Bacillus anthracis as a Bioterrorism Agent: Difference between revisions
Line 98: | Line 98: | ||
<sup>14</sup>[http://www.ncbi.nlm.nih.gov/pubmed/19793896 Kuehn A., Kovac, P., Saksena, R., Bannert, N., Klee, S.R., Ranisch, H., and Grunow, R. "Development of antibodies against anthrose tetrasaccharide for specific detection of <i>Bacillus anthracis</i> spores". <i>Clinical and Vaccine Immunology</i>. 2009. Volume 16. p. 1728-1737.] | <sup>14</sup>[http://www.ncbi.nlm.nih.gov/pubmed/19793896 Kuehn A., Kovac, P., Saksena, R., Bannert, N., Klee, S.R., Ranisch, H., and Grunow, R. "Development of antibodies against anthrose tetrasaccharide for specific detection of <i>Bacillus anthracis</i> spores". <i>Clinical and Vaccine Immunology</i>. 2009. Volume 16. p. 1728-1737.] | ||
<sup>15</sup>[http://www.ncbi.nlm.nih.gov/pubmed/11084608 Stopa, P.J. 2000. "The flow cytometry of <i>Bacillus anthracis<i | <sup>15</sup>[http://www.ncbi.nlm.nih.gov/pubmed/11084608 Stopa, P.J. 2000. "The flow cytometry of <i>Bacillus anthracis</i> spores revisited". <i>Journal of Emergency Medicine</i>. 2000. Volume 41. p. 237-244.] | ||
<sup>16</sup>[http://www.ncbi.nlm.nih.gov/pubmed/16283259 Edwards, K., Clancy, H., and Baeumner, A. "<i>Bacillus anthracis</i>:toxicology, epidemiology, and current rapid-detection methods." <i>Analytical and Bioanalytical Chemistry </i>. 2006. Volume 384. p. 73-74.] | <sup>16</sup>[http://www.ncbi.nlm.nih.gov/pubmed/16283259 Edwards, K., Clancy, H., and Baeumner, A. "<i>Bacillus anthracis</i>:toxicology, epidemiology, and current rapid-detection methods." <i>Analytical and Bioanalytical Chemistry </i>. 2006. Volume 384. p. 73-74.] |
Revision as of 18:40, 27 April 2013
Introduction
Bacillus anthracis is causative agent of anthrax which is used as an agent of bioterrorism. Anthrax is an acute disease affecting humans and animals. Previously anthrax was perceived as a damaging disease affecting domesticated animals, the farming economy, and some industrial workers; however there has been a shift to now view the disease as an agent of bioterrorism. On October 5, 2001 the first man in the United States died of anthrax in over 25 years in which B. anthracis spores were sent in the form of letters by mail. By January 26, 2002, a total of 11 cases of anthrax were identified and 5 victims died. 1 B. anthracis took the form of assymetric warfare where tactics, resources, and potential for destruction was unknown. According to the Center for Disease Control, approximately 1-2 cases of Anthrax occur each year; however in 2001, 22 cases of anthrax total occurred in the United States due to this act of bioterrorism.
B. anthracis is successful as a bioterrorism agent due to its resistance to harsh environmental conditions through its ability to exist in a spore state. With the potential to have extreme and damaging effects on the population, the study of B. anthracis and the discovery of rapid and reliable technologies to identify the species is of utmost concern.
Biology and Genetics of B. anthracis
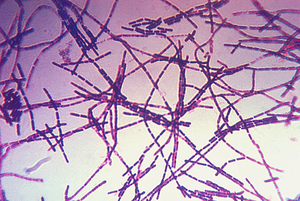
Bacillus anthracis is the organism which causes the disease of anthrax. It is a large Gram positive aerobic, rod shaped, bacillus bacterium (Figure 1). It ranges in size from 1-1.5 x 3-10 µm and is the only obligate pathogen within the genus bacillus.2
Virulence Plasmids
Two virulence plasmids, pXO1 and pXO2, encode the major virulence factors for B. anthracis.3 Plasmid pXO1 codes for three toxins which cause hemorrhage, edema, and necrosis and is 184.5 kilobase pairs (kbp) in size. The exotoxins it codes for are binary, which include the protective antigen (PA), lethal factor (LF) , and edema factor (EF). Therefore, the protective antigen it codes for acts as a binding domain on the host to permit entry of the toxin. pXO2 is a capsule bearing plasmid that is smaller than pXO1 having 95.3 kbp in size. It encodes three genes (cap A, cap B, and cap C) which are involved in the synthesis of the polyglutamyl capsule. The capsule prevents the bacteria from phagocytosis and is poorly recognized by the immune system of the host.4 Both pXO1 and pXO2 plasmids are necessary to provide the anthrax toxin and capsule respectively for B. anthracis to have full virulence. An attenuated strain results when either plasmid is lost.5
Exotoxins of Anthrax
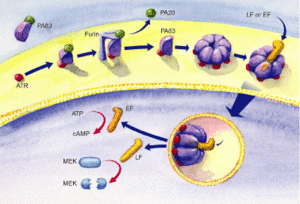
The protective antigen (PA), lethal factor (LF), and edema factor (EF) are proteins that comprise the anthrax toxin. They are encoded by the genes pag, lef, and cya, respectively. The PA factor contains the cell-binding domain. LF or EF are responsible for cell toxicity by gaining access to the host cell's cytoplasms. This is able to occur when PA locates specific host cell surface receptors and cleavage by Furin, a cell surface protein ensues. After cleavage, EF or LF binds to the PA. PA enables the EF or LF toxins to then be released into the host cell's cytoplasm to cause infection (Figure 2). EF results in edema, which is commonly referred to as swelling in which there is an accumulation of fluid trapped in a particular part of the body's tissues. If LF is released into the hosts' cells, cell death occurs.6
Spore and Vegetative State
B. anthracis is a bacterium that can exist in both a spore and vegetative state, which make it a favorable agent for bioterrorism. A spore is a dormant and resilient structure that can exist for extended periods of time with a lack of nutrients. B. anthracis can form spores that are resilient to harsh environments, such as ultraviolet and ionizing radiation, heat, and various chemicals.7 A spore can germinate to exist in its vegetative state when the environmental conditions are favorable. To become a spore again, sporulation occurs, thereby ensuring the survival of B. anthracis by during a destructive environmental condition.
Routes of Infection
Anthrax has three forms in which virulence occurs, which include cutaneous anthrax, gastrointestinal anthrax, and inhalational anthrax. When the host is exposed to the spores of B. anthracis via one of these three routes, infection occurs.
Cutaneous Anthrax
Cutaneous anthrax comprises over 90% of all human cases. It is obtained via a lesion on the skin in which infection occurs through an abrasion, cut, or insect bite.8 This type of anthrax manifests itself through a black eschar, which is dead tissue present on the surface of the skin as a scab, and is associated with edema.
Gastrointestinal Anthrax
Gastrointestinal anthrax results from the ingestion of undercooked meat from animals who have B. anthracis in its vegetative form or dormant spore state. This form of the disease has a high mortality rate because it is difficult to make an early diagnosis due to its’ non specific presentation.

Inhalation Anthrax
Inhalation anthrax is the third method in which anthrax can be contracted. This route of infection is particularly relevant for the contraction of anthrax when used as an agent of bioterrorism. Fly like symptoms are displayed initially the the B. anthracis is inhaled; therefore, it is difficult to diagnose the disease as anthrax. Traditionally, inhalation anthrax was associated with industrial exposure as spores in the textile, meat packing, leather-tanning, bone meal processing, and hair/wool-sorting industries; however it became associated with bioterrorism in 2001 when anthrax was included in envelopes mailed within the United States. Beginning with “flu-like” symptoms of mild fever, fatigue, malaise (discomfort associated with illness or infection), myalgia (muscle pain), and non-productive cough from two to five days after initial exposure, the disease develops into an acute illness characterized by dyspnea (shortness of breath), stridor (wheezing caused by a narrowed or obstructed airway), fever, cyanosis (bluish discoloration of skin), and pleural effusion (excess fluid surrounding the lungs). Anthrax manifests itself in the human body after inhalation of spores by first traveling to alveolar spaces within the lungs. Then the spores are transported through the lymph system to infect the entire body (Figure 3). Once the infection is established, it becomes refractory to treatment; therefore, the success of medical intervention is minimal. However, if the infection is diagnosed very early on, the disease may be successfully treated by multidrug antibiotic regimens and supported care, yet the prognosis of patients with inhalational anthrax is dismal as the mortality rate is quite high.9
Methods of Detection
A variety of methods exist for identifying B. anthracis. Phenotypic, antibody, and nucleic acid based methods are current techniques to indentify the toxin in its various conditions as a spore or in a vegitative state as well as the microorganisms' DNA (Figure 4).
Conventional Phenotypic Methods of Identification
B. anthracis can be detected using conventional microbiological methods via phenotypic identification. The organism selectively grows on polymyxin-lysozyme EDTA-thallous acetate (PELT) agar.10 However, this method requires a 1-2 day incubation period and requires additional tests to confirm the presence of the disease. Additionally, B. anthracis can be visualized using McFadyean’s capsule-staining test which uses McFadyean’s polychrome methylene blue to stain the capsule and rods.11 However, these traditional methods for phenotypic identification of B. anthracis tend to be the most laborious and frequently require 1-2 days of testing. Laboratory personnel require additional training and certification to accurately identify this uncommon organism, which makes these methods impractical. Additionally, to confirm the presence of the pathogen, suspect isolates must frequently be further characterized through molecular techniques.12
Antibody Based Methods of Identification
Immunoradiometric assays, IRMA, utilizes an immunofluorescence system which acts to detect antigens displayed on the surface of a spore. This method can immobilize spores in order measure concentrations of antigens through the amount of antibody used. Typically, immunofluorescence assays provide a rapid detection of spores; however, specific serological detection is complex as a result of cross-reactivity between polyclonal and monoclonal antibodies and antigens as well as between B. anthracis and closely related species such as B. cereus. Despite these complications, the technique of immunoradiometric assays has been proven to successfully identify unique B. anthracis spore surface antigens. This was determined as a result of the lack of cross-reactivity with B. cereus, a closely related species.13 However, because the antibody must be radiolabeled, this method has been supplanted with the enzyme-linked immunosorbent assay.
The enzyme-linked immunosorbent assay, ELISA, detects immobilized spores of B. anthracis through identifying the spore surface antigens. Similar to an IRMA, an ELISA quantifies the amount of antibodies or proteins present in a sample; however unlike the IRMA, the ELISA method is a non-radioactive technique. The antigen must be immobilized when the ELISA is performed on a 96-well plate to permit high throughput results. For a successful ELISA, there must be a highly specific antibody-antigen interaction, which has been accomplished in the analysis of B. anthracis species. Antibodies displayed no cross-reactivity to other members of the B. cereus group, which is closely related to B. anthracis. Therefore, this technique is a reliable and effective technique for the identification of the B. anthracis species.14
Flow cytometry has also been used to detect B. anthracis spores through fluorescently labeled antibodies. A single wavelength of light is used to excite the fluorescent labels to disntiguish the entity of interest. The technique suspends cells in a stream of fluid and directs them through an electronic detection apparaturs. Flow cytometry is advantangeous because it allow the concurrent detection of multiple characteristics of particles. Quantum dots, which have narrower emission peaks than typical fluorophores used in flow cytometry, can be used in the detection B. anthracis. They result in an increase in accuracy of detecting cells in real-time and over extended periods of time. The quantum dots cause an increase in brightness and report more consistent results than traditional fluorophores. Although flow cytometry is a rapid method, it has a low limit of detection of 103 spores, therefore samples must be in abundance, which is not ideal.15
Nucleic Acid Based Methods
Nucleic acid based methods, including PCR, Real-Time PCR, and multiplex PCR, for the detection of B. anthracis rely on the selection of nucleic acid probes that are specific to the species and must have no cross-reactivity with other species. Primers frequently target amplification of the genes, lef, pag, and cap located on either or both of the virulence plasmids pXO1 and pXO2. 16
Using PCR, strains containing the plasmids pXO1 and pXO2 are amplified. Analysis by gel electrophoresis follows as well as DNA sequencing. These methods can provide a measure of the genetic variability between isolates. The sequence rpoB is commonly used in PCR amplification of B. anthracis. The 175 base-pair sequence is highly selective for B. anthracis because it has the ability to discriminate B. anthracis from other Bacillus strains. Only one strain out of 175 tested bacilli strains has been found to be cross-reactive.17 Ultimately, PCR is able to detect the presence of both virulence plasmids, can characterize B. anthracis vaccine strains, and detect spores from soil samples.
Real-Time PCR also known as quantitative PCR (qPCR), utilizes fluorescence to detect the DNA it amplifies. Fluorescence increases in a real-time manner as additional copies of DNA are produced. This type of assay avoids the need for further processing of the DNA to detect and quantify amplified sequences separately as necessary with PCR. Additionally, amplification is observed in real-time rather than after the fact. Since amplification and detection occur in the same chamber, there is a reduced likelihood for cross-contamination of products. In the 2001 anthrax outbreak in the United States in which B. anthracis was used as an agent of bioterrorism, real-time PCR was used to test and compare 542 isolates from clinical, environmental, and suspect powder samples from both this outbreak and prior outbreaks.18 Every hour, five copies per real-time PCR reaction of B. anthracis was detected and amplified. Before amplification occurred, the method required a growth period for 18-24 hours and an extraction from 0.5-1 hour.19 100% specificity was reported using this type of assay even when 56 non B. anthracis species were tested.
Multiplex PCR amplifies specific regions of DNA using numerous sets of primers in a single reaction tube. By amplifying and detecting multiple target sequences on DNA, multiplex PCR is beneficial in eliminating false-negative and false-positive results.20
These amplification methods are often combined with high-throughput techniques for sample preparation and detection. Nucleic acid based systems are more sensitive than antigen and antibody-based detection systems in that they have the ability to detect fewer microorganisms in shorter amounts of time.21 Each method of PCR amplification has their own unique benefits for amplifying DNA; however, not all techniques are ideal to detect low levels of bacteria in environmental samples. For example, the downfall of real-time PCR is that this technique requires a DNAse step to remove DNA to prevent amplification of both DNA and RNA. The DNAse step to remove DNA has been proven to not be completely effective; therefore additional complex and time-consuming steps are necessary to ensure accurate results.
Conclusion
B. anthracis has many genetic similarities among various Bacillus species and can exist in the spore and vegetative state making it an ideal agent for bioterrorism. These features make the detection and specific identification of B. anthracis difficult and have proven to require complex techniques and laborious methods. However, targeting the virulence plasmids pXO1 and pXO2 of B. anthracis have successfully been used in detecting the species. As an agent for a potential biological threat, B. anthracis is more frequently being studied. Due to the heightened interest in the organism, there have been a large number of detection technologies that have recently been developed and are underdevelopment to reliably and quickly characterize and identify the species with the hopes of controlling anthrax outbreaks and treating the outbreaks rapidly.
References
1Spencer, R.C. "Bacillus anthracis". Journal of Clinical Pathology. 2003. Volume 56. p. 182-187.
5Spencer, R.C. "Bacillus anthracis". Journal of Clinical Pathology. 2003. Volume 56. 182-187.
Edited by Alison Lerner, a student of Nora Sullivan in BIOL187S (Microbial Life) in The Keck Science Department of the Claremont Colleges Spring 2013.