Evolution of Thermophilic Archaea: Difference between revisions
Line 24: | Line 24: | ||
Three main features present in most species of archaea are possible adaptations to high temperatures, perhaps indicating that the common ancestor of all archaea was a hyperthermophile.[http://books.google.com/books?id=FtSzl4iastsC&pg=PA142&lpg=PA142&dq=Thermophiles%2BDNA2Bevolution&source=bl&ots=xOvoRm4OZY&sig=70RaRixc8sPV9gZ0ue8TdX9pH4&hl=en&ei=bOPkSYfFCdjelQeS373gDg&sa=X&oi=book_result&c=result&resnum=6#PPA141,M1 4] The first characteristic is the ubiquitous presence of the topoisomerase reverse gyrase. As its name suggests, reverse gyrase coils DNA in the opposite direction in which regular gyrase coils it: DNA coiled by reverse gyrase forms positive supercoils instead of negative supercoils.[http://www.jbc.org/cgi/content/abstract/281/9/5640 3] Most thermophiles, both bacteria and archaea, have positive supercoils in their DNA; as positive supercoils are stronger and more difficult to untangle than negative supercoils, they are useful to organisms which grow at high temperatures, as the heat cannot destroy the genetic material as easily. While not all the DNA in today's archaea is found in positive supercoils, reverse gyrase is found not only in thermophilic archaea, but in some species of mesophilic archaea, indicating that perhaps it was present in the common ancestor of all archaea. | Three main features present in most species of archaea are possible adaptations to high temperatures, perhaps indicating that the common ancestor of all archaea was a hyperthermophile.[http://books.google.com/books?id=FtSzl4iastsC&pg=PA142&lpg=PA142&dq=Thermophiles%2BDNA2Bevolution&source=bl&ots=xOvoRm4OZY&sig=70RaRixc8sPV9gZ0ue8TdX9pH4&hl=en&ei=bOPkSYfFCdjelQeS373gDg&sa=X&oi=book_result&c=result&resnum=6#PPA141,M1 4] The first characteristic is the ubiquitous presence of the topoisomerase reverse gyrase. As its name suggests, reverse gyrase coils DNA in the opposite direction in which regular gyrase coils it: DNA coiled by reverse gyrase forms positive supercoils instead of negative supercoils.[http://www.jbc.org/cgi/content/abstract/281/9/5640 3] Most thermophiles, both bacteria and archaea, have positive supercoils in their DNA; as positive supercoils are stronger and more difficult to untangle than negative supercoils, they are useful to organisms which grow at high temperatures, as the heat cannot destroy the genetic material as easily. While not all the DNA in today's archaea is found in positive supercoils, reverse gyrase is found not only in thermophilic archaea, but in some species of mesophilic archaea, indicating that perhaps it was present in the common ancestor of all archaea. | ||
[[Image:Esterether.jpg|thumb|left|Figure 4: Picture comparing ether linkages in an L-glycerol, as found in archaea, with ester linkages in a D-glycerol, as found in bacteria http://www.ucmp.berkeley.edu/archaea/archaeamm.html]] | [[Image:Esterether.jpg|thumb|left|Figure 4: Picture comparing ether linkages in an L-glycerol, as found in archaea, with ester linkages in a D-glycerol, as found in bacteria http://www.ucmp.berkeley.edu/archaea/archaeamm.html]] | ||
The second characteristic is the ether-linked L-lipids found in archaeal membranes. Because ether bonds are much stronger than ester bonds, the membrane lipids of archaea do not easily come apart at high temperatures, unlike the ester-linked D-lipids of most bacteria. (<b>Figure 4</b>) Ester-linked lipids are the only kind of glycolipids present in archaea; here is further evidence of thermophilic ancestors passing on heat adaptations to future generations. The third characteristic is the high number of modified nucleosides in archaea. Most of the modification occurs in the bases of these molecules. Nine modified nucleosides are known to be present only in archaea, and all of them are most commonly found in thermophilic species, most of which were discovered in a 1991 experiment. [http://www.pubmedcentral.nih.gov/pagerender.fcgi?artid=207908&pageindex=2#page 5] Eleven species of thermophilic archaea were studied in this experiment: <i>Thermoplasma acidophilum, Methanobacterium thermoautotrophicum, Archaeoglobus fulgidus, Methanothermus fervidus, Sulfolobus solfataricus, Thermoproteas neutrophilus, Acidianus infernus, Thermodiscus maritimus, Thermococcus sp., Pyrobaculum islandicum</I>, and <i>Pyrodictium occultum</i>. The occurrance of the same modified nucleosides across these different genera of thermophiles indicates at least a common ancestor of thermophilic archaea, if not of archaea in general. | The second characteristic is the ether-linked L-lipids found in archaeal membranes. Because ether bonds are much stronger than ester bonds, the membrane lipids of archaea do not easily come apart at high temperatures, unlike the ester-linked D-lipids of most bacteria. (<b>Figure 4</b>) In addition, the lipids found in archaea are composed of branched isopropene chains, as opposed to those found in bacteria, which are composed of unbranched fatty acids. Ester-linked lipids are the only kind of glycolipids present in archaea; here is further evidence of thermophilic ancestors passing on heat adaptations to future generations. The third characteristic is the high number of modified nucleosides in archaea. Most of the modification occurs in the bases of these molecules. Nine modified nucleosides are known to be present only in archaea, and all of them are most commonly found in thermophilic species, most of which were discovered in a 1991 experiment. [http://www.pubmedcentral.nih.gov/pagerender.fcgi?artid=207908&pageindex=2#page 5] Eleven species of thermophilic archaea were studied in this experiment: <i>Thermoplasma acidophilum, Methanobacterium thermoautotrophicum, Archaeoglobus fulgidus, Methanothermus fervidus, Sulfolobus solfataricus, Thermoproteas neutrophilus, Acidianus infernus, Thermodiscus maritimus, Thermococcus sp., Pyrobaculum islandicum</I>, and <i>Pyrodictium occultum</i>. The occurrance of the same modified nucleosides across these different genera of thermophiles indicates at least a common ancestor of thermophilic archaea, if not of archaea in general. | ||
==Horizontal Gene Transfer Between Thermophilic Bacteria and Thermophlic Archaea== | ==Horizontal Gene Transfer Between Thermophilic Bacteria and Thermophlic Archaea== |
Revision as of 22:21, 20 April 2009
by Julia DeNiro
Crenarcheota and Hyperthermophiles: An Overview
Thermophilic and hyperthermophilic archaea, specifically crenarcheotes in the class Thermoprotei, are known to inhabit environments such as hot springs, ocean vents, and geysers which are inhospitable to many other forms of life. (Figure 2)
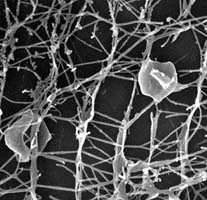
Such habitats are high in concentration of reduced minerals and in temperature, and low in concentration of oxygen and often in pH. Though various genera of these archaea flourish at different temperatures, the most striking examples of hyperthermophiles include archaea in the genera Pyrodictium, (Figure 1) which are adapted to live around thermal vents at the ocean floor, at temperatures ranging from 100-110 degrees Celsius. Acidophiles are also prevalent in this class, especially those belonging to the order Sulfolobales: Sulfolobus solfatericus flourishes at a pH ranging from only 2 to 4.
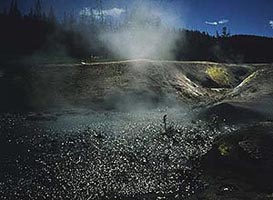
Though certain species of Thermoprotei use oxygen in respiration, most of the metabolisms of these archaea are anaerobic. Ignicoccus islandicus oxidizes hydrogen with sulfur, forming hydrogen sulfide. Pyrodictium might oxidize hydrogen with sulfur or else use anaerobic fermentation.
The hostile habitats in which crenarcheotes live resemble, in many cases, the conditions on early Earth. As the early atmosphere is believed to have had no oxygen in it, the first life forms to develop must clearly have been anaerobic. If life forms evolved before Earth cooled--and it is quite possible that they did, as they could have flourished beneath Earth's forming crust, away from crashing meteorites--these organisms might have been the ancestors of today's thermophilic prokaryotes, including archaea. Though it is relatively certain among microbiologists that the first microbes, ancestors of all life on Earth today, evolved nearly 4 billion years ago, it is still quite uncertain whether these early microbes were thermophiles.
Microfossils and Isotopic Evidence
Though remains of microbial life nearly 4 billion years old have been found, it is typically impossible to tell whether this life was more closely related to bacteria or to archaea. The different forms of proteins and rRNA that distinguish archaea from bacteria are, of course, not preserved in microfossils. Isotopic records can be made of the lipids in ancient cells, but the isopropene chains that comprise archaeal lipids have not yet been found in rocks older than 1.6 billion years. 6 If we were to draw conclusions about archaeal evolution solely from this evidence, we would assume that archaea in their present form evolved relatively late in the history of microbes. However, other isotopic records have provided evidence of the early existence of methanogenesis, about 2.7 Gya. Methanogenesis today only occurs in eukaryarchaea. If this process was occurring nearly 3 Gya ago, it is likely that archaea had evolved much earlier. Whether or not these early archaea were thermophilic or the ancestors of today's crenarchaea, however, is still a matter of speculation and hypothesis.
In 2000, strange filaments were first reported in volcanic hosted massive sulfide deposits (VHMS) at the Kangaroo Caves Formation, East Pilbara, Western Australia. (Figure 3) It was hypothesized that these filaments were microfossils, specifically of ancient thermophilic chemotrophs. Though non-biological filamentous mineral growths can be easily found in sulfide deposits, the filaments at Kangaroo Caves differed in that they were unbranched, with constant diameters. Moreover, they were entangled and clustered in specific ways which resemble those of certain modern thermophilic microbes and other microfossils1.
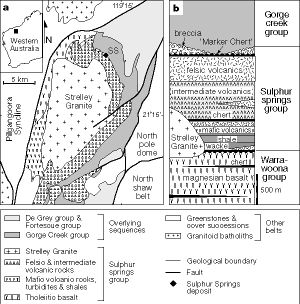
Changes in orientation of filaments in different areas may even be evidence of biological variation between organisms in different microenvironments; such a phenomenon is common today in modern hot-spring thermophiles.
Metal precipitation and volcanic rock textures indicate that East Pilbara was once covered by over 1000 m of seawater. It is estimated from looking at the minerals in the ore that temperature might have reached 300 degrees Celsius, and this hypothesis, coupled with the volcanic rock found in the deposit, is substantial evidence that Kangaroo Caves was once a hydrothermal vent in a primordial sea2.
The rocks and the structures in them are about 3.2 billion years old, meaning that if these filaments really are the remains of primitive microbes, they were flourishing in the Archaean Eon, at the same time as many ancient cyanobacteria. Neither cell structure nor metabolism can be determined by looking at these filaments; even if we could be sure that they were microfossils, we could still not tell whether they were bacteria or archaea. However, it is an exciting possibility that thermophilic life did exist on early Earth and perhaps evolved even earlier, and that if the filaments are microfossils of thermophiles, they are the ancestors of today's thermophilic archaea.
Archaeal Traits as Possible Adaptations to High Temperatures
Three main features present in most species of archaea are possible adaptations to high temperatures, perhaps indicating that the common ancestor of all archaea was a hyperthermophile.4 The first characteristic is the ubiquitous presence of the topoisomerase reverse gyrase. As its name suggests, reverse gyrase coils DNA in the opposite direction in which regular gyrase coils it: DNA coiled by reverse gyrase forms positive supercoils instead of negative supercoils.3 Most thermophiles, both bacteria and archaea, have positive supercoils in their DNA; as positive supercoils are stronger and more difficult to untangle than negative supercoils, they are useful to organisms which grow at high temperatures, as the heat cannot destroy the genetic material as easily. While not all the DNA in today's archaea is found in positive supercoils, reverse gyrase is found not only in thermophilic archaea, but in some species of mesophilic archaea, indicating that perhaps it was present in the common ancestor of all archaea.
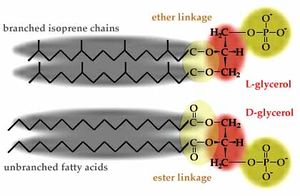
The second characteristic is the ether-linked L-lipids found in archaeal membranes. Because ether bonds are much stronger than ester bonds, the membrane lipids of archaea do not easily come apart at high temperatures, unlike the ester-linked D-lipids of most bacteria. (Figure 4) In addition, the lipids found in archaea are composed of branched isopropene chains, as opposed to those found in bacteria, which are composed of unbranched fatty acids. Ester-linked lipids are the only kind of glycolipids present in archaea; here is further evidence of thermophilic ancestors passing on heat adaptations to future generations. The third characteristic is the high number of modified nucleosides in archaea. Most of the modification occurs in the bases of these molecules. Nine modified nucleosides are known to be present only in archaea, and all of them are most commonly found in thermophilic species, most of which were discovered in a 1991 experiment. 5 Eleven species of thermophilic archaea were studied in this experiment: Thermoplasma acidophilum, Methanobacterium thermoautotrophicum, Archaeoglobus fulgidus, Methanothermus fervidus, Sulfolobus solfataricus, Thermoproteas neutrophilus, Acidianus infernus, Thermodiscus maritimus, Thermococcus sp., Pyrobaculum islandicum, and Pyrodictium occultum. The occurrance of the same modified nucleosides across these different genera of thermophiles indicates at least a common ancestor of thermophilic archaea, if not of archaea in general.
Horizontal Gene Transfer Between Thermophilic Bacteria and Thermophlic Archaea
If the molecular characteristics of archaea are ancient adaptations to thermophily, why are they only shared by archaea? Why have they not developed in thermophilic bacteria? There is no real answer to this question, but previous studies have shown that thermophilic bacteria such as Aquifex and Thermotoga diverged relatively early from the rest of the bacterial lineage. In fact, Aquifex and Thermotoga share so many metabolic traits with thermophilic archaea--reduction of hydrogen for energy and growth at high temperatures, for example--and so much gene transfer has occurred between these bacteria and archaea, that these two genera of bacteria and archaea might be more closely related than previously thought. A 1998 experiment demonstrated that the bacterium Aquifex aeolicus and various species of hyperthermophilic archaea share remarkable genetic similarities. 7 It was discovered that a high fraction of A. aeolicus gene products have proteins commonly found in archaea, a much higher fraction than gene products present in non-thermophilic bacteria, such as Bacillus subtilis and E. coli. (Figure 5)
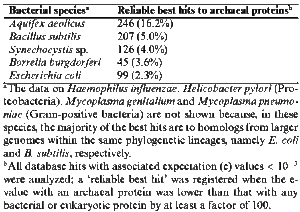
According to the experiment, at least 10% of Aquifex genes have been horizontally transferred from archaea. 7 This discovery does not necessarily indicate that the two lineages are closely related, as horizontal transfer occurs between nonrelated bacteria species, as well as between bacteria and archaea and even bacteria and eukaryotes. However, the archaeal species with which Aquifex shares its genes are all thermophilic, and so the gene exchange could have been a result of convergent evolution, in which tolerance of high temperatures was selected for. Therefore, probably both Aquifex and the archaea used in the 1998 experiment evolved at a time and in an environment in which growth at high temperatures was necessary for survival, perhaps during a time of uncontrolled global warming. Because of the relative old age of such bacterial lineages as Aquifex and its apparent early divergence from other bacteria, this convergence possibly occurred in the Hadean Era, while Earth was still cooling. However, as of yet we can only infer these possibilities. There is not yet enough information on genome sequences in bacterial thermophiles to determine just how ancient they are, or how many genes they exchanged with archaeal thermophiles.
Conclusion
Unfortunately, despite the genomic, metabolic, and fossil evidence of the early evolution of thermophilic archaea, not enough information has been collected to draw any conclusions. The fossil record is incomplete; there is no concrete evidence of archaeal evolution before 1.6 Gya, long after microbes first evolved on this planet. It is not even certain whether certain earlier fossils found in sulfide deposits are really the remains of thermophiles. Though various traits useful to thermophiles are shared by all archaea, they could have developed because of convergent evolution, perhaps in response to a global warming event later than 3 Gya, not necessarily because all archaea share a common, thermophilic ancestor. Though there is a great deal of genetic similarity between thermophilic bacteria and thermophilic archaea, most of it is the result of horizontal gene transfer, and in any case, not enough genomes of thermophilic bacteria have yet been sequenced to draw conclusions about their relationship with thermophilic archaea. In short, much more research must be done and much more evidence, both ancient and modern, must be collected before microbiologists can reasonably hypothesize that archaeal lineages appeared early in the history of Earth, or that present-day archaea, let alone all life on Earth, evolved from a thermophilic ancestor.
References
(1)Wacey, David. Early Life on Earth. 2009. Topics in Geobiology. Volume 31. p. 221-227
(2)Rasmussen, Birger. "Filamentous microfossils in a 3,235-million-year-old volcanogenic massive sulphide deposit". Nature. 2000. Volume 405. p. 676-679.
(3)Hsieh, Tao-shih and Plank, Jody L. "Reverse Gyrase Functions as a DNA Renaturase: Annealing of Complimentary Single-Stranded Circles and Positive Supercoiling of a Bubble Substrate". J. Biol. Chem. 2006. Volume 281. p. 5640-5647.
(4)Wiegel, Jurgen and Adams, Michael W.W. Thermophiles: The Keys to Molecular Evolution and the Origin of Life?. 1998. CRC. p. 141-142.
(5)Edmonds, C.G., Crain, P.F., Gupta, R, Hashizume, T., Hocart, C.H., Kowalak, J.A., Pomerantz, S.C., Stetter, K.O., and McCloskey, J.A. "Posttranscriptional modification of tRNA in thermophilic archaea (Archaebacteria)". J. Bacteriol. 1991. Volume 173. p. 3138-3148.
(6)Gribaldo, Simonetta and Brochier-Armanet, Celine. "The Origin and Evolution of Archaea: A State of the Art. The Royal Society: Biological Sciences. 2006. Volume 361. p. 1007-1022.
(7)Aravind, L., Tatusov, Roman L., Wolf, Yuri I., Walker, D. Roland, and Koonin, Eugene V. "Evidence for massive gene exchange between archaeal and bacterial hyperthermophiles". Trends in Genetics. 1998. Volume 14. p. 442-444.
(8)"Crenarcheota". 2004
(9)"Archaea: Morphology". 2008
Edited by a student of Joan Slonczewski for BIOL 238 Microbiology, 2009, Kenyon College.