Microbes and Animal Behavior: Difference between revisions
No edit summary |
No edit summary |
||
Line 1: | Line 1: | ||
Bella | Bella S. | ||
Microbio 238 2020 | Microbio 238 2020 | ||
==Introduction== | ==Introduction== |
Revision as of 14:44, 24 April 2020
Bella S. Microbio 238 2020
Introduction
Animal behavior can be influenced by many factors, making it an interesting yet complex field. From environmental interactions to genetics, there are many ways to look at a certain behavior. How do microbes, particularly bacteria, play a role? Can these microscopic organisms that live inside animals affect who these animals mate with or even how they communicate with conspecifics? Experiments have used genetics, such as rRNA analysis, to study the make-up of symbiotic microbial communities to further understand a certain behavior, like finding another piece to the puzzle. Because animal behavior covers a wide range of topics, from mating to communication, there are possibly many ways microbes, especially symbiotic microbes, could affect behavior in a variety of animals from hyenas to squids, and even humans. How microbes affect and interact with animals can give us insight into our own relationship with microbial organisms and help us understand our own behavior.
Symbiosis
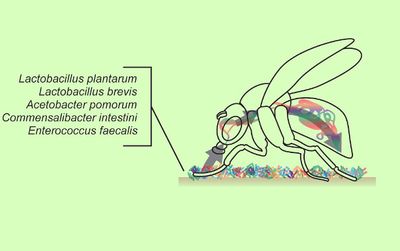
Microbe-animal interactions are often seen in the form of symbiosis, and these relationships impact various animal behaviors from mate choice to interspecies communication. These reactions range widely from parasitic to mutualistic. Marine animals in particular act as hosts for a diverse range of symbiotic microbes, with at least 7 different phyla acting as hosts for chemosynthetic symbionts. In terms of behavior and physiology, there is a diversity of reductants and oxidants that hosts provide for their symbionts. For example, motile animals such as nematode worms migrate between upper oxidized and lower reduced sediment layers to obtain oxidants and reductants. Thyasirid clams even use their feet, which extend up to 30 times the length of their shells,to form burrows, with the length and number of burrows corresponding to the concentration of hydrogen sulfide in the sediment.[1]
There are also many examples in the animal kingdom of gut microbiota influencing animal behavior. For instance, research has shown that commensal bacteria can affect mating in flies. [2] After one generation, flies preferred to mate with those that were on the same diet, either starch or molasses, and these preferences continued for at least 37 generations. This 2010 study hypothesized that the change in diet, i.e. rearing the first group of flies on either starch or molasses, altered their gut microbiome and influenced their behavior. Aside from the clear correlation between diet and mating choice, this was further confirmed by antibiotic treatment which caused the mating choices to become totally random, indicating that microbiota played a role in the previously seen preferences. Additionally, analysis of fly rRNA found that flies transferred to starch contained a much higher percentage of Lactobacillus plantarum than the flies raised on standard cornmeal-molasses-yeast (CMY), which the paper suggests influences behavior via the influencing the level of sex pheromones. Gut microbiota not only affects behavior in insects but in vertebrate animals such as mammals. A 2010 study looked at gut microbiota in mice and the effect on behavior and even brain development. Previous studies had found that exposure to some microbial pathogens in certain developmental periods resulted in behavioral abnormalities. The 2010 study hypothesized that a normal gut microbiota plays an important role in the environmental factors that modulate brain development and function.[3]
Another instance of microbes playing an important role in animal development is seen in marine animals like the squid. Microbial symbionts can either be vertically or horizontally transmitted. Developmental programs must be altered to accommodate the symbiotic relationship, and establish the correct partners and not interlopers. A 2014 review examined what is known about the symbiotic relationship between the bacteria, Vibrio fischeri, and the Hawaiian bobtail squid, Euprymna scolopes.[4] Juvenile squids are colonized in the light organ soon after hatching, the symbiont being obligate for the hosts but not dependent on the host themselves. Within hours of hatching, the juvenile squid has a colonized, bioluminescent organ. This symbiotic relationship is initiated by ventilating the surrounding seawater through the squid’s body cavity, harvesting V. fischeri while excluding other bacterial species. The cells aggregate along the ciliated epithelia, then move into the deep tissues. Finally, a few inoculating cells will fill crypts in the light organ. Vibrio fischeri are bioluminescent bacteria that produce light used by squid hosts as camouflage, an antipredator behavior also known as “counterillumination” that mimics moonlight and starlight. Researchers are also looking into the genome of Vibrio fischeri to better understand how these respiring bacteria generate energy, and what possible role they play in the global carbon cycle.[5]
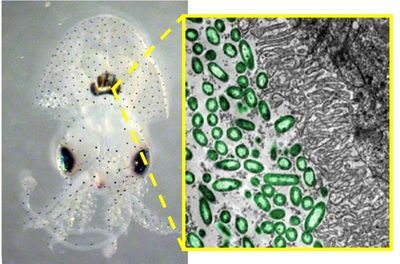
Communication
Microbes can be involved in animal communication, with the animal host utilizing microbial products as signals that communicate social rank or group membership. Also note that a signal is not the same thing as a cue. A signal is an evolved act or structure that can either benefit the signaler or receiver, or both. A cue is a feature of the environment that may be beneficial but it doesn’t evolve. There are many modes of signaling, such as chemical signals like pheromones. In order to determine if a signal is mediated by microbes, scientists must demonstrate that the chemical signal can be synthesized by the microbiota, and if elimination of the microbiota results in a loss of the behavioral trait.[6] Chemical communication comes in many forms. Some animals can synthesize their own chemical signals, while others rely on their environment or symbiotic microbes to obtain the components required for the signal.
Theis et al. (2012) studied microbial-mediated chemical communication in hyenas, specifically fermentative bacteria found in the hosts’ specialized mammalian scent glands.[7]
The study’s findings coincide with the fermentation hypothesis for chemical recognition which states that fermentative bacteria in scent glands generate odorants used by the hosts in communication and that variation in odors is due to the variation in the bacterial communities of the scent glands. A phylogenetic analysis was conducted, and showed that the hyena scent pouch microbiota was made up of mostly obligate or facultative anaerobes closely related to well-documented odor producers. Theis et al. (2012) also found group-specific bacterial communities from the hyenas’ scent pouches. One possible mechanism for this is that Spotted hyenas frequently scent mark where other members have marked as well, thus creating a possible pathway for transmission of bacterial communities among members within a clan.
Another study found that male bats (Saccopteryx bilineata) have a pouch-like scent organ in the leading wing membrane that contains an odorous liquid involved in courtship.[8] Voig et al. (2005) studied the microbial flora within these scent organs to determine if microbial degradation of scents influences a male’s mating success by affecting his scent. During courtship, female preference is important so males must attempt to court females by hovering over them, and fanning their scents towards the females. Everyday males transfer genital and glular liquids into the wing sacs. This 2005 study predicts that males repeat this process in order to minimize microbial fermentation and control the microflora in their wing sacs, which create a scent specific to the individual. This prediction was supported by their findings that microbial diversity was lower in males than females, and that individual males on average carried two microbial strands in their wing sacs.
Many mammal species are able to distinguish between different individuals by their odor. The African dwarf mongoose, Helogale undulata, uses odors produced by anal glands to communicate. Additionally, these mammals can distinguish the marks made by the anal glands of conspecifics. Specifically, the “anal pockets” of an individual has a unique concentration of various carboxylic acid products from bacterial breakdown of sedum and apocrine secretion. Gorman (1976) found that mongooses can discriminate between the anal pocket contents of other mongooses and even synthetic odors.[9]
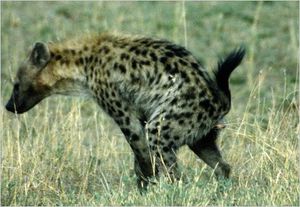
Human Applications
Understanding the ways in which microbes affect animal behavior can help us understand certain disorders in people. The gut microbiota in mammals is essential for digestion, immunity and normal host physiology. One additional and unexpected role is in brain function via the “microbiota-gut-brain axis”.
Previous research has found that animals raised without microbial colonization show abnormal behavior. And now, recent studies have seen a possible link between gut microbiota and Autism Spectrum Disorder (ASD). For instance, Vuong and Hsiao (2017) reviewed studies of microbial dysbiosis (an impaired microbiota) in ASD.[10] Features of ASD in humans include social communication deficiencies, repetitive behaviors/motor movements and other behavioral abnormalities (anxiety, seizures, hyperactivity). Studies that used mice models to examine social behavior in germ-free mice found that germ-free mice were less sociable, interacting with nonsocial objects instead of other mice or preferring to only interact with familiar mice rather than unfamiliar.[11][12] Additionally, other researchers have found that these behavioral abnormalities could be corrected in germ-free mice by postnatal colonization with a wild-type gut microbiota at weaning.[13] It has recently been found that the maternal diet is a perinatal risk factor, at least in mice studies. Buffington et al. (2016) found that maternal obesity induced by a high-fat diet in mice alters the gut microbiome in offspring, resulting in social behavioral deficits which were corrected with transfer of a gut microbiome from control mice.[14] Moreover, treatment with the gut bacteria Lactobacillus Reuters alone sufficiently restored social behaviors. The success of the gut microbiome was connected with its ability to promote hypothalamic levels of oxytocin, a hormone released in social bonding, also known as the “love hormone”, and neuron activation in the ventral tegmental area, a brain region which has many functions including emotion output from the amygdala.
Gut bacteria used as probiotics have had many health benefits in digestion. Additionally, probiotics may have a positive effect on the central nervous system, with possible applications for alleviating certain mental health issues such as anxiety. Studies have used animal models to test the effect of probiotics on certain behaviors. Research has found that certain strains of Lactobacillus have a positive effect on anxiety-related behavior and responses to stress. [15] Zebrafish (Danio rerio) have become models for studying neurobehavior, and have neurotransmitter systems translatable to humans and rodents. Zebrafish microbiota along with human microbiotas have both seen colonizations of species from Lactobacillus. One study found that zebrafish treated with Lactobacillus plantarum exhibited significantly less anxiety-related behavior compared to those untreated.[16] It also saw that L. plantarum administration correlated with an increase in GABA-A receptor of the central nervous system and a significant increase in serotonin transporter expression, as also seen in mice studies.[17] This is similar to anxiety medications (SSRIs) that work to increase serotonin and GABA transmission in the brain. This study also found that probiotic supplementation was protective against stress-induced dysbiosis of the gut microbiota in zebrafish.
Mating
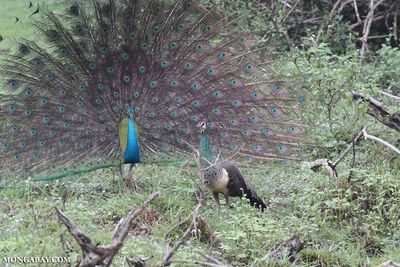
The field of animal behavior includes the study of mating and sexual selection. Sexual selection is a form of natural selection that acts on heritable traits which affect reproduction. One type, intersexual selection, involves mate choice usually by one sex more often than the other. In many species females choose their mates and are selective of that choice. Being selective has direct and indirect benefits. The main indirect benefit is that mating with a high quality male may produce offspring of the same high quality because of genetics. Certain hypotheses, such as the Zahavi handicap principle, state that exaggerated secondary sexual traits (like a male bird’s plumage) are an indicator of genetic quality because only the highest quality male, with good genotypes, can have the most exaggerated traits and overcome the handicap (such as increased predation risk due to brighter, colorful plumage, or the energy cost associated with maintaining the trait). A male bird’s plumage is an example of an ornamental trait. Having a high quality ornamental trait also indicates fewer parasites, which is similar to the Hamilton-Zuk hypothesis that states if pathogens and parasites reduce male vigor, then only males with superior disease resistance will be able to display exaggerated secondary sexual traits.
Previous studies have focused on the effect of bacterial infections on the production of ornamental traits. However, bacteria not only live inside birds but on the surface, in their feathers. Few bacteria living in feathers will have deleterious effects on their host. A diverse group of species, including keratinolytic (feather-degrading) and non-keratinolytic bacteria, may live in feathers. Studies have indicated that energy trade-offs are involved in the bacterial diversity of a bird’s feathers, as in males with larger broods having less bacterial diversity on their feathers due to the energy spent foraging instead of preening/sanitational behaviors that may prevent harmful,pathogenic bacteria from growing.[18] A 2007 study predicted that a relationship between feather color and bacteria could occur because high-quality males will have more energy than low quality males to allocate to cleaning behaviors and reducing bacterial abundance.[19] This would be beneficial, not only in maintaining plumage color and in courtship displays, but because bacterial degradation of feathers may decrease thermoregulation and defense against bacterial infections provided by feathers. However, their results found that male bluebirds (Sialia sialis) with brighter structural color/plumage had a higher abundance of overall general and keratinolytic bacterial load on their feathers. There are many possible explanations; male blue birds with brighter plumage may allocate more time and energy to reproduction than self-maintenance, some of these bacteria may be beneficial and out-compete pathogenic bacteria (similar to the role of normal flora in human skin), and/or bacteria directly alter plumage color.
Sociality
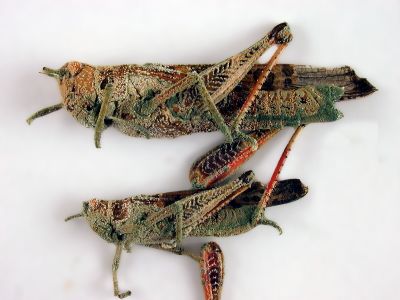
The field of animal behavior also examines sociality, group structure and organization. Once again, understanding the role that microbes play in an animal’s life, such as solitary versus social living, can be useful to people. For instance, the desert locust (Schistocerca gregaria) obtains pest status when they form large groups, or swarms. These insects have been the cause of plagues and famines throughout human history. At the time of writing this (2020) a locust outbreak is occuring in East Africa, forming swarms of as many of 50 million insects, threatening the health of the region’s livestock and livestock feed.[20] Understanding locust biology will help with the ongoing effort in creating the best, most effective control methods. Desert locusts live in solitude, as a grasshopper-like insect, and then transition to the gregarious (sociable) phase as a winged adult locust. Swarms of locusts then migrate in search of food. Conspecific communication is key to initiating this gregarious phase.
The aggregation of locusts is maintained by aromatic compounds released from locusts and their fecal pellets. In particular the phenolic pheromone compounds, guaiacol and phenol, are released from fecal pellets.[21] Research has found that these phenolic compounds originate in the gut bacteria of locusts.[22] Locusts were raised from surface-sterilized eggs on a diet of irradiated feed to ensure the colony was anexic (no other organisms, such as bacteria). Compared to the fecal pellets of normal adult and juvenile locusts, the pellets of germ-free locusts were absent of guaiacol and had reduced levels of phenol. There appears to be a moderately mutualistic relation between locusts and their gut microbiota, although it’s not obligate which is assumed because locusts do not rely on only one microbial species. Further understanding the gut bacteria and diet of locusts could reveal new methods in insect control. There have been promising methods in controlling locusts, and microbes have played a role. For instance, adult desert locusts inoculated with the fungi Metarhizium flavoviride saw reduced food consumption and died in a period of five to fourteen days (depending on the amount of conidia, spores formed by fungi).[23]
Living in groups can have many benefits, and part of studying animal behavior is understanding why certain animals live in groups. For instance, group living can reduce the risk of predation for individuals. This dilution effect means that, assuming only a single kill, the bigger the group the less probable an individual will be killed. Another benefit of group living is reducing time and energy spent on being vigilant, allowing an individual animal to spend more energy on foraging, mating, etc. Other benefits to sociality and group living include increased foraging, defense, and help raising offspring. Another possible and underappreciated benefit is increased access to mutualistic endosymbiotic microbes.
Researchers have hypothesized that endosymbiotic microbes have played an important role in the evolution of sociality in herbivores and even non-herbivores.[24] This theory has been examined in models involving the coevolution of digestive mutualisms and sociality in herbivores, specifically Dictyoptera (cockroaches, termites, mantids).[25] They postulate that this mutualism began with microbes obtained from the environment that aided host nutrition (necessary for digesting plant material/cellulose to gain access to certain nutrients), then leading to coevolution by vertical transmission through multiple host generations. Coevolving microbes would eventually evolve from facultative to obligate endosymbionts, and horizontal transmission between hosts would be necessary for this transition. These factors would create the necessary selective pressures in hosts to evolve social traits like group living parental care but more research is needed to test these hypotheses. Additionally, the benefits of endosymbionts aren’t just involved in nutrition. One issue with living in close proximity to conspecifics is increasing the spread of pathogens. However, endosymbionts can provide protection against pathogens through possible mechanisms such as outcompeting invaders for spots on epithelial cells, producing toxins (such as amensalism, i.e. producing bacteriocins), or altering host physiology (such as angiogenesis, microbial proteins involved in innate immunity).
Conclusion
Understanding the microbial communities associated with host animals has been difficult for researchers, but technology has allowed them to make new strides.[26] In the 90s, there were limits to what hypotheses could be tested due to the difficulty in culturing symbiotic or animal-associated bacteria. Recent innovations that combat this are known as “culture-independent” survey tools that use DNA and 16S rRNA genes to study diversity and function in bacterial communities by examining genetic variation. In addition, metagenomic data can be used to determine possible functions the bacteria conduct for their hosts. In the future, researchers will need to combine methods of culture-independent surveys with culture-based molecular tools to get the most complete picture possible. Continual use of experimental methods, such as manipulating an animal’s microbiome as seen in “germ-free” tests with antibiotics, will also be key to further understanding the function of these microbial communities. Overall, studying microbes, particularly symbiotic bacteria, is an exciting addition to the field of animal behavior and will be crucial in learning more from the organisms around us and ourselves.
References
- ↑ Dubilier et al.: Symbiotic diversity in marine animals: the art of harnessing chemosynthesis. Nature Reviews Microbiology. 2008 6:725-740.
- ↑ Sharon et al.: Commensal bacteria play a role in mating preference of Drosophila melanogaster. PNAS 2010 107 (46):20051-20056.
- ↑ Heijtz et al.: Normal gut microbiota modulates brain development and behavior. PNAS 2011 108 (7): 3047-3052.
- ↑ McFall-Ngai, M.J.: The importance of microbes in animal development: lessons from the squid-vibrio symbiosis. Annual Review of Microbiology 2014 68:177-194.
- ↑ Stabb E.: Bioluminescent Vibrio fischeri in the light-sensing organ of Euprymna scolopes. Joint Genome Institute 2010.
- ↑ Douglas and Dobson.: New synthesis: animal communication mediated by microbes: fact or fiction?. Journal of Chemical Ecology 2013 39:1149.
- ↑ Theis et al.: Evidence for a bacterial mechanism for group-specific social odors among hyenas. Scientific Reports (Nature) 2012 2:615.
- ↑ Voig et al.: Bats, Bacteria, and Bat Smell: Sex-Specific Diversity of Microbes in a Sexually Selected Scent Organ. Journal of Mammalogy 2005 86(4):745-749.
- ↑ Gorman: A mechanism for individual recognition by odour in Herpestes auropunctatus (Carnivora: Viverridae). Animal Behavior 1976 24(1):141-145.
- ↑ Vuong and Hsiao.: Emerging Roles for the Gut Microbiome in Autism. Biological Psychiatry 2017 81(5):411-423.
- ↑ Desbonnet et al.: Microbiota is essential for social development in the mouse. Molecular Psychiatry 2014 19:146-148.
- ↑ Arentsen et al.: Host microbiota modulates development of social preference in mice. Microb Ecol Health Dis 2015 26:29719.
- ↑ Clarke et al.: The microbiome-gut-brain axis during early life regulates the hippocampal serotonergic system in a sex-dependent manner. Molecular Psychiatry 2013 18:666-673.
- ↑ Buffington et al.: Microbial Reconstitution Reverses Maternal Diet-Induced Social and Synaptic Deficits in Offspring. Cell 2016 165(7):1762-1775.
- ↑ Luo et al.: Ingestion of Lactobacillus strain reduces anxiety and improves cognitive function in the hyperammonemia rat. Science China 2014 Life sciences 57: 327–335.
- ↑ Davis et al.: Lactobacillus plantarum attenuates anxiety-related behavior and protects against stress-induced dysbiosis in adult zebrafish. Scientific Reports (Nature) 2016 6:33726.
- ↑ Bravo et al.: Ingestion of Lactobacillus strain regulates emotional behavior and central GABA receptor expression in a mouse via the vagus nerve. PNAS 2011 108:16050–16055.
- ↑ Lucas et al.: Brood size modifications affect plumage bacterial assemblages of European starlings. Molecular Ecology 2005 14:639–646.
- ↑ Shawkey et al.: Bacteria as an agent for change in structural color: correlational and experimental evidence. The American Naturalist 2007 169:S112-S121.
- ↑ Schifrin.: The pandemic threatens the people of East Africa and now locusts threaten their food. PBS 2020.
- ↑ Obeng‐Ofori et al.: Faecal volatiles as part of the aggregation pheromone complex of the desert locust, Schistocerca gregaria(Forskal) (Orthoptera: acrididae). Journal of Chemical Ecology 1994 20:2077–2087.
- ↑ Dillon et al.: A note: gut bacteria produce components of a locust cohesion pheromone. Society for Applied Microbiology 2002.
- ↑ Moore et al.: Reduction of feeding by the desert locust, Schistocerca gregaria, after infection with Metarhizium flavoviride. Journal of Invertebrate Pathology 1992 60(3):304-307.
- ↑ Lombardo: Access to mutualistic endosymbiotic microbes: an underappreciated benefit of group living. Behavioral Ecology and Sociobiology 2008 62:479-497.
- ↑ Nalepa et al.: Detritivory, coprophagy, and the evolution of digestive mutualisms in Dictyoptera. Insectes Sociaux 2001 48:194–201.
- ↑ Archie and Theis: Animal behavior meets microbial ecology. Animal Behavior 2011 82(3):425-436.
Authored for BIOL 238 Microbiology, taught by Joan Slonczewski, 2018, Kenyon College.