The Human Gut Microbiome: Difference between revisions
Line 50: | Line 50: | ||
<br> | <br> | ||
== | ==Small Intestine== | ||
After being liquified, the stomach contents enter the next phase of the GI tract, the small intestine. Nutritional uptake occurs mainly in the small intestine. The most prominent genera of bacteria are Streptococcus and Veillonella. Cultured isolates of the Streptococcus genus include S. mitis, S. bovis (s equnis), and S. salivaris. These bacteria metabolize sugars that come from food components in order to release immune responses in the gut. S. mitis metabolizes N-acetyl glucosamine and raffinose. S. bovis metabolizes L-Arabinose and raffinose. S. salvarius metabolizes L-Arabinose and Trehalose . A study done by Bogert et al in 2013 analyzed both Streptococcus and Veillonella strains of bacteria found in the human small intestine and concluded that it is possible that Streptococcus populations play a major role in the primary carbohydrate metabolism in the small intestine . Veillonella, on the other hand, ferment the lactic acid that is produced by streptococci to create acetic acid and propionic acid . A 2012 study conducted by Zoetendal et al concluded that the microbiome of the small intestine is highly dependent on available carbohydrates, which supports Bogert et al’s findings that Streptococcus is responsible for carbohydrate metabolism. Zoetendal et al did a comparative functional analysis to determine that simple carbohydrate transport phosphotransferase systems, central metabolism, and biotin production are represented in the small intestine . It is important for these bacteria to have strong metabolism pathways because they directly compete with the host. | |||
An example of a possible symbiotic relationship between the human small intestine and bacteria is that of the B. thetaiotaomicron life cycle. It has been demonstrated in mice that there is fucosylated glycan production in the distal small intestine. B. thetaiotaomicron has evolved to be able to sense the availability of carbohydrates in the surrounding environment and use fucose as an energy source. It picks up fucose, which is a terminal a-linked sugar, by secreting an enzyme called a-frucosidase to obtain the monosaccharide fucose and import it as well as metabolize it. When the bacteria sense a scarcity of fructose, specifically, in the lumen it makes the gut epithelium express fucosylated glycans at a higher rate so that it can use them as an energy source . This signaling pathways is showin in figure 3. When L-fucose (fucose) is present it acts as a corepressor by binding to the control of signal production (csp) and stops the host from producing more fucose. The fucose acts through FucR, which is a repressor that is a molecular senor of fucose availability . This tightly coordinated relationship between the host and the bacteria keep the host from overproducing hydrolysable epithelial fucose. It is possible that this relationship also benefits the health of the small intestine by disallowing the colonization of pathogens that are fucosylated glycan-adhesive. | |||
==References== | ==References== | ||
<references /> | <references /> | ||
<br><br>Authored for BIOL 238 Microbiology, taught by [https://biology.kenyon.edu/slonc/slonc.htm Joan Slonczewski], 2023, [http://www.kenyon.edu/index.xml Kenyon College] | <br><br>Authored for BIOL 238 Microbiology, taught by [https://biology.kenyon.edu/slonc/slonc.htm Joan Slonczewski], 2023, [http://www.kenyon.edu/index.xml Kenyon College] |
Revision as of 23:34, 26 April 2023
Section
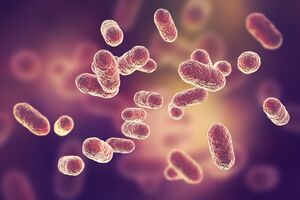
By
At right is a sample image insertion. It works for any image uploaded anywhere to MicrobeWiki.
The insertion code consists of:
Double brackets: [[
Filename: PHIL_1181_lores.jpg
Thumbnail status: |thumb|
Pixel size: |300px|
Placement on page: |right|
Legend/credit: Electron micrograph of the Ebola Zaire virus. This was the first photo ever taken of the virus, on 10/13/1976. By Dr. F.A. Murphy, now at U.C. Davis, then at the CDC. Every image requires a link to the source.
Closed double brackets: ]]
Other examples:
Bold
Italic
Subscript: H2O
Superscript: Fe3+
Evaluation rubric
The page should have minimum 3,000 words and three figures. Number each figure and call out in text, as for example (Figure 1). Some primary research must be cited. Evaluation is based on: writing fluency, including grammar and proofreading; specific details and creative thought; and scientific understanding of microbes.
Sample citations: [1]
[2]
A citation code consists of a hyperlinked reference within "ref" begin and end codes.
To repeat the citation for other statements, the reference needs to have a names: "<ref name=aa>"
The repeated citation works like this, with a forward slash.[1]==Section 1==
Include some current research, with at least one figure showing data.
Every point of information REQUIRES CITATION using the citation tool shown above.
Introduction
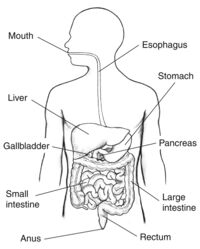
Nutrients from your food are absorbed into your body through complex pathways that occur in the digestive system. Humans need nutrients like proteins, fats, carbohydrates, vitamins, and water in order to survive which are obtained via food digestion. Much of these nutrients are absorbed into the blood through enzymatic pathways. However, some food components are unable to be digested by human enzymes, which is where microbes come into play. Some microbes in the digestive tract, which usually live along the epithelial cells which make up the lining of the digestive tract, are able to digest certain food components that humans are not. Other microbes in the intestines live without a specified benefit to the host and some other microbes can be detrimental to the human host . The digestive system is made up of the gastrointestinal tract-- also known as the GI tract-- liver, pancreas, and gall bladder. This anatomy is shown in figure 1. To reach the nutrients, the body must first break down the food. The first and most simple step to this process is chewing and swallowing food which then begins its pathway through the GI tract.
After being chewed and swallowed the food travel down the esophagus, then to the stomach, then to the small intestine, large intestine, and then to the anus . All the organs that make up the GI tract are hollow to allow the food to pass through. Once the food has passed through the esophagus and into the stomach it is broken down using digestive juices into a liquid form called chyme. Compared to the microbiomes of the small and large intestine, the stomach does not have a large microbiome. This is because the highly acidic environment, as well as other components created by the stomach make the stomach inhabitable for many bacteria. One notable bacterium that lives in the stomach is Helicobacter pylori (H. pylori). H. pylori is in the stomach of most humans but in some cases can cause illnesses such as gastritis or ulcers. It is unique in its ability to use enzymes to neutralize the acidic environment of the stomach.
The chyme produced by the stomach then travels through the small intestine--where most nutrients are absorbed. There are a few genera that are commonly found in the small intestine including Streptococcus and Veillonella. They are known for breaking down and metabolizing carbohydrates. They compete directly with the host for food components. These bacteria do not have a direct beneficial effect on the host, but it is possible that one bacterium, called Bacteroides thetaiotaomicron (B. thetaiotaomicron), has the ability to keep the small intestine from producing too much fucose. The microbiome of the large intesting has the largest plethora of bacteria. Once the chyme has passed into the large intestine, it is depleted of most of the nutrients and the microbes then digest what the host cannot. The large intestine introduces the chyme to a plethora of colonic bacteria. These bacteria are able to digest certain carbohydrates, particularly those from plant derived foods, that the small intestine was not able to. Vitamin B production is also a critical function of the large intestine because it sources the body with the vitamin B that is not ingested from food sources. Vitamin B also is used to produce the energy used by the large intestine. Other functions of the large intestine include, but are not limited to, water absorption via osmosis and the passing of waste to the anus.
Stomach Microbiota
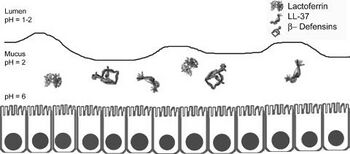
The main purpose of the stomach is to further break down the chewed food. The stomach is able to do this by producing digestive juices made up of stomach acid and enzymes from glands in the stomach lining. Many studies have analyzed the digestive juices for their microbiota. A study done by Bik et al in 2005 analyzed the stomach microbiota for the first time using a small subunit 16S rDNA clone library approach and found five dominant genera including Streptococcus, Prevotella, Rothia, Fusobacterium and Veillonella. Other studies conducted in later years using the same method found other genera including Neisseriae, Haemophilus, Porphyromonas, Rothia, Propionibacterium, and Lactobacillus.
However, many of these bacteria may be transient in the stomach since the stomach is open to a swelling of bacteria from the oral cavity. For example, Lactobacilli is known to be present in the mouth for the purpose of converting the nitrate found in food and saliva into nitrite which can be converted into nitric oxide by gastric juice in the stomach to produce a useful antimicrobial agent . Most microbes in general do not survive in the stomach due to its high acidity. On top of its high acidity, the stomach utilizes its gastric mucosa to produce other factors to kill bacteria that are transported from the oral cavity including peptides B-defensins 1 and 2 and LL-37 as shown in figure 2. These peptides fight off many species of bacteria. Also, an enzyme called lysozyme degrades bacterial peptidoglycan. Proteins in the stomach can also inhibit bacterial growth including lactoferrin and surfactant protein D. Lactoferrin inhibits bacterial colonization by reducing extracellular Fe3+ and surfactant protein D brings the bacteria into close proximity to each other. Finally, other mucosal components such as alpha-1,4-GluNAC-capped O-glycans, which disrupt the biosynthesis of cholesteryl-α-D-glucopyranoside, a component of the H. pylori cell wall . Many bacteria that are specialized to live within the human stomach are still unknown, but among the 128 identified phylotypes identified by Bik et al in 2005, one particularly interesting sequence identified was that belonging to Deinococcus/ Thermus phylum. This phylum is known to live in extreme environments such as hot springs and radioactive sites.
Although much is still unknown about the stomach microbiota, one famous bacterium in the stomach is H. pylori. About 50% of the human population is known to be infected with this bacterium, but most have no symptoms. H. pylori is associated with an increased risk of duodenal ulcer disease, gastric ulcer disease, gastric adenocarcinoma, and gastric lymphoma. H. pylori is a gram-negative bacterium that elicits humoral and cellular immune responses. H. pylori is the only known bacteria to colonize in the gastric mucosa and crosstalk with the host. It does not partake in nutrient absorption but instead is the known cause of gastritis. It can survive the highly acidic environment by using its urease enzyme to produce ammonia and neutralize the acid . Strains of H. pylori that can cause infection have flagella that mobilize it so that it can pass from the lumen, which has a pH between 1-2 to the mucus layer that lines the epithelial cells and has a pH of 2. It is then able to travel through the mucus layer to its ideal environment on the epithelial cell surface which has a pH of 6 . The bacteria is able to attach on to the cell surface via outer membranes proteins that include BabA, SabA, AlpA, AlpB, and HopZ. This adherence allows for the activation of signaling pathways that allow for toxins to enter the cells which incurs gastric mucosal inflammation, production of autoantibodies, and parietal cell loss.
Small Intestine
After being liquified, the stomach contents enter the next phase of the GI tract, the small intestine. Nutritional uptake occurs mainly in the small intestine. The most prominent genera of bacteria are Streptococcus and Veillonella. Cultured isolates of the Streptococcus genus include S. mitis, S. bovis (s equnis), and S. salivaris. These bacteria metabolize sugars that come from food components in order to release immune responses in the gut. S. mitis metabolizes N-acetyl glucosamine and raffinose. S. bovis metabolizes L-Arabinose and raffinose. S. salvarius metabolizes L-Arabinose and Trehalose . A study done by Bogert et al in 2013 analyzed both Streptococcus and Veillonella strains of bacteria found in the human small intestine and concluded that it is possible that Streptococcus populations play a major role in the primary carbohydrate metabolism in the small intestine . Veillonella, on the other hand, ferment the lactic acid that is produced by streptococci to create acetic acid and propionic acid . A 2012 study conducted by Zoetendal et al concluded that the microbiome of the small intestine is highly dependent on available carbohydrates, which supports Bogert et al’s findings that Streptococcus is responsible for carbohydrate metabolism. Zoetendal et al did a comparative functional analysis to determine that simple carbohydrate transport phosphotransferase systems, central metabolism, and biotin production are represented in the small intestine . It is important for these bacteria to have strong metabolism pathways because they directly compete with the host.
An example of a possible symbiotic relationship between the human small intestine and bacteria is that of the B. thetaiotaomicron life cycle. It has been demonstrated in mice that there is fucosylated glycan production in the distal small intestine. B. thetaiotaomicron has evolved to be able to sense the availability of carbohydrates in the surrounding environment and use fucose as an energy source. It picks up fucose, which is a terminal a-linked sugar, by secreting an enzyme called a-frucosidase to obtain the monosaccharide fucose and import it as well as metabolize it. When the bacteria sense a scarcity of fructose, specifically, in the lumen it makes the gut epithelium express fucosylated glycans at a higher rate so that it can use them as an energy source . This signaling pathways is showin in figure 3. When L-fucose (fucose) is present it acts as a corepressor by binding to the control of signal production (csp) and stops the host from producing more fucose. The fucose acts through FucR, which is a repressor that is a molecular senor of fucose availability . This tightly coordinated relationship between the host and the bacteria keep the host from overproducing hydrolysable epithelial fucose. It is possible that this relationship also benefits the health of the small intestine by disallowing the colonization of pathogens that are fucosylated glycan-adhesive.
References
Authored for BIOL 238 Microbiology, taught by Joan Slonczewski, 2023, Kenyon College