Bioluminescence in Pyrocystis fusiformis and Vibrio harveyi: Difference between revisions
Line 1: | Line 1: | ||
==Introduction== | ==Introduction== | ||
[[Image:Dinos.jpg|thumb|400px|right|<i>Pyrocystis fusiformis</i> [http://www.lifesci.ucsb.edu/%7Ebiolum/organism/pictures/dinos.html picture 1] picture taken by Martin Dohm]] | [[Image:Dinos.jpg|thumb|400px|right|<i>Pyrocystis fusiformis</i> [http://www.lifesci.ucsb.edu/%7Ebiolum/organism/pictures/dinos.html picture 1] picture taken by Martin Dohm]] | ||
<br> <i>Pyrocystis fusiformis</i> is a marine dinoflagellate. Dinoflagellates are marine unicellular planktonic organisms. A few species are found in freshwater environments, however 90% of dinoflagellate species are marine. These organisms are found throughout the world’s oceans, concentrating at the top euphotic zone of the ocean’s water column [http://www.jstor.org/sici?sici=0006-3185%281982%29162%3A3%3C423%3ADOSBSI%3E2.0.CO%3B2-Q 7.].Dinoflagellates can perform photosynthetic metabolism, heterotrophic metabolism, or both. <i>P. fusiformis</i> is a mixotroph, meaning that it conducts both photosynthetic and heterotrophic metabolism [http://biology.kenyon.edu/slonc/Micro/D489Mbio.pdf 5]. As photosynthesizing organisms, dinoflagellates produce a substantial amount of the world’s oxygen, and consume a large proportion of the atmosphere’s carbon dioxide. Dinoflagellates can be found in large numbers in the ocean, and as a result consume a considerable amount of carbon dioxide. Their consumption of carbon dioxide creates a major carbon sink in the carbon cycle [http://biology.kenyon.edu/slonc/Micro/D489Mbio.pdf 5]. This carbon sink is crucial for the function of the global carbon cycle. Dinoflagellates are also important in marine food webs and ecosystems. Dinoflagellates consume other planktonic species, as well as provide a food source for marine filter-feeding organisms such as fish, whale sharks, and baleen whales. Dinoflagellates contribute to the producer trophic level of the marine food web, and help to maintain the diversity of marine organisms seen in the marine ecosystem. Some photosynthetic dinoflagellate species live as endosymbionts in marine invertebrates such as sponges and corals [http://www.ucmp.berkeley.edu/protista/dinoflagellata.html 8.]. | <br> <i>Pyrocystis fusiformis</i> is a marine dinoflagellate. Dinoflagellates are marine unicellular planktonic organisms. A few species are found in freshwater environments, however 90% of dinoflagellate species are marine. These organisms are found throughout the world’s oceans, concentrating at the top euphotic zone of the ocean’s water column [http://www.jstor.org/sici?sici=0006-3185%281982%29162%3A3%3C423%3ADOSBSI%3E2.0.CO%3B2-Q 7.].Dinoflagellates can perform photosynthetic metabolism, heterotrophic metabolism, or both. <i>P. fusiformis</i> is a mixotroph, meaning that it conducts both photosynthetic and heterotrophic metabolism [http://biology.kenyon.edu/slonc/Micro/D489Mbio.pdf 5]. As photosynthesizing organisms, dinoflagellates produce a substantial amount of the world’s oxygen, and consume a large proportion of the atmosphere’s carbon dioxide. Dinoflagellates can be found in large numbers in the ocean, and as a result consume a considerable amount of carbon dioxide. Their consumption of carbon dioxide creates a major carbon sink in the carbon cycle [http://biology.kenyon.edu/slonc/Micro/D489Mbio.pdf 5]. This carbon sink is crucial for the function of the global carbon cycle. Dinoflagellates are also important in marine food webs and ecosystems. Dinoflagellates consume other planktonic species, as well as provide a food source for marine filter-feeding organisms such as fish, whale sharks, and baleen whales. Dinoflagellates contribute to the producer trophic level of the marine food web, and help to maintain the diversity of marine organisms seen in the marine ecosystem by providing an essential food source. Some photosynthetic dinoflagellate species live as endosymbionts in marine invertebrates such as sponges and corals [http://www.ucmp.berkeley.edu/protista/dinoflagellata.html 8.]. | ||
<br>''P. fusiformis'' produces bioluminescence on a circadian rhythm, meaning that it photosynthesizes during the day and produces bioluminescence when mechanically or chemically stimulated at night [http://www.jstor.org/sici?sici=0006-3185%281982%29162%3A3%3C423%3ADOSBSI%3E2.0.CO%3B2-Q 7.] <i>P. fusiformis'</i> bioluminescence, or emitted blue-green light, originates from microsources found evenly distributed throughout the cytoplasmic layer surrounding the large central vacuole. A single <i>P. fusiformis</i> cell contains an average of 4,500 microsources. During the day, the microsources migrate from the cell’s periphery to a spherical region distal to the nucleus. During their migration from the periphery, they are replaced by chloroplasts. This results in a lack of daytime bioluminescence. The microsources return to the periphery at night, and produce bioluminescence [http://www.jstor.org/sici?sici=0006-3185%281982%29162%3A3%3C423%3ADOSBSI%3E2.0.CO%3B2-Q 7.] Microsources are composed of a round mass of vesicles which contain electron-dense short rods with rounded ends, sometimes crossed by electron-transparent narrow bands. Their high electron density allows the microsources to produce bioluminescence [http://www3.interscience.wiley.com/journal/119558442/abstract?CRETRY=1&SRETRY=0 6.]. <br> | <br>''P. fusiformis'' produces bioluminescence on a circadian rhythm, meaning that it photosynthesizes during the day and produces bioluminescence when mechanically or chemically stimulated at night [http://www.jstor.org/sici?sici=0006-3185%281982%29162%3A3%3C423%3ADOSBSI%3E2.0.CO%3B2-Q 7.] <i>P. fusiformis'</i> bioluminescence, or emitted blue-green light, originates from microsources found evenly distributed throughout the cytoplasmic layer surrounding the large central vacuole. A single <i>P. fusiformis</i> cell contains an average of 4,500 microsources. During the day, the microsources migrate from the cell’s periphery to a spherical region distal to the nucleus. During their migration from the periphery, they are replaced by chloroplasts. This results in a lack of daytime bioluminescence. The microsources return to the periphery at night, and produce bioluminescence [http://www.jstor.org/sici?sici=0006-3185%281982%29162%3A3%3C423%3ADOSBSI%3E2.0.CO%3B2-Q 7.] Microsources are composed of a round mass of vesicles which contain electron-dense short rods with rounded ends, sometimes crossed by electron-transparent narrow bands. Their high electron density allows the microsources to produce bioluminescence [http://www3.interscience.wiley.com/journal/119558442/abstract?CRETRY=1&SRETRY=0 6.]. <br> |
Revision as of 18:52, 1 May 2009
Introduction
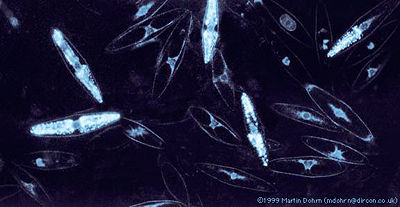
Pyrocystis fusiformis is a marine dinoflagellate. Dinoflagellates are marine unicellular planktonic organisms. A few species are found in freshwater environments, however 90% of dinoflagellate species are marine. These organisms are found throughout the world’s oceans, concentrating at the top euphotic zone of the ocean’s water column 7..Dinoflagellates can perform photosynthetic metabolism, heterotrophic metabolism, or both. P. fusiformis is a mixotroph, meaning that it conducts both photosynthetic and heterotrophic metabolism 5. As photosynthesizing organisms, dinoflagellates produce a substantial amount of the world’s oxygen, and consume a large proportion of the atmosphere’s carbon dioxide. Dinoflagellates can be found in large numbers in the ocean, and as a result consume a considerable amount of carbon dioxide. Their consumption of carbon dioxide creates a major carbon sink in the carbon cycle 5. This carbon sink is crucial for the function of the global carbon cycle. Dinoflagellates are also important in marine food webs and ecosystems. Dinoflagellates consume other planktonic species, as well as provide a food source for marine filter-feeding organisms such as fish, whale sharks, and baleen whales. Dinoflagellates contribute to the producer trophic level of the marine food web, and help to maintain the diversity of marine organisms seen in the marine ecosystem by providing an essential food source. Some photosynthetic dinoflagellate species live as endosymbionts in marine invertebrates such as sponges and corals 8..
P. fusiformis produces bioluminescence on a circadian rhythm, meaning that it photosynthesizes during the day and produces bioluminescence when mechanically or chemically stimulated at night 7. P. fusiformis' bioluminescence, or emitted blue-green light, originates from microsources found evenly distributed throughout the cytoplasmic layer surrounding the large central vacuole. A single P. fusiformis cell contains an average of 4,500 microsources. During the day, the microsources migrate from the cell’s periphery to a spherical region distal to the nucleus. During their migration from the periphery, they are replaced by chloroplasts. This results in a lack of daytime bioluminescence. The microsources return to the periphery at night, and produce bioluminescence 7. Microsources are composed of a round mass of vesicles which contain electron-dense short rods with rounded ends, sometimes crossed by electron-transparent narrow bands. Their high electron density allows the microsources to produce bioluminescence 6..
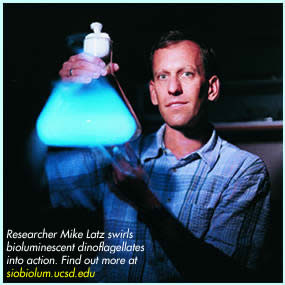
Why does Pyrocystis fusiformis Bioluminesce?
According to the ‘bulgar alarm theory’, Pyrocystis fusiformis bioluminesces in order to attract attention to its predator. When attacked by a predator, P. fusiformis bioluminesces and illuminates itself as well as its predator. When its predator is illuminated, it greatly increases the chance that the predator itself will be preyed upon. This benefits the P. fusiformis species as a whole, because the dinoflagellates tend to exist in groups. The chance that P. fusiformis’ predator will be seen and eaten as a result of bioluminescence is greater than the chance that the entire P. fusiformis colony will be preyed upon, which favors the survival of the P. fusiformis group as well as the luminescent genotype.
One study by Fleisher and Case (1995) 1 supports the ‘buglar alarm theory’. The study shows that two species of squid (Sepia officinalis and Euprymna scolopes) use P. fusiformis bioluminescence to locate and capture nonluminous prey. Holmesimysis sculpta (mysids), Palaemonetes pugio (grass shrimp), and Gambusia affinis (mosquito fish) prey upon P. fusiformis, and were used as squid prey in the study. The squid prey, P. fusiformis, and the squid were placed in dark tanks. The amount of squid prey consumed was measured with and without the presence of P. fusiformis. The interaction was viewed using infrared video cameras. The predation of the three types of squid prey on P. fusiformis caused P. fusiformis to bioluminesce, allowing for easier prey capture by the squid because their prey was illuminated. Mosquito fish were observed to trigger luminescence with each tail stroke, which the squid monitored closely. Grass shrimp appendages also triggered luminescence, and the attention of the squid predators. The average number of mysids, grass shrimp, and mosquito fish consumed by the two species of squid significantly increased with increasing P. fusiformis concentration in the experimental tank.
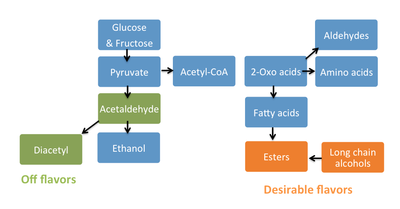
Figure 1 shows that the presence of P. fusiformis in the experimental tanks significantly increased the number of mysid individuals that were consumed by the squid. Higher concentrations of P. fusiformis in the tanks resulted in significantly more mysid individuals consumed because higher concentrations of P. fusiformis led to increased illumination of the mysids. Thus, predation of squid on nonluminescent prey in the dark is positively correlated with the presence of bioluminescent P. fusiformis and supports the ‘buglar alarm theory’.
The presence of P. fusiformis also allowed the squid to capture nonluminescent prey at a quicker rate. In the study, 11 squid in the presence of luminescent P. fusiformis took less than 10 minutes to capture prey, and all prey was consumed within 20 minutes. In the absence of P. fusiformis only one nonluminescent prey individual was consumed, and this attack occurred after 30 minutes. Thus, the presence of luminescent P. fusiformis greatly increased predation on nonluminescent squid prey. This study shows that cephalopods are able to use the light of dinoflagellates such as P. fusiformis to locate their nonluminescent prey 1.
The "Milky Sea" Phenomenon
On many occasions over the centuries, mariners have testified to witnessing unusual nocturnal ocean displays where the surface of the ocean produces an intense glow that seems to extend in all directions for many miles. This phenomenon has been labeled the ‘milky seas’ phenomenon, and little is known about its formation mechanisms, spatial extent, global distribution, and ecological implications. The sparse information that is known about this effect is derived almost entirely from archived ship logs, and this information is subject to error resulting from human perception and interpretation. The information known results from 235 documented cases reported since 1915. Based on this information, milky seas are known to occur only at night, glow continuously over an extensive area, are independent of wind speeds, last anywhere from several hours to several days, and may be associated with oceanographic fronts or biological slicks. More than 70% (171 cases) of documented milky seas were observed in the northwest Indian Ocean, most commonly during the summer southwest monsoon. These observation statistics seem to be biased towards active shipping routes, however reports of milky seas from other heavily used shipping routes are exceedingly infrequent 3.
The ‘milky seas’ phenomenon was initially hypothesized to be a result of large aggregations of Pyrocystis fusiformis individuals producing bioluminescence. This hypothesis has been discounted, however, due to the fact that P. fusiformis emits brief, bright flashes in response to mechanical disturbance. It seems unlikely that brief flashes, even if repeated, could produce the ‘milky sea’ effect. It also seems unlikely that a sustained uniform mechanical stimulation exists that would allow P. fusiformis to cause the ‘milky sea’ phenomenon. A more likely hypothesis explaining the ‘milky sea’ phenomenon states that luminous bacteria such as Vibrio harveyi could be the source of emitted light because they can emit a continuous glow that can persist for days under specific conditions. This hypothesis suggests that the ‘milky sea’ effect results from bioluminescent bacteria living in microalgal blooms in the surface waters of the ocean. A study by Miller et al. (2005) supports the second hypothesis, and details the first satellite observations of the ‘milky seas’ phenomenon.
In the study, a 15,400-km squared area of the northwestern Indian Ocean, roughly the size of Connecticut, was observed to glow over 3 consecutive nights. Measurements from the U.S. Defense Meteorological Satellite Program constellation of satellites were used that detected low-light emissions picked up from the area where the milky sea was observed by a passing British merchant ship, the SS Lima on January 25th, 1995. The ship’s log states,
“25, January 1995. At 1800 [GMT] (2100 local time) on a clear moonless night while 150 nautical miles east of the Somalian coast, a whitish glow was observed on the horizon, and after 15 minutes of steaming, the ship was completely surrounded by a sea of milky-white color with fairly uniform luminescence. The bioluminescence appeared to cover the entire sea area, from horizon to horizon…and it appeared as though the ship was sailing over a field of snow or gliding over the clouds…thick patches of kelp appeared black against the white water. 3”
Unprocessed OLS nighttime visible satellite imagery from approximately one-half hour into the Lima’s encounter with the milky sea indicates the presence of a large, bright feature near the Lima’s location, which was observed to persist over the next two nights. Positions of the Lima reported over the course of its encounter coincide closely with the boundaries of the satellite-observed bright feature. This occurrence constitutes the first time a milky sea has been recorded by both human visual and satellite observation. Miller et al. explain that this milky sea most likely resulted from Vibrio harvei living in association with a microalga Phaeocystis bloom, as the Lima observed patches of “kelp” in the milky sea. The area where the milky sea was observed is known to be a preferred habitat for phytoplankton colonies and an area where algae blooms often occur. The researchers also discovered the presence of a cold-core eddy where the milky sea was observed, which most likely contributed to maintaining the conditions necessary to keep the algae bloom in place and allowed the milky sea to occur 3.
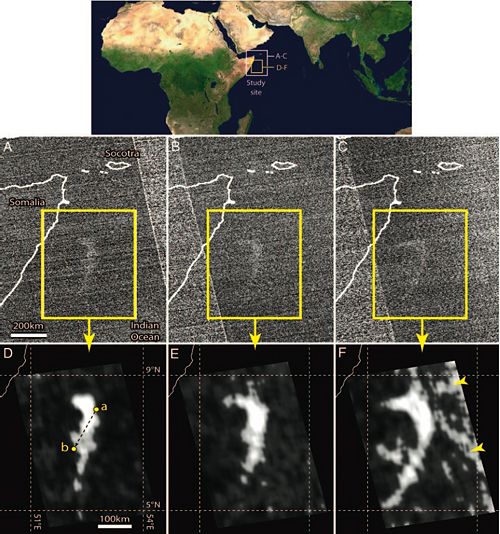
The researchers explain that the emitted light of the milky sea was produced by high concentrations of Vibrio harveyi participating in quorum sensing. V. harveyi only bioluminesce in high cell density, and use quorum sensing to determine if there is a high enough concentration of other individuals of its species in order to do so. The lux system mediates V. harveyi’s bioluminescence. In this system, the LuxI protein synthesizes an acyl homoserine lactone autoinducer (AI). AI diffuses into the surrounding enviroment, where it accumulates. When it reaches a high enough concentration, AI diffuses back into the V. harveyi cell and binds to the activator protein LuxR, resulting in increased transcription of the lux operon. The lux operon activates transcription of the luciferase target genes that allow V. harveyi to bioluminesce 5. In this system, the more V. harveyi individuals that are producing AI, the more the individuals will bioluminesce. Figure 2 displays the satellite image of the milky sea event.
V. harveyi individuals are able to quorum sense and bioluminesce during a milky sea event because they aggregate with algal blooms. The microalga bloom seen in the milky sea event in 1995 allowed the bacteria to attach to a solid substrate, which aided the bacteria’s quorum sensing because it kept the bacteria close together and allowed them to sense the AI concentration. Miller et al. (2005) hypothesize that algal blooms may be a necessary component of milky sea events because they allow the bacteria to maintain high enough levels in order to bioluminesce. Luminous bacteria associate with algal species in the ocean in order to gain nutrients from material produced by the algae. The bacteria grow on the algae in colonies, producing and accumulating AI and ultimately emitting light. When algal blooms occur, the bacteria also bloom due to the increase in nutrients. Milky seas occur as large algal blooms begin to break down and as massive amounts of decomposing lipids and hydrocarbon-rich microbial material accumulate as a surface film, where the growth of a luminous bacterial species is favored. Confined to the film, where nutrients from the decomposing algae accumulate, AI accumulates and luminescene is induced 4.
Milky seas remain a lesser studied phenomenon. The use of satellite imaging will hopefully allow researchers to discover more about milky sea formation mechanisms, global distributions, and ecological implications.
Industrial Use for Bioluminescence in Pyrocystis fusiformis
Pyrocystis fusiformis is considered a useful organism industrially because of its use in bioassays. Phytoplankton bioassays are currently used as biological tools to determine the extent of contamination in a given area. Phytoplankton are useful test organisms in bioassays because they are simple and inexpensive organisms in comparison to fish and vertebrate species. In 1989, a bioluminescent dinoflagellate bioassay termed “QwikLite” was developed using P. fusiformis. The bioassay measured the light output from bioluminescent dinoflagellates to assess acute and sublethal toxic effects. QwikLite specifically aided in the testing for toxicity at naval facilities. Most naval facilities are located within harbors and bays and therefore have direct impacts on marine flora and fauna, particularly plankton. The development of the dinoflagellate bioassay allowed for the identification of toxins in the area near the naval base in both the water and sediment within a few days, while other bioassay tests need several weeks to determine the outcome of the tests.
QwikLite was compared with other conventional toxicity tests using minnows and shrimp to assess storm water outfalls and industrial wastewater treatment plant effluent collected at the U.S. Naval Shipyard, Norfolk Virginia 2. Water samples were collected from various storm water outfalls as well as dry dock outfalls during storm events from September through June 1996. P. fusiformis was placed in solution containing a portion of the collected water samples, and put in a Laboratory Plankton Test Chamber (LPTC). The LPTC measured light output from P. fusiformis, and its readings allowed researchers to determine the toxicity of the sample. P. fusiformis lost its ability to bioluminesce following exposure to heavy metals, organic compounds such as ammonia and polycyclic aromatic hydrocarbons, and sediments bound with metals and organics. The QuikLite bioassay proved to be an effective and sensitive indicator of toxicity in contaminated waters and sediments.
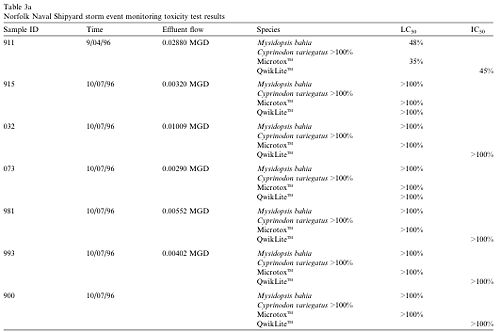
Table 1 shows the concentration of test material that caused a 50% reduction or inhibition of bioluminescence in the QwikLite bioassay (IC50), and a 50% reduction of test individuals in the other bioassays used (LC50). The first sample in the table displayed toxicity, while the other samples did not. The first sample caused a 50% reduction in bioluminescence when P. fusiformis was treated with 45% of the test material, and caused a 50% reduction in shrimp with 48% of the test material. The other samples did not contain toxins because it took more than the entire sample to reach a 50% reduction in bioluminescence as well as the other bioassay test organisms 2. The data indicates that QuikLite can be used as an bioassay and yield accurate results. Pyrocystis fusiformis can be used in bioassays to provide a fast and sensitive assessment of present environmental conditions.
Conclusion
Pyrocystis fusiformis is an interesting marine dinoflagellate that produces bioluminescence. It was thought to be the cause of the “milky sea” phenomenon, but this theory has been discredited. Pyrocystis fusiformis has proven to be an excellent organism to use in bioassay toxicity tests.
References
Written by student Kim Miller Edited by student of Joan Slonczewski for BIOL 238 Microbiology, 2009, Kenyon College.