Rhizosphere Interactions: Difference between revisions
No edit summary |
|||
Line 102: | Line 102: | ||
====Ectomycorrhizae==== | ====Ectomycorrhizae==== | ||
[[Image:ectomycorrhizae.gif|thumb|200px|right|Ectomycorrhizae [http://www-mykopat.slu.se/Newwebsite/mycorrhiza/kantarellfiler/bilder/C.GIF Reference to Source of Image]]] | [[Image:ectomycorrhizae.gif|thumb|200px|right|Ectomycorrhizae [http://www-mykopat.slu.se/Newwebsite/mycorrhiza/kantarellfiler/bilder/C.GIF Reference to Source of Image]]] | ||
Ectomycorrhizae colonate about 3 percent of plant seeds. These fungi interact with the plant roots so that they can obtain sugars because they are poor degraders of cellulose and other plant wall material. The way they interact with the plant root is purely extracellular. They interact by producing a net-like structure called a hartig net that weaves between the root cortical cells. A sheath or mantle of fungal tissue covering part or all of the root is responsible for most of the increase in surface area caused by mycorrhizae [19]. Some fungi, such as ''[http://en.wikipedia.org/wiki/Boletus Boletus] betulicola'', have only a narrow range of plants they can associate with. Other fungi have a much larger range of host plants. One such fungus, ''[http://en.wikipedia.org/wiki/Pisolithus_tinctorius Pisolithus tinctorius]'', associates with 46 tree species and eight genera. | Ectomycorrhizae colonate about 3 percent of plant seeds. These fungi interact with the plant roots so that they can obtain sugars because they are poor degraders of cellulose and other plant wall material. The way they interact with the plant root is purely extracellular. They interact by producing a net-like structure called a hartig net that weaves between the root cortical cells. A sheath or mantle of fungal tissue covering part or all of the root is responsible for most of the increase in surface area caused by mycorrhizae [19]. Some fungi, such as ''[http://en.wikipedia.org/wiki/Boletus Boletus] betulicola'', have only a narrow range of plants they can associate with. Other fungi have a much larger range of host plants. One such fungus, ''[http://en.wikipedia.org/wiki/Pisolithus_tinctorius Pisolithus tinctorius]'', associates with 46 tree species and eight genera [27]. Although these fungi generally have a positive symbiosis with plants, some evidence is showing that they are becoming invasive to non-native An example is ''[http://en.wikipedia.org/wiki/Amanita Amanita muscaria]'' associating with ''[http://en.wikipedia.org/wiki/Nothofagus Nothofagus]''. | ||
====Arbuscular Mycorrhizae==== | ====Arbuscular Mycorrhizae==== |
Revision as of 21:29, 12 March 2016
Rhizosphere
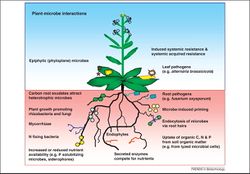
The rhizosphere is a microecological zone in direct proximity of plant roots. It is often operationally defined as the soil that clings to roots after being gently shaken in water. The actual extent of the rhizosphere is dependent on the zone of influence of the plant roots and associated microorganisms. This area of soil is considered to be the most biodiverse and dynamic habitat on Earth (Hinsinger, 2009) and The rhizosphere is also a metabolically busier and more competitive environment than the surrounding soil, which is known as bulk soil. Unlike the soil associated with the rhizosphere, bulk soil is not penetrated by plant roots and typically have lower microbial communities within it. Contrastingly, the rhizosphere is an area heavily influenced by microorganisms that feed on the compounds exuded by the roots within the soil. Additionally, displays of symbiotic relationships between plants and microorganisms are shown when plant roots release nutrients such as sugars and amino acids through water soluble compounds that sustain and provide for microbial existence. The rhizosphere is by no means simple, and understanding the way it works will greatly enhance the way people view plant ecology and soil science as a whole.
Physical Environment
Plant roots have strong effects on the physical environment of the rhizosphere. Shifts in the physical environment, brought about by root manipulation, have significant impacts on the availability and form of substrates used for microbial metabolism. In turn, this strongly influences the prevalence of different microbial functions, as well as their total biomass within the rhizosphere. This interplay goes both ways, as manipulation by microbes also plays a strong role in the ways that plant roots interact with, benefit from, and influence the soil environment around them.
Water Relations
The availability of water is a major component in determining the diversity and prosperity of soil organisms. Water availability is quantified by the water potential, which describes the transport and energy potential state of water in different forms and locations [21]. Plant transpiration, via leaf stomata, are a mechanism for connecting the soil-plant-atmosphere continuum that is driven by a vapor pressure gradient between two physical locations (I.E. the plant root and surrounding soil or the leaf interior and the atmosphere). As water transpires across the leaf surface, tension is created within the leaf that is ultimately transferred down to the root system via adhesion and cohesion of water through the vascular tissues of the plant. This can drastically alter the water potential of the rhizosphere, resulting in large fluctuations in the availability of water and solutes for microbes. This fluctuation is dramatically greater than that of the bulk soil, where changes in water potential are driven predominantly by evaporation and occur at much slower rates [21]. This fluctuation in available water puts a selective pressure on the diversity and total biomass of microbes in the rhizosphere, by selecting for those with better mechanisms for water uptake [27].
On average, most soils consist of about 50% pore space, composed of air and water with differing concentrations of solutes [25]. The constituents of the soil solution provide the substrates necessary for microbes to thrive, and ultimately determine the types of biotic and abiotic interactions taking place. Within the rhizosphere, solute activity is significantly higher than that of the bulk soil. One reason is that plant roots secrete ions and organic compounds that interact with organisms and other molecules in the environment around them. Additionally, plant roots uptake solutes in the soil solution, causing changes in ion concentrations and helping drive the rate and direction of chemical processes in the rhizosphere. As a result, the bolstered microbial population of the rhizosphere carry out enzymatic processes, such as transformation of carbon and nitrogen species, at higher rates than in the bulk soil [21].
Soil Physical Properties
The relative ratio of sand, silt, and clay have large impacts on the availability of water, air, and nutrients for microbial functions. The movement of roots through the soil profile occurs more rapidly in sandy soils relative to high clay soils. Additionally, sand has larger pore spaces between granules, allowing nutrient and water percolation to occur more rapidly. Increased porosity also allows for plant secreted compounds to extend further away from the root membrane. Therefore, the larger the granule size, the further the rhizosphere and microorganisms associated with it will extend into the surrounding soil. Larger granules require, however, that microbes travel further distances to attain nutrients or engage in interactions with plant roots. This is especially true in soils with very low water potential [27].
Additionally, soil structure of the rhizosphere is also affected by the increased interactions of microorganisms. In addition to the binding effects of plant mucilages, other root carbon depositions also stimulate soil bacteria biofilm production. These biofilms are composed of extracellular carbon polymers that act as an aggregating mechanism for mineral particles and organic matter. The rate and composition of bacterial exopolymers is highly dependant on the composition of root-secreted compounds, but have, in general, been shown to be significantly higher in the rhizosphere than in bulk soil [12].
Mycorrhizal fungi have also been shown to increase aggregation through physical constriction by fungal hyphae, as well as by secretion of their own exopolymer deposition. Further, the sorptive forces of plant roots and mycorrhizae provide an attractive force the promotes mineral alignment and provide a “backbone” for stabilizing aggregate surfaces [12]. In addition, the decomposition of roots and hyphae also increases soil aggregation by the delivery of new organic material, as well as producing larger pores and preferential flow pathways for air and water. The relationship of roots with associated mycorrhizae can alter both the decomposition rate as well as the final content of the decomposition products [25].
Soil Chemistry
The effects of pH extend to almost every characteristic of soil and are affected by many interactions within the rhizosphere. An example is respiration, which leads to carbon dioxide (and eventually to bicarbonate/carbonic acid) generation [25]. In addition to respiration of the roots themselves, the carbon-rich environment of the rhizosphere promotes high levels of respiration by other macro and microorganisms, to a far greater extent than is occurring in the bulk soil. Increased microbial activity also increases microbial transformations of chemical species. These transformations often result in increased acidity, such as nitric acid production during nitrification and sulphuric acid production during sulphur reduction [25]. The influencing effects of pH in the rhizosphere are critical in supporting a biologically diverse microbial community. Manipulation of the pH by plant roots is constantly occurring and, while it is commonly believed that plants manipulate the soil pH in order to change the availability of nutrients, it also undoubtedly influences the abundance and diversity of associated microorganisms [12].
Microbes are also essential in performing reduction-oxidation (redox) reactions in soil. These redox reactions provide energy for the microbes, while also changing the valence of ions in solution. The changes in valence are important for plants, which require essential nutrients to be in bioavailable, often inorganic forms in order to be absorbed [25]. Increases in microbial populations, associated with the rhizosphere, also result in increased redox reactions. Additionally, a study by Yang & Crowley showed that variations in different iron valence concentrations resulted in quantitative differences between rhizosphere microbial populations [12]. While we have been aware of how the rhizosphere promotes increased microbe populations for some time now, we are only beginning to understand how the redox state of various ions are affecting these same populations.
Rhizodeposits and microbial exopolymer secretion also increase the amount of molecular chelation and complexation in the rhizosphere compared to the bulk soil. These complexes provide different functions, depending on the composition. Some of these functions include reducing or increasing solubility, altering the charge, and reducing toxicity of molecules [25]. The changes in solubility are essential for allowing plant roots and mycorrhizae access to highly immobile nutrients, such as phosphate and iron.
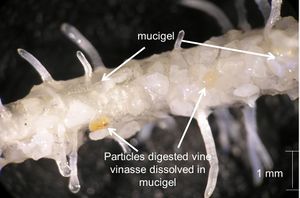
Plant-Derived Compounds
Plant-derived compounds are responsible for providing the additional carbon that allows the rhizosphere to host a large variety of organisms. Different species of plants appear to favor production of different types of polymers. A study by Small et. al. found the rhizosphere populations of canola and potato were much more similar to each other than to strawberry [1]. These compounds fall into five categories: exudates, secretions, mucilages, mucigel, and lysates.
Exudates include surplus sugars, amino acids, and aromatics that diffuse out of root cortex cells into the intercellular space (apoplast) and surrounding soil. They comprise the largest category of root secretions and are therefore considered to have to broadest effect in rhizosphere manipulation. The concentration of exudates are magnitudes greater closer to the root, as the microbial community removes the compounds faster than the root can replenish them [10]. The root controls the rate of exudate release into the rhizosphere through production of solute-specific membrane channels. The best known examples of exudates are organic acids to increase P solubility and reduce Al toxicity [10]. Due to their diffusive nature, exudates are limited to compounds of low molecular weights [1].
Secretions are byproducts of metabolic activity, known as secondary metabolites. Because they are released from the cell via active transport, secretions can be of both low and high molecular weight. They are also important in increasing mobilization of insoluble compounds, such as Fe and P. However, their importance and release mechanisms are less studied than other plant-derived compounds [10].
Lysates are generally described as all the contents of a cell once the cell is lysed and the compounds are released into the surrounding soil. When an epidermal root cell dies and is broken open, lysates from within the cell become available to the surrounding microbial community. These components include proteins, nucleic acids, lipids, and various forms of carbohydrates [14]. These compounds are important in effective the C/N ratio of soil organic matter. Due to the nature of their release, plants have considerably less control over the concentration of these compounds in soil [10].
Mucilages are cells sloughed off the root cap as the root grows. Abrasive forces of the root against soil particulate matter is responsible for the removal of cells. These cells consist of cellulose, pectin, starch, and lignin. These compounds are often highly recalcitrant and therefor contribute to higher diversity of carbon decomposers [14].
Mucigel is a slime coating the surface of a root that increases the connectivity between plant roots and the surrounding soil. It is more common on the main body of the root and root hairs than the tip. During very low soil water potential, mucigel is responsible for allowing plants to continue the uptake water and nutrients, as well as reducing the negative effects of desiccation [27].
Microbial Communities
Firstly, the rhizosphere can be seen as a competitive environment for a diverse range of microorganisms that inhabit it. Various microorganisms compete with each other for resources such as nutrients, space, and water. Some may even develop more close associations to outcompete their competitors [18].
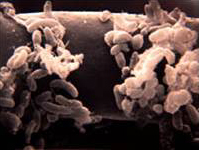
Bacteria
Bacteria are the most numerous organisms in the soil. Accordingly, studies suggest that proteobacteria, actinobacteria, non-sporulating rods, pseudomonads, and actinomycetes are among the most dominating populations of bacteria within the rhizosphere [27]. Due to their small mass, they only account for a small amount of the biomass within soil. Metabolites released from the root hairs of the plants act as chemical signals for bacteria to actively move towards the root surface whereby they can obtain nutrients [18]. Additionally, bacteria known as plant growth promoting rhizobacteria(PGPR) colonize roots very efficiently. They perform important functions to satisfy plant health and growth in various ways. Furthermore, symbiotic relationships with plants leads to benefits for both the plant and PGPR. Such benefits that plants directly receive include better nutrient uptake and stimulation of root growth [2].
The most common genera of bacteria are the following: Agrobacterium, Mycobacterium, Alcaligenes, Cellulomonas, Micrococcus, and Pseudomonas.
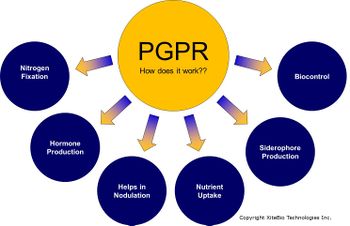
Fungi
Both pathogenic and symbiotic fungi associate with the rhizosphere, however there are many factors such as plant development and soil type that determine what specific fungal communities live within it. In particular, root exudates have been deemed an important factor when selecting for specific rhizosphere fungi (Buee). Zygomycetes and hyphomycetes establish the most readily in the rhizosphere because they metabolize simple sugars [27]. Pythium and Aphanomyces are pathogenic fungi that cause diseases to plant roots. Furthermore, fungi such as Gibberella form a symbiotic relation with plant roots and stimulates their growth. In times of flooding, when too much moisture accumulates around the base of the tree, a bacterial pathogen named Armillaria may infect and cause root rot.
Archaea
Archaea were initially viewed as extremophiles, but they now have been found in a broad range of environments that include soils, oceans, and even skin. In comparison to bacteria, there is less known information about the interactions archaea has with soil, but what we do know is that they are very important for the process of ammonification within the rhizosphere (Buee). Along with ammonification, the process of transforming organically bound nitrogen of microbial/plant biomass to ammonia, archaea also play a major role in both the carbon and nitrogen cycle.
Interestingly, current research done on rhizosphere archaea state that they are heavily associated with the major plant crop, rice. Much of the global methane gas emissions in the world come from rice paddies and are due largely in part to the large community of methanogenic archaea producing the methane gas. And while soil in rice paddies tend to be anaerobic, oxygen is able to diffuse into roots and create oxic conditions on the root surface and adjacent rhizosphere soil [2]. Accordingly, it is the oxygen-insensitive enzymes along with the DNA repair mechanisms archaea possesses that allows them to thrive in oxidized rhizosphere rice soils. Therefore under specific conditions such as high CO2 and or reduced oxygen, they increase abundantly and become major contributors to processes within the rhizosphere.
Biotic Interactions in the Rhizosphere
The main types of organisms that interact with the plant in the rhizosphere are rhizobial bacteria, fungal mycorrhizae, and endophytes (fungal/bacterial symbionts that reside within plant cells). Some of the main benefits that microbes in the rhizosphere provide to the plant are nitrogen fixation, increased phosphorus uptake, and defense against pathogens. In return, plants provide photosynthetic carbon to the organisms, in which they provide 15-20% to rhizobial nodules, 4-20% to mycorrhizae, and about 15% released to the rhizosphere. A range of interactions are present in the rhizosphere: from beneficial symbiotic relationships to detrimental pathogenic interactions. (Sylvia, 2005). However, with symbiosis comes microbes who contribute less than others to maintain plant health, although they gain the benefit from the cooperation from other organisms. These organisms are called cheaters, and there is current research being done on how plants choose their partners knowing that cooperation will exist. Plants give recognition signals to potential microorganisms for symbiosis; however, these signals are not completely accurate as they can also attract rhizobia and mycorrhizae that can potentially cheat. In a recent study on the sanctioning hypothesis, soybean plants living in a mutualistic environment with rhizobia were grown in an Ar:O2 atmosphere that was very low on N2, that significantly limited the rhizobia from fixing nitrogen. As a result, the plant immediately restricted its oxygen influx to rhizobia as a means of punishment, which inhibited further growth of the bacteria since they are aerobic.
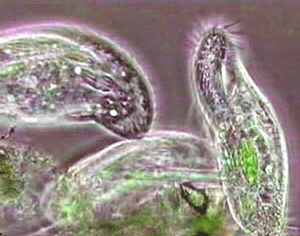
Impact of Rhizobial Microbes on Plants
Plants secrete secondary metabolites that inhibit certain effective microbes, which can either be beneficial to the plant (by pathogen suppression) or detrimental to the plant (by competition for nutrients), On the other hand, microbes can be beneficial to the plant by either promoting plant growth by providing essential macronutrients (N, P) or by inhibiting the activity of pathogenic microbes, while pathogenic microbes can be harmful to the plant by either directly infecting the plant through releasing toxins or by infecting the beneficial microbes around them. Microorganisms in the rhizosphere contribute both chemical and physical modifications that directly affect plants. Chemical changes occur as a result of the microbial decomposition of organic matter in which the conversion of organic matter and microbial biomass to mineral compounds (ammonium) provides plants with a form of nutrients that are readily available for uptake. For example, mycorrhizal fungi are responsible for aiding in water and nutrient uptake, as well as phosphorus, manganese, and copper. Rhizobial bacteria are responsible for reducing manganese (Mn4+ to Mn2+) and iron (Fe3+ to Fe2+) which is available for plant uptake. Manganese and iron are vital nutrients that play a large role in lignin synthesis, which increases resistance of plant pathogens to plant roots. Mycorrhizal fungi are responsible for water and nutrient uptake, especially phosphorus, zinc, and copper, as well as increasing soil aggregation. In tropical environments where phosphorus is deficient, these fungi are involved in Phosphorus fixation, which retrieves phosphorus deep in the soil profile while the rhizobial bacteria solubilize the mineral phosphorus for plant uptake. Furthermore, the fixation of atmospheric dinitrogen by both asymbiotic and symbiotic bacteria results in increases in the available nitrogen pool that can be accessed by plants in the rhizoplane, resulting in the continual release of nutrients to the plant roots. Physical changes occur primarily through the production of extracellular polymeric substances, such as polysaccharides and glomalin, which improve soil aggregation and soil texture. The presence of mucigel in the rhizoplane is crucial to the water relation of plants since the hydrated compound acts as a protective, lubricative layer for the root cap that aids in the uptake of water. Without this compound, plants would have a higher chance of suffering from times of high water stress since it would be difficult for plants to efficiently penetrate the soil and form a symbiotic relationship with fungi that aid in water uptake. In addition to the soil texture and organic matter content, the variety, age, and health of the plants play a large role in both the composition and dynamics of microbial activity in the rhizosphere.
In addition to soil properties and the types of microorganisms present in a soil, plants play an important role in the community diversity of rhizosphere microorganisms. Plant roots cause chemical and physical changes to the soil they inhabit and these changes will affect the microbial diversity in and around the rhizosphere. Root exudates will select for/against certain populations of microorganisms. Many plants exhibit genetic resistance/tolerance to rhizosphere microorganisms; the variety of plant will determine, in part, the community makeup of the microorganisms in the rhizospere. The ability of a plant to form symbiotic relationships with soil microbes will also determine rhizosphere microbial populations. The age and health of the plants present will also play a role in the microbial community dynamics of the rhizosphere. Plant roots increase the tilth of a soil and subsequently affect the physical properties of the soil.
Plants can also compete with rhizosphere microorganisms for resources like water and nutrients. (Sylvia, 2005)
Symbiotic or Mutualistic Relationships
In a relationship, the plant usually provides a source of carbon and the bacteria or fungus fills some other more specialized function. In many cases the association is not absolutely necessary for the survival of both members, but provides significant benefit.
The recently sequenced genome of Laccaria Bicolor (an ectomycorrhizal fungus) shows a excess of enzymes used in ammonia uptake, and a lack of enzymes that would be needed to degrade plant wall material. Thus, it is assumed the fungus is providing nitrogen (and perhaps other nutrients), and a guarantee not to attack to the tree in return for glucose. (Martin, 2008). As described below, fungi are also often an important source of phosphorous.
The microbial partner can also help the plant to survive in an otherwise inhospitable environment. For example, specific endophytes have been shown to confer heat resistance to grasses that grow in a hydrothermal area, or salt tolerance to costal grasses. (Rodreguez, 2008)
Mycorrhizal Fungi
Over 80 percent of all land plants have a mutualistic relationship with one or more mycorrhizal fungi. Mycorrhizal fungi extend the effective root length by 100 fold or more. Not only do they increase the surface area over which nutrients and water can be taken up by the plant, but fungal hyphae can also reach into smaller pores than roots can. The most valuable asset mycorrhizae provide is accessing immobile nutrients, such as phosphorous. In return, the plant passes a significant amount of its carbon reserves to the fungi. (Sylvia, 2005) Although, mycorrhizal fungi are beneficial for the most part, they can also be mildly detrimental to the plant, or have circumstances in which the plant feeds from the fungi.
TYPES OF MYCORRHIZAL FUNGI:
Orchid Mycorrhizal Fungi
An example in which mycorrhizal fungi and plants are beneficial to each other are seen in the symbiosis they have with orchids. For a small stage in the orchids life, it depends of fungi to give it sugars. Orchid seeds cannot germinate unless they have fungus that are attached. In return for its sugars, the orchid gives the fungi trace elements. Although this positive interaction is seen, the mycorrhizal fungi do not benefit completely. The plant parasitises the fungus that invades it. [5]
Ectomycorrhizae
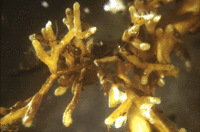
Ectomycorrhizae colonate about 3 percent of plant seeds. These fungi interact with the plant roots so that they can obtain sugars because they are poor degraders of cellulose and other plant wall material. The way they interact with the plant root is purely extracellular. They interact by producing a net-like structure called a hartig net that weaves between the root cortical cells. A sheath or mantle of fungal tissue covering part or all of the root is responsible for most of the increase in surface area caused by mycorrhizae [19]. Some fungi, such as Boletus betulicola, have only a narrow range of plants they can associate with. Other fungi have a much larger range of host plants. One such fungus, Pisolithus tinctorius, associates with 46 tree species and eight genera [27]. Although these fungi generally have a positive symbiosis with plants, some evidence is showing that they are becoming invasive to non-native An example is Amanita muscaria associating with Nothofagus.
Arbuscular Mycorrhizae
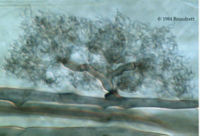
Arbuscular mycorrhiza are also referred to as endomycorrhizae because it’s branched arbuscule grows within the root cortical cell. Direct connection between the plant and fungal cytoplasm allows the transfer of nutrients from the fungi to the plant and carbon from the plant to the fungi to be more efficient. Examples of mycorrhizal fungi include Glomus tenue and Scutellospora. (Sylvia, 2005) These types of fungi have many benefits on the plant performance and on the health of the soil. For example, by making up 50 percent of fungal mycelium in soil, which is a bulk of the soil mass, they help the soil structure. They keep structure and aggregates together. These fungi release a substance called glomalin which is sticky and holds the soil together as well, further increasing its stability. The way in which the performance of the plant is increased is by allowing it to uptake phosphorus more efficiently. Because AM creates a larger surface area, they can have more access to phosphorus. Because phosphorus is easily taken up, it reduces the amount of fertilizer needed to be applied. This ultimately reduces the amount of eutrophication in the water, therefore decreasing pollutants. Studies have also shown that arbuscular mycorrhiza are beneficial in protecting soil born pathogens, therefore allowing them to grow stronger, and healthier. [5] [7] [28]
Nitrogen Fixing Bacteria

Many organisms have the ability to fix nitrogen but not many of these actually do. About 86 species in 2 genera of archaea, 38 genera of bacteria, and 20 genera of cyanobacteria that can fix nitrogen. This ensures that any nitrogen lost can be replenished. Nitrogen fixation is a very important process in soil, as it benefits the plant in many ways. When the nitrogen is being fixed by bacteria it is directly benefiting the plant by making ammonium that the plant can then utilize to make things such as amino acids and proteins. Another way in which the plant acquires nitrogen is when the nitrogen fixing bacteria dies, and releases an abundant amount of nitrogen into the environment that the plants can then utilize. Nitrogen fixers not only benefit their symbiont, but can benefit other plants that are not involved in the symbiotic relationship. Some nitrogen can be leaked and taken up by other plants. Most of the nitrogen is recycled into the soil. As legume plants decompose and die. Due to the high energetic cost of fixing dinitrogen, a significant part of nitrogen fixation occurs near the plant roots, where there is an influx of sugar to power the process. Some nitrogen fixation occurs in the rhizosphere by free-living bacteria (the ability is found only in prokaryotes). When these otherwise free living bacteria form a close association with a plant they’re called “associative symbiotes”. An example of a free living nitrogen fixer is Bradyrhizobium. There are several drawbacks to this lifestyle. For example, Nitrogen fixing bacteria are sensitive to fluctuations in oxygen. Although many are aerobes who use oxygen as a terminal electron acceptor, for some oxygen can poison the nitrogenase enzyme. Free-living bacteria need to take energetically costly steps such as having extremely fast respiration or producing large amounts of slime to protect nitrogenase. Another disadvantage associative symbiotes have to deal with is direct competition with the rest of the rhizosphere for the available carbon. Experiments indicate that carbon is a limiting factor in rhizosphere nitrogen fixation. An alternate form of nitrogen-fixing lifestyle is to actually live in the plant root cells themselves. Bacteria who do this are called “mutualistic symbionts.” An example of one such bacteria is Rhizobia. These bacteria form nodules on plants, which are huge masses of bacteria living inside plant cells that have been modified for this purpose. The process of forming a nodule requires recognition between bacteria and plant (usually very specific), and gene expression changes in both players. The plant provides carbon to the bacteria and the bacteria provide fixed nitrogen to the plant. The masses of bacteria produce leghemoglobin to protect nitrogenase from oxygen. Leghemoglobin gives the nodules a pink color, which is an indication that nitrogen fixation is occurring. If the nodule is white or gray in color, the nodule is fixing very small amounts of nitrogen or none at all. Much like the hemoglobin in the human body, leghemoglobin binds to oxygen to its subunits and holds onto it so that it cannot be used. (Sylvia, 2005).
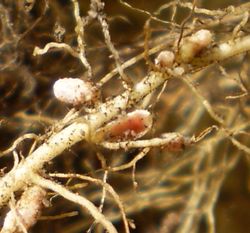
Legume nodules
The nodule is formed by rhizobia bacteria entering the cell root and making nodules. These nodules are small in size. These nodules can be see after 2-3 weeks of growing.These are the type of nodules in which the pink color given by the leghemoglobin can be indicative of nitrogen fixation occurring. When a legume is no longer fixing nitrogen it will usually turn green and the plant will get rid of it.
Perennial legume nodules
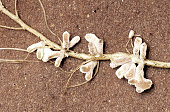
These kinds of nodules are found on alfalfa and clover. They are about half an inch in diameter.They are also long and have a fingerlike shape. When these modules are mature, they have a larger center with many finger like protrusion. These nodules grow mostly around the tap root and with live for quite a long time.
Annual legume nodules
These nodules can be found on beans, peanuts, and soybeans. They’re spherical and are pea -size. Unlike the perennial nodule, these nodules are short-lived. When plants are filling their pods, the nodules will not fix nitrogen. This is because the plant is too busy giving its nutrients to the seeds instead of the nodule.
Frankia
Frankia are actinomycete filamentous bacterium that form nodules. These nodules inhabit the roots of actinorhizal plants such and shrubs and trees. The process used for Intracellular infection by Frankia is quite complex. Frankia and plants use specific signals that activate a symbiotic signaling pathway. Research today is not clear what the biochemical characterizations are of Frankia, but have found that Flavonoids improve nodulation and are necessary for the infection. Recent genome sequencing has allowed scientists to theorize how and why prokaryotes and plant symbioses occurred, adaptations, metabolic diversity, and horizontal gene flow among symbiotic prokaryotes. [6]
Inoculants
Inoculation of fields with the soil from other fields has been practiced for centuries as a method of inoculation. In addition, many commercial microbial inoculants are now sold on the consumer market. As organic foods and farming is becoming more popular, use of beneficial microbial and fungal inoculants has increased substantially. [13] Modern seed companies are dipping seeds in beneficial inoculants before sale. However, the specific conditions surrounding the seed at germination and the specific soil characteristics determining the ability of the soil to support a population of desirable rhizosphere microbes will be the ultimate determinants in the successful colonization of the desired inoculant. The unsustainability of synthetic fertilizers and pesticides has been exhibited in recent years, calling for research and transition to biofertilizers and biopesticides. However, there is still concern over uncertain affects of transplanting microorganisms including the effects it may have on native ecosystems. Currently, the primary organisms being used for these products are mycorrhizal fungi, dinitrogen-fixing bacteria, and beneficial rhizobacteria. [27]
Ongoing Research
The rhizosphere is a complex ecosystem made up of numerous communities. Its diversity and benefits have yet to be fully understood. Research continues to demonstrate the influence below-ground communities have on their above ground partners. All studies below have been published between 2008 and 2016.
■ Arbuscular mycorrhizal fungi enhance tolerance of vinca to high alkalinity in irrigation water. Cartmill and colleagues found that arbuscular mycorrhizal fungi are capable of increasing the salt tolerance of plants. Arbuscular mycorrhizal fungi (AMF) inoculated plants and non-AMF inoculated plants were irrigated with varying HCO3- concentrations. Results demonstrated that non-AMF inoculated plants could tolerate and produce quality plants at less than or equal to 2.3 mM HCO3-. However, AMF inoculated plants could produce quality plants at 7.5mM HCO3-. Applications for this finding include increasing the tolerance of crops to irrigation water of high alkalinity. [3]
■ The interaction between iron nutrition, plant species and soil type shapes the rhizosphere microbiome. Plants are able to alter microbial habitat by creating small chemical niches via root-secreted phytochemicals. In this paper, Pii et a.l studied developmentally distinct plants that obtain nutrients in different ways (tomato and barley), and respective root exudates’ effects on the surrounding rhizosphere. In addition, they included iron free nutrient solution treatments to induce iron starvation. They found that when nutritionally starved, both barley and tomato compensate by excreting root secretions selecting for plant growth promoting microbes despite distinct strategies for nutritional acquisition. Their findings are applicable to nutritional stress that often curtails productivity in agriculture. [22]
■ Glyphosate effects on soil rhizosphere-associated bacterial communities. Glyphosate is the most widely used herbicide in agriculture. There are many studies that have investigated short-term effects of glyphosate on the rhizosphere, however there is little known about its long term effects. Newman et al. studied its effect over 3 years and found Acidobacteria in soy and corn rhizospheres decreased in response to long term glyphosate exposure. Because Acidobacteria plays an important role in several biogeochemical processes, the authors speculated that their decrease can alter the nutritional status of soil. These findings call attention to the need for additional long-term studies on the effects of glyphosate. In addition, it highlights agricultural dependence on pesticides and the need for alternative approaches to pest reduction.
■ Rhizobacteria isolated from common bean in southern Italy as potential biocontrol agents against common bacterial blight. Common bacterial blight, caused by Xanthomonas axonopodis is able to devastate acres of crop. Bactericides are limited thus alternative solutions have been studied in recent years. After analyzing 162 bean rhizobacteria from southern Italy, Giorgio et al. found 6 rhizobacteria were able to reduce pathogenicity and symptoms in lab assays. Further study of these rhizobacteria could potentially lead to new and sustainable biocontrol methods. [17]
■ Water management impacts on arsenic behavior and rhizosphere bacterial communities in a rice agro-ecosystem. Rice agriculture is unique due to specific flooding techniques and management. Arsenic at high levels may cause health concerns and because rice is grown in water, it has a higher chance of being contaminated with arsenic. Arsenic is transformed into plant roots when competition in the rhizosphere and lack of As- reducing/oxidizing microbes causes mobilization of As- to plant roots. As- reducing and oxidizing bacteria can regulate accumulation and speciation. However there are few studies of how flooded non-flooded rice agriculture systems affect the rhizosphere in relation to arsenic. Das et. al found that alternating wetting and drying cycles rather than having constant flooded or dry conditions was best for reducing levels of arsenic. This is due to the decrease in As-, Fe- and sulfur-reducing bacteria and an increase in of As-, Fe- and sulfur-oxidizing bacteria in the rhizosphere soil. Rice is an economically and nutritionally important crop and arsenic contamination is an increasing concern. This study highlights the importance of management techniques in relation to microbial activity. [4]
■ Effects of the soil microbial community on mobile proportions and speciation of mercury (Hg) in contaminated soil. Mercury contamination has been am unceasing concern due to its toxicity and its increasing accumulation in our food sources. Szakova et al. recently investigated mercury resistant bacterial strains found in mercury contaminated soils included Paenibacillus alginolyticus, Burkholderia glathei, Burkholderia sp., and Pseudomonas sp. This study assessed the ability of the bacterial strains to deplete mobile and mobilizable mercury. After exposure to soluable HgS in microbial agar, gas chroatogrphy was used to determine organomercury compounds in the microbes. It was found that Paenibacillus sp. Made up 42% of the microbial community after HgS exposure, suggesting its importance in the Hg transformation process. Szakova et al. will be further this research by testing Paenibacillus sp. for bioremediation potential. [28]
■ Plant-growth-promoting rhizobacteria: drought stress alleviators to ameliorate crop production in drylands. Drought is the top constraint to agricultural productivity. Plant growth promoting rhizobacteria (PGPR) have demonstrated success in mitigating the impacts of drought. This is done by biochemical and physical changes including the production of heat shock proteins, dehydrins, volatile organic compounds, and modifications of phytohormonal levels. Kaushal and Wani is currently screening PGPRs applicable to help crop productivity limits attributed to drought and drylands. [11]
References
[1] Bakker, Peter A. H. M., Roeland L. Berendsen, Rogier F. Doornbos, Paul C. A. Wintermans, and Corné M. J. Pieterse. “The Rhizosphere Revisited: Root Microbiomics.” Frontiers in Plant Science 4 (2013). doi:10.3389/fpls.2013.00165.
[2] Bonkowski, M. et al. “Rhizosphere fauna: the functional and structural diversity of intimate interactions of soil fauna with plant roots.” link.springer.com. Plant and soil, 2009. Vol 321. 20 June. 2009.
[3] Cartmill, A. D., Valdez-Aguilar, L. A., Bryan, D. L., & Alarcón, A. (2008). Arbuscular mycorrhizal fungi enhance tolerance of vinca to high alkalinity in irrigation water. Scientia Horticulturae, 115(3), 275-284.
[4] Das, S., Chou, M., Jean, J., Liu, C., & Yang, H. (2016). Water management impacts on arsenic behavior and rhizosphere bacterial communities and activities in a rice agro-ecosystem. Science of The Total Environment, 542, 642-652.
[5] Deacon, J. (n.d.). He Microbial World: Mycorrhizas. Retrieved March 12, 2016, from http://archive.bio.ed.ac.uk/jdeacon/microbes/mycorrh.htm
[6] Frankia Home. (n.d.). Retrieved March 12, 2016, from http://web.uconn.edu/mcbstaff/benson/Frankia/FrankiaHome.htm
[7] Gianinazzi, S., Gollotte, A., Binet, M., Van Tuinen, D., Redecker, D., & Wipf, D. (n.d.). Agroecology: The key role of arbuscular mycorrhizas in ecosystem servi. Retrieved March 12, 2016, from http://link.springer.com/article/10.1007/s00572-010-0333-3
[8] Giorgio, A., Cantore, P. L., Shanmugaiah, V., Lamorte, D., & Iacobellis, N. S. (2015). Rhizobacteria isolated from common bean in southern Italy as potential biocontrol agents against common bacterial blight. Eur J Plant Pathol European Journal of Plant Pathology, 144(2), 297-309.
[9] Hinsinger, P., Bengough, A. G., Vetterlein, D., & Young, I. M. (2009). Rhizosphere: Biophysics, biogeochemistry and ecological relevance. Plant Soil Plant and Soil, 321(1-2), 117-152.
[10] Jones, D. L., Nguyen, C., & Finlay, R. D. (2009). Carbon flow in the rhizosphere: Carbon trading at the soil–root interface. Plant Soil Plant and Soil, 321(1-2), 5-33.
[11] Kaushal, M., & Wani, S. P. (2015). Plant-growth-promoting rhizobacteria: Drought stress alleviators to ameliorate crop production in drylands. Ann Microbiol Annals of Microbiology, 66(1), 35-42.
[12] Kent A.D. & Triplett E.W., 2002. Microbial communities and their interactions in soil and rhizosphere ecosystems. Ann. Rev. Microbiol., 56, 211-236.
[13] Kiers, E. T., & Denison, R. F. (2008). Sanctions, Cooperation, and the Stability of Plant-Rhizosphere Mutualisms. Annu. Rev. Ecol. Evol. Syst. Annual Review of Ecology, Evolution, and Systematics, 39(1), 215-236.
[14] Lakshmanan, Venkatachalam. “Chapter Three - Root Microbiome Assemblage Is Modulated by Plant Host Factors.” In Advances in Botanical Research, edited by Harsh Bais and Janine Sherrier, 75:57–79. Plant Microbe Interactions. Academic Press, 2015. http://www.sciencedirect.com/science/article/pii/S0065229615000658.
[15] Lindeman, W., & Glover, C. (n.d.). College of Agricultural, Consumer and Environmental Sciences. Retrieved March 12, 2016, from http://aces.nmsu.edu/pubs/_a/A129/
[16] M. Buée & W. De Boer & F. Martin & L. van Overbeek & E. Jurkevitch. “The rhizosphere zoo: An overview of plant-associated communities of microorganisms, including phages, bacteria, archaea, and fungi, and of some of their structuring factors.” www.planta.cn. Plant and soil, 2009. Springer Science. 28 April. 2009.
[17] Newman, M. M., Hoilett, N., Lorenz, N., Dick, R. P., Liles, M. R., Ramsier, C., & Kloepper, J. W. (2016). Glyphosate effects on soil rhizosphere-associated bacterial communities. Science of The Total Environment, 543, 155-160.
[18] Nihorimbere V., Ogena M., Thonart P. “Beneficial effect of the rhizosphere microbial community for plant growth and health.” Vol 15, 2011. 5 May, 2010. Web. 11 March, 2016.
[19] NYBG.org: Hidden Partners: Mycorrhizal Fungi and Plants. (n.d.). Retrieved March 12, 2016, from http://sciweb.nybg.org/science2/hcol/mycorrhizae2.asp.html
[20] Osorio Vega, N. W. (2007). A review on beneficial effects of rhizosphere bacteria on soil nutrient availability and plant nutrient uptake. SciELO, 60(1).
[21] Philippot L. et al. “Going back to the roots: the microbial ecology of the rhizosphere.” www.nature.com. Nature Reviews Microbiology. 23 Sept. 2013.
[22] Pii, Y., Borruso, L., Brusetti, L., Crecchio, C., Cesco, S., & Mimmo, T. (2016). The interaction between iron nutrition, plant species and soil type shapes the rhizosphere microbiome. Plant Physiology and Biochemistry, 99, 39-48.
[23] Rillig, M. C., & Mummey, D. L. (2006). Mycorrhizas and soil structure. New Phytologist New Phytol, 171(1), 41-53.
[24] Rodriguez, Rusty J, Joan Henson, Elizabeth Van Volkenburgh, Marshal Hoy, Leesa Wright, Fleur Beckwith, Yong-Ok Kim, Regina S Redman "Stress tolerance in plants via habitat-adapted symbiosis" The ISME Journal (07 Feb 2008).
[25] Scow, K. Soil Microbiology class notes. Winter 2015, University of California, Davis.
[26] Singer, Michael J and Donald N. Munns. 2006 Soils: an Introduction. Pearson Education Inc. New Jersey.
[27] Sylvia, D., Fuhrmann,J., Hartel, P., Zuberer, D. 2005. Principles and Applications of Soil Microbiology. Pearson Education Inc. New Jersey.
[28] Száková, J., Havlíčková, J., Šípková, A., Gabriel, J., Švec, K., Baldrian, P., . . . Tlustoš, P. (2016). Effects of the soil microbial community on mobile proportions and speciation of mercury (Hg) in contaminated soil. Journal of Environmental Science and Health, Part A, 51(4), 364-370.
[29] Zahran, H. H. (n.d.). Zahran HH.. Rhizobium-Legume Symbiosis and Nitrogen Fixation under Severe Conditions and in an Arid Climate. Microbiol Mol Biol Rev 63: 968-989. Retrieved March 12, 2016
Edited by students of Kate Scow