Mycena chlorophos
1. Classification
a. Higher order taxa
Domain: Eukaryote; Phylum: Basidiomycota; Class: Agaricomycetes; Order: Agaricales; Family: Mycenaceae; Genus: Mycena (Taxonomy).
b. Species
Mycena chlorophos
2. Introduction
3. Genome structure
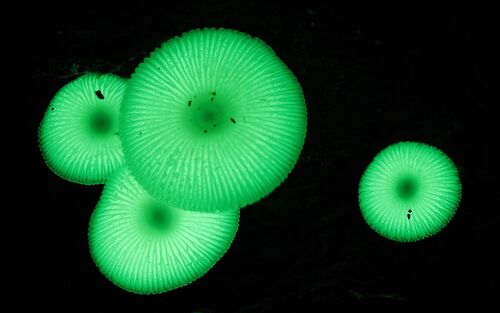
Mycena chlorophos is a bioluminescent fungus primarily found in subtropical Asia (1). It is an aerobe that emits a pale green light from its pileus and gills (Figure 1) and one of sixteen Mycena fungi that emit light (2). Mycena species are characterized by their bell-shaped caps and thin stems (1). Current research on M. chlorophos investigates the mechanism that generates bioluminescence (4). Research on this fungus has potential importance in the medical field, as the bioluminescent feature of M. chlorophos may be used to further develop noninvasive bioimaging techniques (5).
3. Genome structure
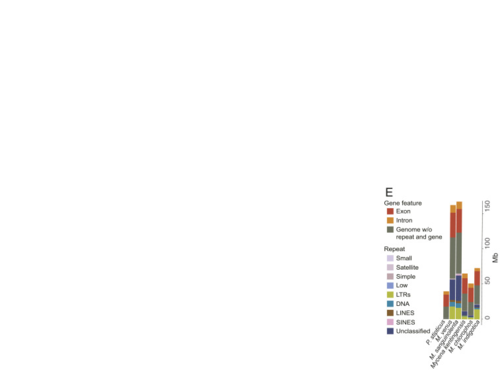
Compared to other fungi within the genus Mycena, M. chlorophos has the smallest genome and proteome (Figure 2E). It also has the highest repeat methylation count of all Mycena species sequenced to date (6).
Repeat sequences make up 11.7% of M. chlorophos’ genome (6). The repeat sequences are long terminal repeats (LTRs), DNA transposons, unclassified repeats, long interspersed nuclear elements (LINES), or simple repeats (Figure 2E). Whole-genome shotgun sequencing found that the M. chlorophos genome is 55.6% GC content and 44.4% AT content (6). In total, the genome is 43.6 million base pairs long and the proteome is 50 Mega base pairs long (6,7).
Two genes, h3h (GenBank: BBH43493.1) and luz (GenBank: LC435377.1), have been identified within M. chlorophos’ metabolic gene clusters (MGC). These MGCs are small and contain relatively recently duplicated genes (9). Luz and h3h are significant in the bioluminescent pathway of M. chlorophos (8) by producing Luciferin, a compound that generates bioluminescence.
4. Cell structure
Figure 3. A depiction of a typical gilled basidiocarp. This image was created in January 2006 by Debivort from English Wikipedia. Image licensed under CC BY-SA 3.0.
M. chlorophos produces basidiospores, which range from 7.0 to 9.0 by 5.0 to 6.0 μm in size (Figure 3). The spores are ellipsoidal and thin walled (2). The pileus marginal cells of M. chlorophos are fusoid to fusoid-ventricose-shaped, ranging from 44 to 100 μm by 12.5 to 23 μm in size. M. chlorophos are dikaryotic and have clamp connections present throughout the hyphae (2).
The distribution of luminescence in M. chlorophos is not homogenous (10). The light intensity in the cap and upper gill region is greater than in the lower gill region. In the stipe, luminescence is weak (10). Microscopically, the luminescence processes are localized to the membrane of the hymenium and basidia cells of the gill. Parts of the luminescence system are also known to be on the surface of the cell membrane (10).
5. Metabolic processes
Mycena chlorophos is an obligate aerobe and contains metabolic gene clusters to produce molecules of the class Luciferin. M. chlorophos has bioluminescent properties which are produced through the oxidative decarboxylation of luciferins (9). The bioluminescence mechanism of M. chlorophos is not fully understood, but is suspected to be activated by the synthesis of trans-3,4-dihydroxycinnamic acid from trans-4-hydroxycinnamic acid via the enzyme hispidin 3-hydroxylase (McH3H). McH3H is dependent on NAD(P)H and contains FAD (8). This mechanism results in fluorescence through the production of an unstable luciferin compound (REF). Digestion of the REF by a luciferase then produces a more stable oxyluciferin and photons with a wavelength of 522 nm (9,10).
6. Ecology
M. chlorophos is indigenous to the tropical regions of Southeastern Asia and the Pacific islands (11). It is mainly found in forests, growing on fallen debris, and is only able to grow in relatively humid conditions (11). Approximately two days after the maturation of the fruiting bodies, the pileus of M. chlorophos is bioluminescent at temperatures of approximately 20°C (10). Under extremely wet conditions, the fruit-bodies of the mushrooms become deformed, and under extremely dry conditions, the mushroom caps become warped and broken (12).
7. Pathology
There is currently no research suggesting any significant pathogenic properties of M. chlorophos. To grow, M. chlorophos feeds off the decaying organic matter found in forests (13). This is typical of the species in the genus Mycena. Research on other Mycena fungi suggests that the luminescent property of the fungi attracts insects, which encourages spore distribution (14).
8. Current Research
One area of current research on M. chlorophos has focused on the bioluminescent property of the fungus. Biochemical and physiological research of the bioluminescent property aims to provide insight into the localization of the bioluminescent reaction. Past research involved studying the distribution of the bioluminescent material in the pileus of M. chlorophos as well as the impact of varying cultural conditions on the light emission and life span of the organism (10). Researchers used a camera and a luminescence spectrometer to determine the intensity of the bioluminescence at different time intervals. It was found that the pileus emitted light for the longest amount of time when conditions were around 20ºC and when there was 90% relative humidity (10). After monitoring the bioluminescence activity of the pileus in response to varying chemicals, researchers were able to find that the light emission of the pileus appeared to be cell and region-specific (10). Currently, riboflavin, riboflavin 5'-monophosphate, and flavin adenine dinucleotide have been identified as possible light emitters in M. chlorophos (3).
A variety of different experiments have also been performed to elucidate the mechanism of fluorescence. Currently, trans-p-hydroxycinnamic acid is believed to induce bioluminescence in M. chlorophos; upon purification of the acid from living gills of M. chlorophos, researchers treated early-stage fruiting bodies of M. chlorophos and found evidence of bioluminescence (1). Trans-4-aminocinnamic acid was also found to inhibit the bioluminescence of the fungus. When the glowing gills of M. chlorophos were exposed to trans-4-aminocinnamic acid, fluorescence decreased (4). Moreover, researchers have also characterized the enzyme responsible for catalyzing the conversion of hispidin to 3-hydroxyhispidin in M. chlorophos, hispidin 3-hydroxylase (h3h). H3h is a commonly conserved enzyme in the Mycena genus and catalyzes the synthesis of a luciferin substrate (8).
9. References
- Teranishi, Katsunori. 2016. Trans-p-Hydroxycinnamic acid as a bioluminescence-activating component in the pileus of the luminous fungus Mycena chlorophos. Tetrahedron 72(5):726-733.
- Desjardin, D. E., Perry, B. A., Lodge, D. J., Stevani, C. V., & Nagasawa, E. 2010. Luminescent Mycena: new and noteworthy species. Mycologia 102(2): 459–77.
- Teranishi, Katsunori. 2016. Identification of possible light emitters in the gills of a bioluminescent fungus Mycena chlorophos. The Journal of Biological and Chemical Luminescence 31(7):1407-1413.
- Teranishi, Katsunori. 2017. Inhibition of bioluminescence in the living gills of the luminous fungus Mycena chlorophos by trans-4-aminocinnamic acid. Biochemical and Biophysical Research Communications 488(2):355-339.
- Syed, A., & J. Anderson. 2021. Applications of bioluminescence in biotechnology and beyond. Chemical Society Reviews 50(9):5668-5705.
- Ke, H., H.H. Lee, C.I. Lin, Y. Liu, M.R. Lu, J.A. Hsieh, C. Chang, P. Wu, M.J. Lu, J. Li, G. Shang, et al. 2020. Mycena genomes resolve the evolution of fungal bioluminescence. Proceedings of the National Academy of Sciences 117(49):31267-31277.
- National Center for Biotechnology Information. 2014. Mycena Chlorophos. DiArk 3.0 Team.
- Tong, Y., M. Trajkovic, S. Savino, W. J.H. van Berkel, and M. Fraaije. 2020. Substrate binding tunes the reactivity of hipidin 3-hydroxylase, a flavoprotein monooxygenase involved in fungal bioluminescence. Enzymology 295(47):16013-16022.
- Slot, Jason C., Gluck-Thaler, Emile. 2019. Metabolic gene clusters, fungal diversity, and the generation of accessory functions. Current Opinion in Genetics & Development 58: 17-24.
- Teranishi, Katsunori. 2015. Localization of the bioluminescence system in the pileus of Mycena chlorophos. The Journal of Biological and Chemical Luminescence 31(2): 514-99.
- Teranishi, Katsunori. 2017. Second bioluminescence-activating component in the luminous fungus Mycena chlorophos. Luminescence 32(2):182-189.
- Sun, Jennifer. 2013. Molecular investigation of the bioluminescent fungus Mycena chlorophos: comparison between a vouchered museum specimen and field samples from Taiwan. [Honors thesis, State University of New York].
- Niitsu, H., & N. Hanyuda. 2000. Fruit-body production of a luminous mushroom, Mycena chlorophos. Mycoscience 41(6):559-564.
- Niitsu, H., N. Hanyuda, and Y. Sugiyama. 2000. Cultural properties of a luminous mushroom, Mycena chlorophos. Mycoscience 41(6):551-558.
- Seas-Carvajal, C., & G. Avalos. 2013. Distribution of bioluminescent fungi across old-growth and secondary tropical rain forest in Costa Rica. Revista de Biologia Tropical 61(2):531-537.
10. Authorship Statement
Author contributions: A.J.H wrote the sections on classification, introduction, and genome structure; D.L.B-H. wrote the sections on pathogenicity and distribution; E.L.T. wrote the section on cell structure; H.N. wrote the section on ecology; K.Q.W. wrote the section on metabolic processes and contributed to genome structure; D.L.B-H. and H.N. wrote the current research section; E.L.T. curated the figures; and A.J.H., D.L.B-H., E.L.T., H.N., and K.Q.W. edited the final article draft.