Utilization of Bacillus thuringiensis in Genetically Modified Crops: Difference between revisions
Line 28: | Line 28: | ||
==Bt Crops== | ==Bt Crops== | ||
[[Image:Bt whole process.jpeg|thumb|300px|right|Figure 4. The overall process of the production and binding of the Cry proteins. The Cry proteins are directly produced by Bt crops that have the <i>Cry</i> gene integrated into its genome. The toxins produced by the Cry proteins bind to the gut receptors of the target organism, resulting in the organism's death [10].]] | [[Image:Bt whole process.jpeg|thumb|300px|right|Figure 4. The overall process of the production and binding of the Cry proteins. The Cry proteins are directly produced by Bt crops that have the <i>Cry</i> gene integrated into its genome. The toxins produced by the Cry proteins bind to the gut receptors of the target organism, resulting in the organism's death [10].]] | ||
Crops with the incorporated <i>Bacillus thuringiensis</i> Cry gene have quickly taken over the agricultural market in the last several decades. While the usage of simple <i>Bacillus thuringiensis</i> crystals as a topical pesticide is still effective, the toxin is unable to protect the crops from any pests that may attack the plant below the surface of the soil. Crops that have successfully transformed the Cry gene into their own genome can suddenly produce their own Cry proteins. The result is new strains of plants that can protect themselves at all times both above and below the soil. These self-sufficient plants can now produce their own pesticides. The built-in insect resistance allows farmers to save the money they would spend on spraying pesticides while still producing a high yield of crops that are harvested.<br> | |||
<br>As previously explained, the Cry gene encodes for the expression of Cry proteins. A genetically modified plant steadily produces the crystalline proteins if the Cry gene has been incorporated into its genome. The genetically modified plants that contain the Cry gene are known as Bt plants. If a target organism begins to eat a Bt plant, it also is consuming Cry proteins. The proteins are solubilized once they are consumed and activated into toxins. These toxins then bind to specific receptors that are found on the epithelial cells of the insect gut. They insert themselves into the epithelial cells, which create large pores in the cells. These pores change the osmolar balance of the cells, which results in cell death (figure 4). This process occurs rapidly, resulting in the quick death of the target organism before it further damages the crop. The type of insecticide and target organism varies depending on the strain of <i>Bacillus thuringiensis</i> from which the Cry gene was extracted. For example, the strain of <i>Bacillus thuringiensis</i> that is used to make Bt176 corn targets the cell receptors in the guts of European corn borers [1]. Therefore, there are a large variety of different strains of Bt crops that are engineered to resist specific insect activity. | |||
<br> | <br> | ||
Revision as of 01:11, 22 April 2015
Introduction
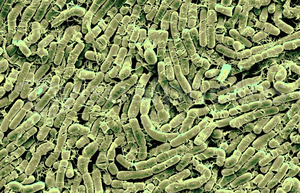
By Zoë Frazier
The bacterium Bacillus thuringiensis is a gram-positive and rod-shaped microbe that is 2–5 µm in length and 1.0 µm wide [9]. It is found in soil ecosystems, moist environments, and within caterpillar guts [5]. Bacillus thuringiensis is non-pathogenic for most organisms expect for a variety of insect species. Bacillus thuringiensis contains a gene that is known to code for Cry proteins. These proteins are produced during the sporulation phase of the Bacillus thuringiensis lifecycle. The Cry proteins are crystalline in structure and incredibly toxin to insects if consumed. The insect species that are killed by a variety of different Bacillus thuringiensis strains include vegetable insects (tomato and tobacco hornworms), field crop insects (European corn borers, alfalfa caterpillars and webworms), fruit crop insects (Leaf roller and Achemon sphinx), and tree insects (tent caterpillars, fall webworm, pine butterfly, Western spruce budworm). Additionally, mosquitoes, black flies, fungus gnats, cottonwood leaf beetles, elm leaf beetles, and Colorado potato beetles can also be affected if they consume the toxic Cry proteins [4].
Historically the Cry proteins produced by Bacillus thuringiensis have been used as a topical pesticide. However, due to advances in biotechnology, scientists have developed a mechanism to extract the Cry gene and insert it into another organism’s genome. The Cry gene is inserted into common crops that are in high demand, such as corn, wheat, cotton, canola, soy, and potato crops. Once the Cry gene is inserted into the genome, the plant can express its own insecticidal properties. This genetic alteration results in plants that are continuously producing pesticides, which helps to cut down or even eliminate the usage of spray-on pesticides. This new technological advancement is so popular in agriculture that by 2014, a total of 93% of all corn crops in the United States contained the Cry gene [3]. There are many different strains of Bacillus thuringiensis, all of which can be used to genetically modify plants to be resistant to specific target organisms. Despite the many seeming advantages to using genetically modified crops, there are several ecological effects that could drastically change the success rate of Bt crops. Both target organisms and secondary pests have been observed to adapt or evolve to resist the toxic effects of Bt crops. The following page will further describe the integration of Bacillus thuringiensis into our modern day agricultural system along with the social and ecological implications associated with the usage of this microbe.
History
In 1901, a Japanese scientist by the name of Shigetane Ishiwata was the first to discover Bacillus thuringiensis. In the early twentieth century, Bacillus thuringiensis was the cause of a widespread disease that targeted silkworms. Ishiwata was able to isolate the microbe from samples of dead silkworm larvae. In 1927, a German scientist named Ernst Berliner isolated another strain of Bacillus thuringiensis and observed that the microbe developed endospores and parasporal crystals simultaneously. It was not until 1955 that Christopher Hannay finally determined that the parasporal crystals directly caused insect death. This discovery sparked research involving the potential uses for this naturally occurring insecticide. Finally in 1961, the first Bt-based insecticide was registered by the US Environmental Protection Agency to be used in the United States. However, the market for the Bacillus thuringiensis pesticide properties changed drastically in 1982 when the technology for the genetic modification of plants was developed. Scientists discovered that if they extracted the Cry gene from Bacillus thuringiensis, inserted it into a plasmid, and integrated that plasmid into a plants genome, the plant would express a resistance to insect activity. After several years of working with the plasmid, the first crop of transgenic plants was grown in 1996 [5].
Structure and Phylogeny
Bacillus thuringiensis is a rod-shaped microbe that appears in pairs or short chains. It is a gram-positive organism that is motile. Bacillus thuringiensis contains the hag gene, which encodes for the expression of flagellin. This protein is produced in large quantities and comes together to form the microbe’s flagellum. The presence of the hag gene is crucial for the process of H serotyping, which allows us to categorize different bacterial strains based on their antigens [9]. However, H serotyping sometimes is not able to differentiate all of the different strains of Bacillus thuringiensis. Additional technology for identifying the different Bacillus thuringiensis strains has been developed due to the importance of identifying novel strains.
Bacillus thuringiensis belongs to the phylum Firmicutes, which is known to include gram-positive bacteria that produce endospores. Bacillus thuringiensis is also in the genus Bacilli, which is characterized by rod-shaped bacteria that can be aerobic or facultative anaerobes. The closest relatives to Bacillus thuringiensis are Bacillus cereus, Bacillus anthracis, and Bacillus mycoides. Although they are all close relatives, Bacillus thuringiensis is unique due to its sporulation products and environmental habitats. During sporulation, Bacillus thuringiensis produces a crystalline protein byproduct. Additionally, Bacillus thuringiensis can live in the midgut of insects and in environments that are free of other gram-positive, spore-forming, bacillus bacteria.
Interestingly, a small number of strains of Bacillus thuringiensis are known to be pathogenic to humans. Genetic sequencing of these strains shows that the insecticidal Cry genes are not present. In fact, genetic analysis shows that the two pathogenic strains, Bt 97-27 and Bt Al Hakam, are more closely related to Bacillus anthracis and Bacillus cereus than other strains of Bacillus thuringiensis. Additional analysis of 16S and 23S rDNA nucleotide sequences show that Bacillus thuringiensis and Bacillus cereus must exchange genetic material and a fairly high frequency in their natural environment [9]. Overall, there are a total of five known Bacillus thuringiensis strains, but novel strains are still being discovered.
Life Cycle
The life cycle of Bacillus thuringiensis occurs in two cycles: vegetative cell division and sporulation. During cell division, a septum forms midway along the plasma membrane. The original vegetative cell then divides into two identical daughter cells. Sporulation of Bacillus thuringiensis is a far more complicated process that involves asymmetric cell division and is completed after a total of seven stages. Stage 1 is when the axial filament forms and stage 2 is when the forespore septum begins to form. Next, during stage 3, the parasporal crystals and the forespore begin to form. Stages 4 through 6 involve the development of the spore nucleoid as well as the formation of the spore cortex and coat. In the final stage, the spore becomes fully mature (figure 2) [9]. While sporulation is a common process for many microbes, the production of the parasporal crystals is unique to Bacillus thuringiensis. These toxic crystalline proteins are created due to the expression of Cry genes and are produced only during sporulation. These proteins constitute 20-30% of the cell dry weight. Once the spores are released into a soil environment, they are able to survive for between 6 months and a year. The germination speed of the spores greatly depends on the surrounding environment. There is little bacterial competition in an insect larvae gut, which is when there are slow rates of Bacillus thuringiensis spore germination. However, in the soil, the spores have a faster germination speed, which may enhance the microbe’s ability to survive. The type of soil, whether it is used for agricultural purposes or not, does not have an effect on the survival of Bacillus thuringiensis spores. Only intense heat-shock or extremely acidic soils are shown to kill off Bacillus thuringiensis spores [11].
Bt Toxins
Figure 3.
Bt Crops
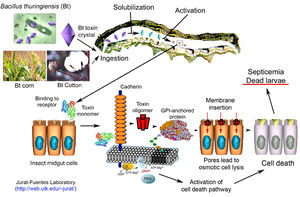
Crops with the incorporated Bacillus thuringiensis Cry gene have quickly taken over the agricultural market in the last several decades. While the usage of simple Bacillus thuringiensis crystals as a topical pesticide is still effective, the toxin is unable to protect the crops from any pests that may attack the plant below the surface of the soil. Crops that have successfully transformed the Cry gene into their own genome can suddenly produce their own Cry proteins. The result is new strains of plants that can protect themselves at all times both above and below the soil. These self-sufficient plants can now produce their own pesticides. The built-in insect resistance allows farmers to save the money they would spend on spraying pesticides while still producing a high yield of crops that are harvested.
As previously explained, the Cry gene encodes for the expression of Cry proteins. A genetically modified plant steadily produces the crystalline proteins if the Cry gene has been incorporated into its genome. The genetically modified plants that contain the Cry gene are known as Bt plants. If a target organism begins to eat a Bt plant, it also is consuming Cry proteins. The proteins are solubilized once they are consumed and activated into toxins. These toxins then bind to specific receptors that are found on the epithelial cells of the insect gut. They insert themselves into the epithelial cells, which create large pores in the cells. These pores change the osmolar balance of the cells, which results in cell death (figure 4). This process occurs rapidly, resulting in the quick death of the target organism before it further damages the crop. The type of insecticide and target organism varies depending on the strain of Bacillus thuringiensis from which the Cry gene was extracted. For example, the strain of Bacillus thuringiensis that is used to make Bt176 corn targets the cell receptors in the guts of European corn borers [1]. Therefore, there are a large variety of different strains of Bt crops that are engineered to resist specific insect activity.
Ethical Issues Surrounding Bt Crops
Include some current research, with at least one figure showing data.
Evolved Resistance and Secondary Pests
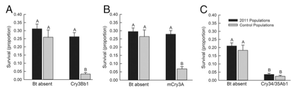
Figure 5.
Conclusion
Include conclusion
References
[1] Aeschbacher, K., Messikommer, R., Meile, L. & Wenk, C. (2005). Bt176 Corn in Poultry Nutrition: Physiological Characteristics and Fate of Recombinant Plant DNA in Chickens. Poultry Science Association, 84, 385 – 394.
[2] Catarino, R., G. Ceddia, F.J. Areal, and J. Park. (2015). The impact of secondary pests on Bacillus thuringiensis (Bt) crops. Plant Biotechnology Journal, 1-12.
[3] Cheeke, T.E., U.M. Schütte, C.M. Hemmerich, M.B. Cruzan, T.N. Rosenstiel, and J.D. Bever. (2015). Spatial soil heterogeneity has a greater effect on symbiotic aburscular mycorrhizal fungal communities and plant growth than genetic modification with Bacillus thuringiensis toxin genes. Molecular Ecology, 1-33.
[4] Cranshaw, W.S. Bacillus thuringiensis: Fact Sheet No. 5.556. Insect Series, Home and Garden. Colorado State Univserity.
[5] de Maagd, R.A. 2015. Chapter 20: Bacillus thuringiensis- Based Products for Insect Pest Control. Principles of Plant-Microbe Interactions. Springer International Switzerland, 2015. 185-192.
[6] Finucane, M.L. & J.L. Holup. (2005). Psychosocial and cultural factors affecting the perceived risk of genetically modified food: an overview of the literature. Social Science & Medicine, 60, 1603 – 1612.
[7] Gassmann, A.J. J.L., Petzold-Maxwell, E.H. Clifton, M.W. Dunbar, A.M. Hoffmann, D.A. Ingber, and R.S. Keweshan. (2014). Field-evolved resistance by western corn rootworm to multiple Bacillus thuringiensis toxins in transgenic maize. PNAS, 111(14), 5141-5146.
[8]Hansen Jesse, L.C. & J.J. Obrlycki. (2000). Field deposition of Bt transgenic corn pollen: lethal effects on the monarch butterfly. Oecologia, 125, 241 – 248.
[9] Ibrahim, M.A., N. Griko, M. Junker, and L.A. Bulla. 2010. Bacillus thuringiensis: A genomic and proteomics perspective. Bioengineered Bugs 1:1, 31-50.
[10] Jurat-Fuentes, J.L. "What Is Bacillus Thuringiensis (Bt)?" Bt Mode of Action. The University of Tennessee Institute of Agriculture. Web. <http://web.utk.edu/~jurat/Btresearchtable.html>.
[11] Petras, S.F. and L.E. Casida. (1985). Survival of Bacillus thuringiensis Spores in Soil. Applied and Environmental Microbiology, 59, 1496 – 1501.
[12] Uzogara, S.G. (2000). The impact of genetic modification of human foods in the 21st century: A review. Biotechnology Advances, 18, 179 – 206.