Evolution of HIV
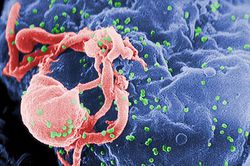
Human immunodeficiency virus/acquired immune deficiency syndrome (HIV/AIDS) is a global problem of a staggering magnitude that not only impacts human health but economic and social development. In 2013, it was estimated that 35 million people were living with HIV, with over 2 million new infections [1]. It is transmitted in many ways, such as unprotected sex, infected blood donations, intravenous drug use, and from mother to child. HIV/AIDS destroys the immune system by infecting CD4+ T cells and leaves the patient unable to fight off other opportunistic diseases. In order to understand the lethality of HIV and how it evades current treatment options despite continued efforts to fight this disease, it is critical to examine the molecular components of this virus and its history.
Early Beginnings
What most people consider HIV today is actually comprised of several strains. HIV-1 is the predominant strain that has spread worldwide, while HIV-2 is still limited to parts of Africa. Furthermore, HIV-1 is divided into several subtypes, of which type M is the most predominant globally, with subtypes N, O, and P being much rarer. As well, HIV-1 type M is further subdivided into several subclades.
HIV is descended from a virus that infects African primates, called simian immunodeficiency virus (SIV), and which jumped from primates to humans many times to create the different strains [2]. However, most of the time, SIV is too weak to cause AIDS in humans. It requires mutations and adaptations to the human host, and it has been suggested that it be transmitted between multiple human hosts in a certain time frame for SIV to accumulate useful mutations that would change it into HIV. This can be seen in subtypes of HIV that are not globally relevant. They are much weaker pathogens, replicate and are passed on poorly, and are usually locally constrained [3]. This indicates that the main subtypes of HIV that are globally important have accumulated many mutations and were shuttled around quickly to cause the global health crisis today.
Using evolutionary analysis, HIV-1 subtype M has been dated to around 1920. This was done with early sequence data of HIV viruses, as well as collection of samples from blood and tissue that was used to create phylogenetic trees. As well, most of the data has suggested that it started in the Democratic Republic of the Congo, in an area now known as Kinshasa. Both a high density of samples and a high diversity of sequence from samples in Kinshasa have supported this. From there, HIV migrated outwards to surrounding areas, helped by transport links such as river traffic and railway connections [4].
Around 1960, the evolution and growth of HIV exploded and transmission increased exponentially. This can be attributed to the establishment of reservoirs of infection in sex workers, as well as the use of unsterilized needles for injections in medical clinics. The disease was then spread to Haiti, where it made its way to the United States. This is all in contrast to subtype O, which never made it out of the region. This indicates that the right convergence of spatial, temporal, and societal variables all lined up to give HIV the boost it needed to become a pandemic[4].
In the United States, 1981, doctors first noticed the emergence of two rare diseases that tended to only afflict the elderly and the immunocompromised, Pneumocystis pneumonia and Karposi’s sarcoma. What was startling about this outbreak was the fact that these disease were found in young and otherwise healthy adults. These opportunistic infections in seemingly healthy adults alerted the CDC and the American public to a previously unknown disease with an unknown means of transmission. Public anxiety grew, and it became clear that multiple communities were at risk from this new disease. The virus was discovered in 1983, and more and more was discovered about the etiological agent and the disease it caused[5].
Reverse Transcriptase and Mutation
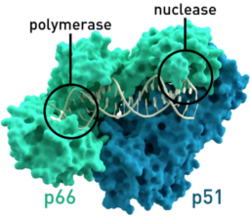
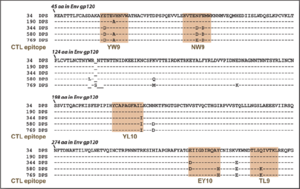
HIV relies on multiple proteins to replicate and propagate itself. One of these is reverse transcriptase, which transcribes RNA from the virus into DNA to be integrated into the genome of the host. This is a critical function for the replication of the virus, because HIV does not have the necessary translational and replication machinery, relying on the host to transcribe HIV’s genetic information along with the host information to create the proteins it needs to form progeny.
It is known that HIV’s reverse transcriptase is a “sloppy” enzyme. In the transcription from RNA to DNA, reverse transcriptase makes many mistakes, which leads to a high mutation rate. These mutations allow HIV to change rapidly and combat any host defenses that may arise, such as drugs against proteins or cytotoxic T lymphocytes produced by the immune system. As well, the mutation of the virus allows for fast evolution and the adaptation to the host, which may lead to higher virulence and pathogenicity. In a paper by Preston et al., published in Science, the group showed that on average, the HIV genome encodes roughly 5-10 mutations per round of replication[7]. Relative to the size of its genome, this is a very high rate of mutation compared to many organisms, leading to an accelerated timeframe for HIV mutation[7]. While some mutations are deleterious and lead to decreased fitness, some increase fitness of viral progeny and propagate the disease.
Drugs Therapy and Resistance
With the introduction of antiretroviral therapy, the prognosis for an HIV infection became markedly better. However, challenges remain in drug treatment, which can cost over $10,000 a year in the United States, representing up to 84% of HIV/AIDS treatment costs[16].
Highly active antiretroviral therapy (HAART) works by targeting the virus at multiple points in its life cycle. HIV needs to attach to CD4 and CCR5 or CRCX4 (link to Wikipedia) on the cell surface, which is blocked by entry inhibitors. HIV then needs to fuse with the cell membrane to release its RNA into the cell, which is blocked by fusion inhibitors. HIV then reverse transcribes its RNA into DNA, which is blocked by reverse transcriptase inhibitors. Nucleoside reverse transcription inhibitors work by acting as a nucleobase analog that can be incorporated into the growing chain, but block extension. Non-nucleoside reverse transcription inhibitors work by binding to the reverse transcriptase enzyme and inhibiting its function. The reverse transcribed DNA is then integrated into the genome, which can be blocked by integrase inhibitors. Once the DNA is integrated, the host transcribes and translates the DNA into RNA and protein. HIV’s proteins are unique in that they are translated as a long peptide chain, which is then cleaved by proteases to form mature proteins. This is blocked by protease inhibitors. Once the necessary proteins and RNA made, the new virus is packaged and buds from the cell to infect a fresh host[8].
In order to combat resistance to the drugs, because of its high mutation rate, HAART is comprised of a multi-drug cocktail. By administering multiple drugs, usually three, targeting multiple points in its life cycle, it minimizes the chances that HIV can develop resistance to all the drugs at once and create an escape mutant. However, if the levels of drug present in the body are not enough to completely suppress and block replication, but enough to exert a selection pressure on the viral population, then resistance can quickly develop. As well, if the drug regimen is not followed strictly every day, and is instead sporadic, then this offers opportunity for the virus to adapt and mutate.These resistant strains can be passed among people, much like antibiotic resistance bacteria[9].
For instance, nucleoside and nucleotide reverse transcriptase inhibitor analogues can be blocked by HIV through two types of mutations. The attached nucleotide or nucleoside can be excised through an ATP or pyrophosphate attack on the phosphodiester bond of the chain, known as thymidine analogue mutations. As well, the active site of the reverse transcriptase enzyme can be mutated to no longer fit the analogue bases, changing methionine 184 to valine. Another class of drug, the non-nucleoside reverse transcriptase inhibitor, works by binding to the active site of the enzyme. However, mutations can quickly accumulate which change the conformation and hydrophobicity of the binding pocket. Occasionally a single point mutation can markedly change the geometry and properties of the active site. This leads to a decreased affinity for the drug and a parallel increase in resistance to the compound[9]. In these cases, if viral load increases despite HAART, then the drug regimen must be quickly changed to suppress further mutation which may result in increased pathogenicity.
Epidemiology and the Future
A person’s response to the infection is primarily dictated by his or her human leukocyte antigen (HLA) locus and the major histocompatibility complex that this locus encodes for. A study by Payne et al. looks into the response that the virus takes when presented with an HLA type that is highly protective because escape mutants have modulated their behavior to adapt. The study compared populations that have been exposed to the virus for different periods of time: Botswana, where the virus has been established for a longer period of time, and South Africa. It suggests that virulence decreases with time as the virus becomes adapted to the human population and the various HLA subtypes, as there is less of a protective effect from previously protective HLA subtypes in Botswana, while the same HLA subtype in South Africa conferred more protection. The authors hypothesize that this is happening with antiretroviral therapy as well[11].
A competing study, published by The Lancet HIV, examines a specific population in Europe, and reached a different conclusion. They found that virulence has actually increased, mainly based by the faster drop in CD4+ counts in HIV infected patients, the main way to diagnose the induction of AIDS[12]. However, the contradicting findings may be because of two different populations with two different genetic backgrounds and two different contexts that may change the response of the virus. It is important to note that there are many different responses to the disease and that findings may not be generalizable, due to many variables that can affect the expression and response to the virus.
Promising new therapies for HIV/AIDS includes genomic editing through the CRISPR-Cas9 system, which can specifically target specific loci in a genome and perform exquisitely precise editing by deletion, insertion, or a simple change of information. The Δ32 mutation in CCR5, which is found in some European populations, changes the conformation of CCR5, a critical coreceptor needed for HIV entry into the cell. These people present as phenotypically normal, yet HIV cannot enter into their cells, so they are effectively immune to HIV infection. A striking example is the "Berlin patient." He was effectively cured of HIV when his treatment for leukemia was to ablate his immune system and receive a bone marrow transplant from a matched donor with this specific mutation[14]. This reconstituted his immune system with cells that were resistant to HIV infection, and to this day he has remained virus free. Therefore, if CRISPR-Cas9 can create this mutation in stem cells, creating a population of infection resistant progenitor cells and lymphocytes, this could render a person immune to infection.
Another finding that was published in Nature in 2015 was the announcement that a pseudo-vaccine had been created. When injected into muscle cells, the vector inserted DNA into the genome that, when expressed, would code for an engineered antibody-like protein that attached to the receptors needed by HIV to enter the cell, CD4 and CCR5. These would prevent HIV from entering the cell[15].
References
1. Global Statistics (2014). AIDS.gov.
2. Zimmer, Carl. (2015). “Two Strains of HIV Cut Vastly Different Paths.” New York Times.
5. “History of AIDS Up to 1986."(2014). Avert.org.
8. “The HIV Life Cycle.” (2013). AIDSinfo. National Institutes of Health.
9. Clavel, F. et al. (2004). “HIV drug resistance.” The New England Journal of Medicine 350: 1023-1035.
14. Ledford, Heidi (2009). "Stem-cell transplant wipes out HIV." Nature News.
Edited by Sam Du, a student of Suzanne Kern in BIOL168L (Microbiology) in The Keck Science Department of the Claremont Colleges Spring 2015.