Alpha-toxin, or phospholipase C (PLC), degrades lecithin and sphingomyelin in eukaryotic cell membranes. Lecithin is any sort of yellow-brown fatty acid substances which are amphiphilic and sphingomyelin, a type of sphingolipid found in eukaryotic membranes.<ref name=obrien></ref> Alpha-toxin is uniquely an enzyme and also has contains two Zn2+ ions that are essential for catalysis.<ref name=titball></ref> The structure of alpha-toxin contains two domains with the N-terminal composed of α-helices and the C-terminal composed of β-sheets. The N-domain contains the phospholipase C active site.<ref name=titball></ref> | ||
[[Image:alphatox1.png|thumb|300px|right|Alpha-toxin shown interacting with lipid bilayer of eukaryotic membrane. Interactions with ions are shown, denoting the critical role of Ca2+ and Zn2+ in the function of alpha-toxin. [http://ac.els-cdn.com/S1075996499901919/1-s2.0-S1075996499901919-main.pdf?_tid=843ae610-28a7-11e7-a31e-00000aacb35e&acdnat=1493008740_a6dead265896beb6ba4f37cf4b555556 ].]] | |||
[[Image:alphatox2.png|thumb|300px|right|The structure of PFO. β-barrels are evident in the four separate domains. Domain 1 and 2 are purple, domain 3 is green and domain 4 is red. [http://www.mdpi.com/2072-6651/7/5/1702/htm].]] | |||
The function of the C-domain is not fully understood, however recent studies suggest that the C-domain may be important for the interaction between alpha-toxin and membrane phospholipids through calcium ions that are coordinated by the amino acid residues.<ref name=titball></ref> There are structural variants of the alpha-toxin, individual to the bacterium. Structural differences usually do not affect the phospholipase C activity but may have biological significance. Some variations are more resistant to degradation.<ref name=titball></ref> | |||
===Cell Damage=== | ===Cell Damage=== | ||
Cell Damage: | Cell Damage: | ||
Line 49: | Line 57: | ||
Kappa-toxin, like alpha-toxin, is also an enzyme. Kappa toxin digests collagen and collagen-related products and does not target other products such as albumin or hemoglobin. However, because collagen makes up about 25% of total protein content in the body, mostly as connective tissue, this enzyme is highly disruptive to bodily function.<ref name=louis>1. Louis D. S. Smith. (1979). Virulence Factors of Clostridium perfringens [with Discussion]. Reviews of Infectious Diseases, 1(2), 254-262. Retrieved from http://www.jstor.org/stable/4452312 | Kappa-toxin, like alpha-toxin, is also an enzyme. Kappa toxin digests collagen and collagen-related products and does not target other products such as albumin or hemoglobin. However, because collagen makes up about 25% of total protein content in the body, mostly as connective tissue, this enzyme is highly disruptive to bodily function.<ref name=louis>1. Louis D. S. Smith. (1979). Virulence Factors of Clostridium perfringens [with Discussion]. Reviews of Infectious Diseases, 1(2), 254-262. Retrieved from http://www.jstor.org/stable/4452312 | ||
</ref> Upon intravenous injection of kappa-toxin, the reticulin framework of tissue suffers extensive damage, up to hemorrhage into the lungs.<ref name=louis></ref> Injection into the muscle reduces tissue into a swampy, bloody mush. In gas gangrene, kappa-toxin is responsible for the reduction of muscle into pulp. Kappa-toxin also acts as a virulence factor by releasing amino acids that promote the further growth of C. perfrigens.<ref name=louis></ref> | </ref> Upon intravenous injection of kappa-toxin, the reticulin framework of tissue suffers extensive damage, up to hemorrhage into the lungs.<ref name=louis></ref> Injection into the muscle reduces tissue into a swampy, bloody mush. In gas gangrene, kappa-toxin is responsible for the reduction of muscle into pulp. Kappa-toxin also acts as a virulence factor by releasing amino acids that promote the further growth of C. perfrigens.<ref name=louis></ref> | ||
[[Image:kappatox1.jpeg|thumb|200px|right|Structure of alpha-toxin. Two separate domains are pictured with N- and C-domains and Zn2+ and possible Ca2+ ions. [http://ac.els-cdn.com/S1075996499901919/1-s2.0-S1075996499901919-main.pdf?_tid=843ae610-28a7-11e7-a31e-00000aacb35e&acdnat=1493008740_a6dead265896beb6ba4f37cf4b555556].]] | |||
==Vaccines and Antitoxins== | ==Vaccines and Antitoxins== | ||
Line 59: | Line 69: | ||
==Treatment== | ==Treatment== | ||
Treatment must be aggressive and timely. The “triangle” of treatment for gas gangrene is describes as: antibiotics, hyperbaric oxygen therapy, and surgery. High doses of antibiotics such as penicillin and clindamycin will be administered intravenously, even before proper diagnosis. Protein synthesis inhibitors such as rifampin or tetracycline are also effect because they inhibit the synthesis of exotoxins produced by Clostridium. Additional anti-microbial agents are recommended because nonclostridial bacterial are often found in gas gangrene cultures.<ref name=hoi>1. Hoi, H., MD, & Cunha, B. A., MD. (2017, February 09).<i> Gas Gangrene Treatment & Management.</i> Retrieved April 24, 2017, from http://emedicine.medscape.com/article/217943-treatment | Treatment must be aggressive and timely. The “triangle” of treatment for gas gangrene is describes as: antibiotics, hyperbaric oxygen therapy, and surgery. High doses of antibiotics such as penicillin and clindamycin will be administered intravenously, even before proper diagnosis. Protein synthesis inhibitors such as rifampin or tetracycline are also effect because they inhibit the synthesis of exotoxins produced by Clostridium. Additional anti-microbial agents are recommended because nonclostridial bacterial are often found in gas gangrene cultures.<ref name=hoi>1. Hoi, H., MD, & Cunha, B. A., MD. (2017, February 09).<i> Gas Gangrene Treatment & Management.</i> Retrieved April 24, 2017, from http://emedicine.medscape.com/article/217943-treatment | ||
</ref> All infected tissue will have to be removed, sometimes resulting in amputation. If wounds had previously healed, it is optimal to reopen and clean them. Debridement of necrotic tissue must be performed often under close watch until normal production of tissue resumes. Reconstructive surgery such as skin grafts may be used to help mitigate the loss of tissue. Prosthetics are an option for amputations. Sometimes, hyperbaric oxygen therapy will be used to help treat gas gangrene because the elevated oxygen content in the blood will help wound healing.<ref name=piet></ref> However, results for hyperbaric oxygen therapy are conflicting because there are few comparative studies. However, studies on animal models indicate that hyperbaric oxygen therapy severely restricts Clostridium, likely due to the increased oxygen content in tissue.<ref name=hoi></ref> The production of alpha-toxin by Clostridium is the main cause of the rapidly advancing tissue necrosis. A tissue partial pressure of oxygen of 250mmHg is needed to stop production of toxins, so early treatment and repetitive (more than two times) of hyperbaric oxygen is best.<ref name=bakker>1. Bakker, D. J. (2012). <i>Clostridial myonecrosis (gas gangrene).</i> Undersea & Hyperbaric Medicine, 39(3), 731. | </ref> All infected tissue will have to be removed, sometimes resulting in amputation. If wounds had previously healed, it is optimal to reopen and clean them. Debridement of necrotic tissue must be performed often under close watch until normal production of tissue resumes. [[Image:oxygen.png|thumb|300px|right|Use of hyperbaric oxygen therapy in wound healing of 53-year-old diabetic patient with spontaneous gas gangrene. Leg was preserved with use of hyperbaric oxygen. Healthy tissue growth can be seen last image. [http://www.ijps.org/article.asp?issn=0970-0358;year=2012;volume=45;issue=2;spage=316;epage=324;aulast=Bhutani].]] Reconstructive surgery such as skin grafts may be used to help mitigate the loss of tissue. Prosthetics are an option for amputations. Sometimes, hyperbaric oxygen therapy will be used to help treat gas gangrene because the elevated oxygen content in the blood will help wound healing.<ref name=piet></ref> However, results for hyperbaric oxygen therapy are conflicting because there are few comparative studies. However, studies on animal models indicate that hyperbaric oxygen therapy severely restricts Clostridium, likely due to the increased oxygen content in tissue.<ref name=hoi></ref> The production of alpha-toxin by Clostridium is the main cause of the rapidly advancing tissue necrosis. A tissue partial pressure of oxygen of 250mmHg is needed to stop production of toxins, so early treatment and repetitive (more than two times) of hyperbaric oxygen is best.<ref name=bakker>1. Bakker, D. J. (2012). <i>Clostridial myonecrosis (gas gangrene).</i> Undersea & Hyperbaric Medicine, 39(3), 731. | ||
</ref> | </ref> | ||
Major clinical studies have shown that the most mortality was achieved with initial conservative surgery and then rapid follow-up with hyperbaric oxygen therapy. When hyperbaric oxygen therapy is delayed, mortality rate steeply increases. Aggressive surgery is not recommended—it is better to wait until the line between dead tissue and healthy tissue is apparent before surgery.<ref name=bakker></ref> It is also important to begin hyperbaric oxygen therapy even before surgery—amputation rates drastically decrease without prompt, initial hyperbaric oxygen therapy. | Major clinical studies have shown that the most mortality was achieved with initial conservative surgery and then rapid follow-up with hyperbaric oxygen therapy. When hyperbaric oxygen therapy is delayed, mortality rate steeply increases. Aggressive surgery is not recommended—it is better to wait until the line between dead tissue and healthy tissue is apparent before surgery.<ref name=bakker></ref> It is also important to begin hyperbaric oxygen therapy even before surgery—amputation rates drastically decrease without prompt, initial hyperbaric oxygen therapy. | ||
==References== | ==References== | ||
<references /> | <references /> | ||
<br><br>Authored for BIOL 238 Microbiology, taught by [mailto:slonczewski@kenyon.edu Joan Slonczewski], 2017, [http://www.kenyon.edu/index.xml Kenyon College]. | <br><br>Authored for BIOL 238 Microbiology, taught by [mailto:slonczewski@kenyon.edu Joan Slonczewski], 2017, [http://www.kenyon.edu/index.xml Kenyon College]. |
Revision as of 02:40, 28 April 2017
Pathology

By Yiyi Ma
Clostridial myonecrosis (gas gangrene) is a necrotizing soft tissue infection that targets skeletal muscle. It is typically caused by Clostridium perfrigens, Clostridium septicum, and Clostridium novyi.[1] It is opportunistic infection that is extremely fast spreading and highly lethal. Clostridium is a genus of anaerobic bacteria, gram positive bacteria. Clostridium use anaerobic fermentation to produce hydrogen gas and carbon dioxide, creating the gas in the blisters that are symptomatic of gas gangrene. 80-90% of gas gangrene cases are caused by Clostridium perfrigens. Clostrdium are ubiquitous and species can be found in environments ranging from the human gastrointestinal tract to soil.[2] Clostridial myonecrosis typically occurs when open or traumatic wounds are exposed to these bacteria and subsequently become contaminated with spores or vegetative cells. Historically, gas gangrene has been associated with battlefield wounds but is now mostly obsolete due to modern advances in health and technology.[3] Modern cases of clostridial myonecrosis also include surgical contamination and also spontaneous infection. Most infections happen under circumstances of poor vascular health or vascular damage which obstruct blood flow and allow anoxic tissue.[3] Presently, about 90% of contaminated traumatic wounds exhibit Clostridium culture but less than 2% develop myonecrosis.[1] Myonecrosis can also occur without an open wound if blood flow to tissues is reduced. Because of this, people who have vascular disease affecting blood flow to extremities (such as diabetes mellitus or atherosclerosis) have a higher risk of mortality.[1] Untreated clostridial myonecrosis carries a 100% fatality rate. Treated myonecrosis has a mortality rate of 20-30% and spontaneous cases have a mortality rate between 67-100%. Infections in the trunk of the body have a higher mortality rate than extremities such as arms or legs.[1] Myonecrosis most commonly occurs in the extremities and symptoms of infection develop six to forty-eight hours after initial infection of Clostridium. Initial symptoms of infection include sudden and severe pain in the site of infection before clinical signs are noticeable. Body temperature is initially within normal range but quickly rises soon after. Skin surrounding the infection site is initially tight and shiny but then quickly becomes greyish and then dark. Muscles are dark red, black, or green and do not contract or bleed. A sickly sweet odor is often present. Necrosis can spread as quickly as 6 inches per hour.[4] Shock and death can occur within 12 hours. Because infection is very aggressive and progresses extremely quickly, treatment must also be aggressive, ranging from antibiotics to amputation.[5] Infections are typically characterized with very little host response to Clostridium. Immune response is in reaction to exotoxins produced by Clostridium rather than actual bacterium. There is usually no pus created during infection.[1]The exact reason as to why there is poor immune response is not exactly known. For infection to happen, the partial pressure of oxygen must be low enough for Clostridium to grow. Clostridium perfrigens are not strict anaerobic bacteria, they can grow freely in 30% oxygen and 70% oxygen severely reduces their growth. After infection, incubation of Clostridium can range from one hour to many weeks. Toxins such as lecithinase, collagenase, hyaluronidase, hemagglutinin and hemolysin toxins are released into the surrounding tissue. Types of toxins include theta, kappa and alpha-toxin. Theta- toxin causes vascular injury and destroys leukocytes. Kappa toxin destroys connective tissue and helps speed the necrosis. Alpha-toxin lyses red blood cells, leukocytes, platelets, myocytes, and fibroblasts. Symptoms of gas gangrene include:[5] - Fever, vomiting, increased heart rate and sweating - Painful swelling around wound - Pale skin that turns grey, purple, black or dark red - Blisters with smelly discharge - Jaundice
Types
Clostridium perfrigens produces more than twelve toxins that may be disease-causing. At least eight of the toxins are thought to be lethal. The major toxins of Clostridium perfrigens are alpha, beta, epsilon, and iota. These toxins group C. perfrigens into the five toxigenic types: A, B, C, D, and E. Type A strains produce mostly alpha-toxin. Type B strains produce beta and epsilon toxins. Type C strains produce beta and delta toxins. Type D produce the epsilon toxin and type E produces iota toxin.[6] Like alpha-toxin, beta-toxin releases arachidonic acid and produce transmembrane pores in eukaryotic cells and have hemolytic activity.[7] Delta-toxin is also a hemolytic toxin that bind to the GM2 ganglioside on erythrocytes.[8] Kappa-toxin digests collagen in connective tissues, breaking down skeletal muscle. Epsilon-toxin is a toxin that forms pore. The pores leak red blood cells and serum proteins.[9]Iota-toxin is a binary toxin that performs ADP-ribosylation of actin.[10]
Theta-Toxin
Theta (θ) toxin, also known as perfringolysin O (PFO) is a toxin secreted by Clostridium perfringens that is a pore-forming chlosterol-dependent cytolysin. PFO is expressed in almost all Clostridium perfrigens strains and plays a role in gas gangrene advancement. The genes for producing PFO and alpha-toxin are located on the chromosome rather than on the plasmid (such as beta, epsilon and iota). Although PFO is widely encoded by most C. perfrigens strains, some food-poisoning strains do not have the PFO gene.[11]
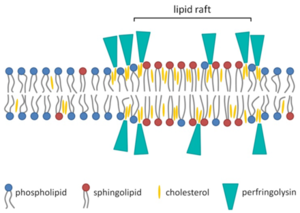
PFO is an average sized protein (500 amino acids) that varies in protein structure and gene location. This effects virulence of the bacteria and is likely explained by recombination.[11] PFO also contains a signal peptide that helps secretion through general secretory pathway (GSP). When traversing the cell membrane, it is cleaved. In solution at high concentrations, PFO likely forms high concentrations with a head-to-tail structure. PFO monomers contain four domains that are mostly β-barrels. PFO is dependent on cholesterol as a cellular receptor for pore formation. Cholesterol is ubiquitous in mammalian membranes. The mechanism of PFO cytolysis of mammalian cells begins with the loops of domain four attaching to the plasma membrane of the eukaryotic cell. One of the loops contains a conserved undecapeptide which helps anchor the PFO to the membrane of the cell and initiate necessary conformational changes to allow the β-barrel to enter the membrane of the eukaryotic cells. Binding capacity of the PFO may be dependent on the accessibility of the cholesterol in the cellular membrane, which is dependent on the spacing of the phospholipid groups and the saturation level of the cholesterol-phospholipid acyl chain.[11] Thickness of the phospholipid bilayer may affect the efficiency of pore formation by PFO. When domain four binds to the eukaryotic cellular membrane, the PFO molecule undergoes structural changes and allows association with another monomer. The prepore structure then forms. The prepore structure is made of oligomerized monomers that have not inserted the β-barrel into the membrane yet. Two amphipathic transmembrane β-hairpins are inserted into the membrane by each monomer. Every single monomer does same action in synchronization.
Alpha and Theta-Toxin Synergy in Immune Response
The PFO gene acts synergistically with the alpha-toxin gene, with drastically decreased virulence in mutant strains lacking both genes. Clostridial myonecrosis is often characterized by poor immune response. The immune system is capable to killing unidentified C. perfrigens in both anaerobic and aerobic conditions—the problem is not in the killing ability of the immune system but rather the evasiveness and indirect methods of blocking the immune system that the bacteria uses. Alpha-toxin also directly promotes platelet aggregation and PFO promotes an increased expression of adhesion molecules. The combination of the two toxins promotes increased leukocyte adhesion through upregulation of adhesion molecules to endothelial cells after subjection to the alpha-toxin.[3] Normally, these markers would help traffic neutrophils into the site of infection. However, the upregulation of increased adherence traps the neutrophils into the lining of the blood vessels, causing thrombosis, edema and poor blood flow.[11] This, in turn, results in poor movement of leukocytes to the area of infection and contributes to low levels of oxygen and increased growth and spread of Clostridium. C. Perfrigens is able to evade the phagosome only through the presence of both PFO and alpha-toxin. PFO is also critical for macrophage cytotoxicity.[12]PFO’s effect on the eukaryotic membrane benefits the bacteria because it also aids in escape of the macrophage. Although immune response is poor, it is not absent. Infection still requires a moderately large population of bacteria, showing that the immune system does play a role in obstructing the infection.[12]
Alpha-Toxin
Alpha-toxin, or phospholipase C (PLC), degrades lecithin and sphingomyelin in eukaryotic cell membranes. Lecithin is any sort of yellow-brown fatty acid substances which are amphiphilic and sphingomyelin, a type of sphingolipid found in eukaryotic membranes.[12] Alpha-toxin is uniquely an enzyme and also has contains two Zn2+ ions that are essential for catalysis.[3] The structure of alpha-toxin contains two domains with the N-terminal composed of α-helices and the C-terminal composed of β-sheets. The N-domain contains the phospholipase C active site.[3]
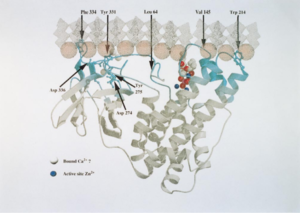
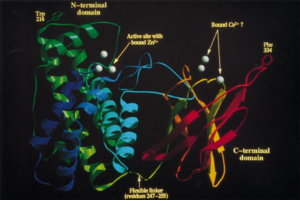
The function of the C-domain is not fully understood, however recent studies suggest that the C-domain may be important for the interaction between alpha-toxin and membrane phospholipids through calcium ions that are coordinated by the amino acid residues.[3] There are structural variants of the alpha-toxin, individual to the bacterium. Structural differences usually do not affect the phospholipase C activity but may have biological significance. Some variations are more resistant to degradation.[3]
Cell Damage
Cell Damage: In gas gangrene, alpha-toxin has three crucial roles: it is able to evade leukocytes, reduce blood supply through damaging the vascular system, and also changes host cell metabolism by activating protein kinase C and arachidonic acid cascade.[3] Alpha-toxin increases the making of intercellular adhesion molecules, interleukin-8, platelet-activating factor, endothelial leukocyte adhesion, and other molecules that increase edema and transportation across vascular membranes.[13] Alpha-toxin creates huge phosphorylcholine (PC) and carboxyfluorescien (CF) destruction in the cell membrane, resulting in the rupture of the membrane. The enzyme has with a strong affinity for PC and CF. Exposed hydrophobic residues on the N- and C- domains are docked with the membrane bilayer and are in position to coordinate with the fatty-acyl groups of the phospholipids.[3] The hemolytic activity of the alpha-toxin arises from the hydrolysis of cell membrane phospholipids.[3] However, there is data suggesting that some hydrolysis of the membrane is caused by naturally occurring phospholipase C in the cell’s own membrane, which is activated by the protein kinase C pathway. In addition, in vitro, cells attacked with alpha-toxin produced increased levels of arachidonic acid and metabolites of arachidonic acid, resulting in increased vasoconstriction.[3]Increased vasoconstriction would benefit the bacterium due to lower levels of oxygen and poor leukocyte transport.
cpa Gene
cpa Gene: The cpa gene which encodes for the alpha-toxin is located on the most stable region of the chromosome of the bacterium, possibly due to the critical nature of this toxin for the survival of C. perfrigens. Alpha-toxin, like theta-toxin, is one of the few toxins located on the genome and not on a plasmid in the cell, showing the conservation and importance of the toxin. Transcriptional regulation of cpa controls levels levels of alpha-toxin production. Common to many pathogenic bacteria, C. perfrigen regulates gene expression of cpa through the VirR/VirS system, with VirS acting as a sensor histidine kinase in response to a currently unknown environment signal, which then phosphorylates VirR.[3]The phosphorylated VirR is then able to control the transcription of specific genes. In mice, the inactivation of the cpa gene resulted in attenuated strains of C. perfrigens. Mice that were also immunized with an alpha-toxoid protected them from experimental gas gangrene, which may lead for possible future treatment.[3]
Kappa Toxin
Kappa-toxin, like alpha-toxin, is also an enzyme. Kappa toxin digests collagen and collagen-related products and does not target other products such as albumin or hemoglobin. However, because collagen makes up about 25% of total protein content in the body, mostly as connective tissue, this enzyme is highly disruptive to bodily function.[14] Upon intravenous injection of kappa-toxin, the reticulin framework of tissue suffers extensive damage, up to hemorrhage into the lungs.[14] Injection into the muscle reduces tissue into a swampy, bloody mush. In gas gangrene, kappa-toxin is responsible for the reduction of muscle into pulp. Kappa-toxin also acts as a virulence factor by releasing amino acids that promote the further growth of C. perfrigens.[14]

Vaccines and Antitoxins
Because gas gangrene is not a prevalent issue in the general population, vaccines and antitoxins have not received much attention. In addition, cases are hard to predict because infection is opportunistic. However, gas gangrene is an issue for elderly, immunocompromised people, people with peripheral arterial disease or victims or traumatic injury. Surgery in the abdomen, especially in the gastrointestinal tract, raise chances for infection with gas gangrene.[3] Vaccines created from alpha-toxin can use filtrates of C. perfringens type A. However, a recurring issue is the creation of a toxoid eliciting an antagonistic immune response. Some instances of anti-toxin for gas gangrene has been extremely successful. In a review of 113 cases9, an average of 75% recovery was made after two doses of antiserum post-infection. In other cases, antiserum did not make a difference in the prevention of gas gangrene which may reflect the difficulties in preparing an immunogenic toxoid as well as inoculation immediately after injury.[13] Alpha-toxin and its cpa gene is well characterized, opening opportunities for genetically defined toxoids. The C-domain of alpha-toxin lacks enzymatic and toxic activity and may be a source of immunization. In mice vaccinated with the C-domain polypeptide, an antibody response that protect against at least 50MLD of alpha-toxin was elicited. However, this is specific to C. perfrigens. Further research on a different toxoid must be investigated for different infections of Clostridium. Immunization with toxoid of alpha-toxin did provide protection of 10MLD of C. perfrigens type A in mice.[3] A benefit to this vaccine is that it can be produced in E. coli. Inactive mutated forms of alpha-toxin would also be a viable option due to mutations on cpa that have been shown to express low levels of lethality. However, research has yet to be done on this method of making vaccines.[3] Difficulties in producing an effective vaccine currently lie in antigenic architecture of the alpha-toxin. Monoclonal antibodies that neutralize the phospholipase C activity do not necessarily do the same activity for hemolytic and other lethal functions of the alpha-toxin. This supports the suggestion that the N-domain of alpha-toxin holds the lethalities of the alpha-toxin while C-domain does not. Experimental data of inoculation with N-domain versus C-domain of alpha-toxin show that inoculation with C-domain provided protection and stopped all biological activity, while N-domain produced antibody which stopped phospholipase C activity but no other lethal functions.[3]
Treatment
Treatment must be aggressive and timely. The “triangle” of treatment for gas gangrene is describes as: antibiotics, hyperbaric oxygen therapy, and surgery. High doses of antibiotics such as penicillin and clindamycin will be administered intravenously, even before proper diagnosis. Protein synthesis inhibitors such as rifampin or tetracycline are also effect because they inhibit the synthesis of exotoxins produced by Clostridium. Additional anti-microbial agents are recommended because nonclostridial bacterial are often found in gas gangrene cultures.[15] All infected tissue will have to be removed, sometimes resulting in amputation. If wounds had previously healed, it is optimal to reopen and clean them. Debridement of necrotic tissue must be performed often under close watch until normal production of tissue resumes.

Reconstructive surgery such as skin grafts may be used to help mitigate the loss of tissue. Prosthetics are an option for amputations. Sometimes, hyperbaric oxygen therapy will be used to help treat gas gangrene because the elevated oxygen content in the blood will help wound healing.[5] However, results for hyperbaric oxygen therapy are conflicting because there are few comparative studies. However, studies on animal models indicate that hyperbaric oxygen therapy severely restricts Clostridium, likely due to the increased oxygen content in tissue.[15] The production of alpha-toxin by Clostridium is the main cause of the rapidly advancing tissue necrosis. A tissue partial pressure of oxygen of 250mmHg is needed to stop production of toxins, so early treatment and repetitive (more than two times) of hyperbaric oxygen is best.[4]
Major clinical studies have shown that the most mortality was achieved with initial conservative surgery and then rapid follow-up with hyperbaric oxygen therapy. When hyperbaric oxygen therapy is delayed, mortality rate steeply increases. Aggressive surgery is not recommended—it is better to wait until the line between dead tissue and healthy tissue is apparent before surgery.[4] It is also important to begin hyperbaric oxygen therapy even before surgery—amputation rates drastically decrease without prompt, initial hyperbaric oxygen therapy.
References
- ↑ 1.0 1.1 1.2 1.3 1.4 1. Qureshi, S., MD. (2017, January 18). Clostridial Gas Gangrene (J. Geibel MD, Ed.). Retrieved April 23, 2017, from http://emedicine.medscape.com/article/214992-overview#a4
- ↑ 1. Clostridium perfringens, their Properties and their Detection. (n.d.). Retrieved April 24, 2017, from http://www.sigmaaldrich.com/technical-documents/articles/analytix/clostridium-perfringens.html
- ↑ 3.00 3.01 3.02 3.03 3.04 3.05 3.06 3.07 3.08 3.09 3.10 3.11 3.12 3.13 3.14 3.15 3.16 1. Titball, Richard W., Claire E. Naylor, and Ajit K. Basak. "The Clostridium perfringensα-toxin." Anaerobe 5.2 (1999): 51-64.
- ↑ 4.0 4.1 4.2 1. Bakker, D. J. (2012). Clostridial myonecrosis (gas gangrene). Undersea & Hyperbaric Medicine, 39(3), 731.
Cite error: Invalid
<ref>
tag; name "bakker" defined multiple times with different content - ↑ 5.0 5.1 5.2 1. Pietrangelo, A., MD, & Cirino, E., MD. (2016, February 16). Gas Gangrene. Retrieved April 23, 2017, from http://www.healthline.com/health/gas-gangrene#overview1
- ↑ 1. McDonel, J. L. (1980). Clostridium perfringens toxins (type a, b, c, d, e). Pharmacology & therapeutics, 10(3), 617-655.
- ↑ 1. Steinthorsdottir, V., Halldórsson, H., & Andrésson, O. S. (2000). Clostridium perfringens beta-toxin forms multimeric transmembrane pores in human endothelial cells. Microbial pathogenesis, 28(1), 45-50.
- ↑ 1. Cavaillon, J. M., Jolivet-Reynaud, C., Fitting, C., David, B., & Alouf, J. E. (1986). Ganglioside identification on human monocyte membrane with Clostridium perfringens delta-toxin. Journal of leukocyte biology, 40(1), 65-72.
- ↑ Cole, A. R., Gibert, M., Popoff, M., Moss, D. S., Titball, R. W., & Basak, A. K. (2004). Clostridium perfringens [straight epsilon]-toxin shows structural similarity to the pore-forming toxin aerolysin. Nature structural & molecular biology, 11(8), 797
- ↑ 1. Tsuge, H., Nagahama, M., Nishimura, H., Hisatsune, J., Sakaguchi, Y., Itogawa, Y., ... & Sakurai, J. (2003). Crystal structure and site-directed mutagenesis of enzymatic components from Clostridium perfringens iota-toxin. Journal of molecular biology, 325(3), 471-483.
- ↑ 11.0 11.1 11.2 11.3 1. Verherstraeten S, Goossens E, Valgaeren B, et al. Perfringolysin O: The Underrated Clostridium perfringens Toxin? Popoff MR, ed. Toxins. 2015;7(5):1702-1721. doi:10.3390/toxins7051702.
- ↑ 12.0 12.1 12.2 1. O'Brien, D. K., & Melville, S. B. (2004). Effects of Clostridium perfringens alpha-toxin (PLC) and perfringolysin O (PFO) on cytotoxicity to macrophages, on escape from the phagosomes of macrophages, and on persistence of C. perfringens in host tissues. Infection and immunity, 72(9), 5204-5215.
- ↑ 13.0 13.1 1. Jun Sakurai, Masahiro Nagahama, Masataka Oda; Clostridium perfringens Alpha-Toxin: Characterization and Mode of Action. J Biochem 2004; 136 (5): 569-574. doi: 10.1093/jb/mvh161
- ↑ 14.0 14.1 14.2 1. Louis D. S. Smith. (1979). Virulence Factors of Clostridium perfringens [with Discussion]. Reviews of Infectious Diseases, 1(2), 254-262. Retrieved from http://www.jstor.org/stable/4452312
- ↑ 15.0 15.1 1. Hoi, H., MD, & Cunha, B. A., MD. (2017, February 09). Gas Gangrene Treatment & Management. Retrieved April 24, 2017, from http://emedicine.medscape.com/article/217943-treatment
Authored for BIOL 238 Microbiology, taught by Joan Slonczewski, 2017, Kenyon College.