Porphyromonas Gingivalis and Gum Disease
Introduction and History
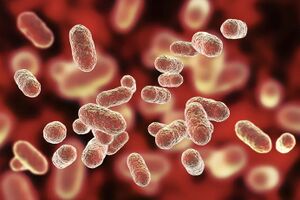
When the typical person hears the term “PG,” their mind usually thinks of a family-friendly movie or age-appropriate content. However, for periodontists, the acronym PG evokes a much different and darker sentiment. Porphyromonas gingivalis, commonly known as PG, is one of the leading causes of periodontal disease worldwide and one of the most destructive forces in dental health today [1]. Periodontal disease, often referred to as gum disease, is frequently caused by PG infections in the gums and bones that support teeth [1]. Gingivitis, redness, and swelling of the gum tissue surrounding the teeth, are among the milder outcomes of such infections, while more persistent infections can lead to the loss of multiple primary teeth and even impact the structural integrity of the jaw bone. According to a recent report, around 47% of adults over the age of 30 have some form of periodontal disease, and this condition is more commonly found in men and people living below the federal poverty line [1].
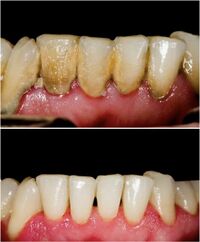
P. gingivalis is an anaerobic, gram-negative bacterium and an integral part of the oral microbiota [2]. It can be found in the oral cavity of all humans and is a relatively small cell, typically about 50 nm in diameter [2]. As an obligate anaerobe, it cannot grow in the presence of oxygen. This rod-shaped bacterium is a chemoorganotroph that obtains energy from organic compounds and can ferment a wide range of carbohydrates, including glucose and fructose [3]. P. gingivalis belongs to the family Porphyromonadaceae, which is in the order of Bacteroidales and the phylum Bacteroidetes [4]. In the world of microbiology, P. gingivalis is known for its many virulence factors that allow it to evade host defenses and penetrate tissues.
The first strain of P. gingivalis, W83, was isolated by a researcher named H. Werner in Bonn, Germany [5]. Werner collected the bacteria from an undocumented oral infection, and the isolated strain was eventually brought to the Pasteur Institute in France by Madeline Sebald [4]. The strain finally made its way to Christian Mouton in Quebec, Canada, in the late 1970s [5]. Throughout this time, this strain was often cultured and subject to intense study [5]. Today, P. gingivalis is still subject to intense research from periodontists, microbiologists, and professionals in various fields.
P. gingivalis is capable of invading periodontal tissues via various adaptations, such as the formation of biofilms and using the intracellular transport mechanism of epithelial cells. Once the invasion begins, P. gingivalis has developed unique adaptations that allow it to manipulate its host's immune system. Although most P. gingivalis make their way to the periodontal pockets, they are impacted by various other organ systems that can exacerbate gum disease in patients. Though the mechanisms for P. gingivalis causing gum disease are relatively well-established, it has recently gained major attention due to its role in aggravating various systemic diseases.
Biofilm Formation and Intracellular Invasion
P. gingivalis, like many bacteria in the oral cavity, utilizes the formation of biofilm to increase its virulence. The oral cavity is composed of a wide range of substrates, including soft tissue, enamel, and even implanted materials. P. gingivalis has developed an intricate mechanism for biofilm formation, allowing it to adhere to a diverse range of materials. The bacterium expresses two types of fimbriae, which are proteinaceous appendages anchored to the outer membrane of bacteria [6]. One of these fimbriae types is long and composed of FimA protein subunits [6]. This FimA fimbriae assists P. gingivalis in its initial attachment to host cells [7]. After associating with the host cell, P. gingivalis coats the surrounding area with capsular polysaccharides [8]. The coating creates a barrier between P. gingivalis and other surface components, such as proteins and lipopolysaccharides [7].
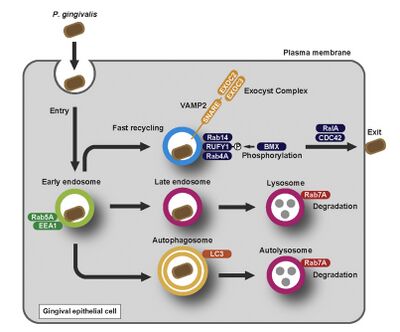
P. gingivalis biofilms tend to be mixed, meaning they also contain other bacterial species. Thus, P. gingivalis has adapted to use quorum sensing as a tool for biofilm formation [8]. Specifically, the LuxS/Autoinducer-2 system allows P. gingivalis to communicate with neighboring species [9]. This communication is essential, as it allows P. gingivalis and its neighboring species to know when they have collectively reached their critical mass. At this point, the mixed bacteria coordinate their extracellular matrix formation at the same time; This coordination dramatically increases the likelihood of successful biofilm formation. P. gingivalis interactions with neighbors are also beneficial from a nutritional standpoint. Another major oral bacterium, T. denticola, often occupies the same areas as P. gingivalis. P. gingivalis secretes isobutyric acid, which enhances the growth of T. denticola [10]. Meanwhile, T. denticola produces succinate, which enhances the growth of P. gingivalis [10]. These interactions may explain why P. gingivalis and T. denticola show enhanced biofilm formation when cultured together [11].
These biofilm formation mechanisms enable P. gingivalis to thrive in subgingival dental plaque. However, this bacterium can also be found in other areas of the periodontal space, such as the epithelial gingival cells of our periodontal tissue [12]. Gingival cells are an important component of our innate immune system, acting as a barrier to prevent bacterial invasion of the periodontium [13]. Despite this, P. gingivalis has been found to penetrate this barrier and invade the deeper tissue layers of the periodontium [1]. In these layers, P. gingivalis faces less pressure from immune defenses, allowing it to proliferate, multiply, and spread into adjacent tissues [14].
The intracellular invasion process of P. gingivalis consists of four steps: adhesion, entry, intracellular trafficking, and exit [15]. First, P. gingivalis uses its FimA fimbriae to attach to the α-5-β-1 integrin on the surface of gingival cells [6]. It then exploits the cellular endocytosis pathways used by other molecules to enter the cell. Once inside the host cell, P. gingivalis disrupts essential cellular functions such as proliferation and cellular migration. After causing severe damage to the function of the gingival cells, P. gingivalis may exit the cell via various exocytotic pathways. Some of these pathways lead to the bacterium being sorted into lytic compartments, such as lysosomes and autolysosomes [16]. Others allow P. gingivalis to enter a recycling pathway, where functional proteins and lipids from the bacteria are utilized by the gingival cells [17].
Sabotaging the Immune System
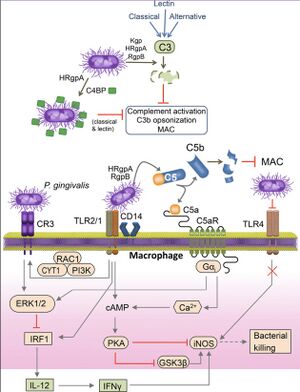
Invasion of bacteria into the periodontal pockets is not the only pathway by which P. gingivalis causes periodontal disease. Once in the host cell, P. gingivalis causes an inflammatory response. However, in deeper layers of the periodontal tissues, these inflammatory responses aren’t as sensitive or dramatic as those at the epithelial surface [18]. One of these fimbriae types is long and composed of FimA protein subunits [6]. Periodontal diseases show that inflammation does occur in these deeper tissues. This is because P. gingivalis is capable of releasing large amounts of endovesicles that often have endotoxins, which further promote an immune response separate from that elicited by the bacterial infection [19]. The immune response to the bacterial infection and the presence of endotoxins lead to a joint immune response to the presence of P. gingivalis. While our immune system is typically sought to protect our bodies from harm, P. gingivalis has developed a survival mechanism that promotes inflammation for its own survival.
Complement component 5 is a protein that is an important element of the complement cascade system, a part of our immune response that assists antibodies and phagocytic cells to eliminate microbes from an organism [20]. It is also the first part of the membrane attack complex, a group of proteins that form a pore-like structure in pathogens [21]. This pore formation severely disrupts the membrane of the pathogen, causing quick cell lysis and death [21]. In a mouse model deficient in C5a, a peptide fragment of the larger C5 protein, P. gingivalis failed to colonize the periodontium [22]. Further, in mice treated with a C5a antagonist, the development of periodontitis was inhibited relative to the control group [23]. In 2014, it was discovered how P. gingivalis uses the C5 protein of our complement immune response to further infect host cells [23]. P. gingivalis is able to associate with macrophages [23]. When the C5 protein is synthesized for the membrane attack complex, P. gingivalis attacks the protein to form C5a and C5b fragments [23]. This increases the presence of C5a fragments, which bind to the C5a receptors of macrophages, otherwise known as white blood cells. Binding to the C5a receptor, under normal circumstances, triggers a sequence of intracellular signaling events that allow it to interact with toll-like receptor 4, or TLR4 [21]. TLR4 is a key protein that aids in the recognition of pathogens within the cellular environment and induces the cell to kill them [24]. With P. gingivalis causing a dramatic increase in the concentration of C5a, the communication between the C5a receptor and TLR4 protein is severely impaired [24].
Before P. gingivalis has infected a cell, the cell's TLR proteins would typically notify the immune system of bacterial presence, and the C5 protein would play a key role in decimating the pathogen [23] [24]. In a complex manipulation of signaling pathways, P. gingivalis flips the script to use the C5 protein to make the C5-TLR communication system ineffective. After P. gingivalis infection, macrophages that were previously meant to eliminate P. gingivalis from the periodontal pockets now become associated with the bacteria. As the macrophages travel across tissues, they now are prone to spreading P. gingivalis to neighboring sites, increasing the virulence of the bacterium. With such function, P. gingivalis is able to use our body's defense mechanisms to increase its spread around teeth and their supporting structures. This is not all bad news, however, as this C5a signaling system opens the door for the development of novel antibiotics [23].
Stress and Loose Teeth
The central nervous system and the oral cavity are in very close proximity, yet neuroscience phenomena and dental conditions are not often referenced in relation to one another. However, in 2017, actress Demi Moore made the claim on The Tonight Show that she lost two of her front teeth due to stress, linking these seemingly distant fields. Although the specifics of Moore’s case are unknown, her claim poses an interesting question: what is the link between stress and tooth loss? One potential explanation may be the link between cortisol and P. gingivalis [25].
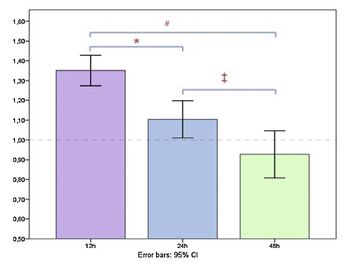
Periodontal disease is a leading contributor to loose teeth and even tooth loss in adults, with P. gingivalis as the main cause [26]. Once P. gingivalis enters and proliferates in the periodontal pocket, it is exposed to gingival crevicular fluid (GCF). GCF is an inflammatory secretion that is produced when the tissues surrounding the periodontal pocket become inflamed [27]. It is composed of anti-inflammatory elements, products of tissue breakdown, and antibodies against the invading bacteria [27]. GCF flows in and out of the gingival pocket in a small stream, transporting only a few microliters every hour [27]. GCF has been studied for its potential diagnostic purposes; Periodontal pathogens like P. gingivalis produce broad-spectrum neutral proteinases that cleave a wide range of proteins [27]. These proteins can be detected in GCF and provide healthcare providers with insight into bacterial infections. One study found that GCF also carries cortisol, a glucocorticoid hormone released by the adrenal glands [28]. Cortisol is primarily involved in the stress response, which is activated by the hypothalamus pituitary adrenal (HPA) axis that travels from the top of the brainstem to our kidneys.
P. gingivalis that have invaded the periodontal pockets are constantly exposed to GCF. During times of stress, when the adrenal glands release a surge of cortisol, the concentration of cortisol in the GCF fluid increases dramatically [28]. A 2014 study found that exposure of P. gingivalis to cortisol increases the growth of the bacteria in a dose-dependent manner [29]. The concentrations used to promote this growth varied and were within the range of cortisol concentrations found in saliva [29]. This finding indicates that during times of stress, cortisol further increases the population of P. gingivalis found in periodontal pockets. Although it was well documented that stress levels can increase the progression of periodontal disease and make treatment options less effective, the mechanisms behind this phenomenon were poorly characterized [30]. This interaction between P. gingivalis and stress may, at least partially, explain this phenomenon in dentistry. While it is unclear whether P. gingivalis was responsible for Moore's tooth loss, the relationship between P. gingivalis and cortisol will certainly impact the way periodontists approach the treatment of bacterial infections.
Alzheimer's and Other Systemic Diseases
P. gingivalis can cause immense damage as it infects the gums and surrounding supports structures of our teeth. When P. gingivalis exits the oral cavity and travels to other organ systems, its potential to cause major health complications increases dramatically. P. gingivalis can enter our blood vasculature during daily activities such as chewing food and brushing our teeth, by traveling through open ulcers that exist on epithelial cells in our oral cavity [31]. Once in the circulatory system, P. gingivalis can accumulate and survive in tissues outside of the oral cavity, such as the plaque tissues of our blood vessels [32]. At the cellular level, P. gingivalis has been found to survive a viable state when cultured in pancreatic and dendritic cells [33]
[34]. P. gingivalis's ability to survive beyond its natural habitat in the oral cavity allows it to impact a wide range of systemic conditions. P. gingivalis has been well-documented to have major impacts on neurological, cardiovascular, and even oncological conditions [35].
As intense scientific research and advancements in medicine prolong expected life expectancy, Alzheimer's disease has become one of the most feared conditions across the globe. Alzheimer's disease is the most common form of dementia and is known for its major symptoms of causing mild memory loss and reduced ability to respond to the environment [36]. In 2020, as many as 5.8 million Americans were living with Alzheimer's disease, and this is projected to grow to 14 million by the year 2060 [36]. In a nationwide retrospective study conducted across the United States, it was found that individuals suffering from chronic periodontitis are at a significantly greater risk of developing Alzheimer's disease [37]. This relationship was found across various age groups, which was key since the risk for periodontal disease and Alzheimer's disease both increase with age [37]. There are two current models for the link between these conditions, one based on an inflammation model and the other based on P. gingivalis virulence factors [38] [39].
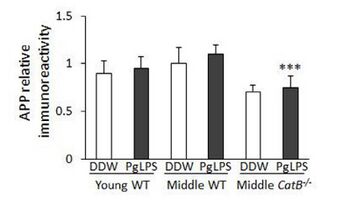
In the inflammatory model, it is hypothesized that in extreme cases of periodontitis, proinflammatory cytokines can enter the systemic circulatory system [38]. These proinflammatory molecules are hypothesized to reach the central nervous system and increase the production of cerebral cytokines [38]. Elevated cytokine levels are known to exacerbate the neuroinflammatory response, which leads to neuron death and the progression of dementia diseases. On the other hand, the P. gingivalis model is based on the bacterium's release of LPS [40]. LPS is a critical component of the cell membrane of gram-negative bacteria. It establishes selective permeability and maintains structural integrity[41]. The injection of LPS of P. gingivalis in mice led to significantly increased Cathepsin B expression in middle-aged mice [42]. Cathepsin B is a lysosomal protease enzyme that plays a role in forming the alpha-beta plaque associated with Alzheimer's disease [42]. After injection, several of these mice also demonstrated AD-like symptoms [42]. This evidence provides a key link between the P. gingivalis virulence factors and the onset of AD. Furthering this hypothesis was the finding that alpha-beta peptide was found in macrophages of P. gingivalis infected mice [43]. This is a key finding, considering how P. gingivalis manipulates the immune system to use macrophages as bacteria transporters rather than bacteria killers [22]. The finding of Cathepsin B in macrophages supports these previous findings. Also, alpha-beta particles being present in macrophages means a potential peripheral source for alpha-beta peptide production, which is most often associated with the amyloid precursor protein found in the brain and other tissues [43]. This bacterial model presents how virulence factors produced for P. gingivalis pathogenesis can have a significant impact on escalating systemic CNS diseases.
P. gingivalis can cause a plethora of issues in the cardiovascular system. One example is when P. gingivalis travels through the bloodstream and accumulates in the plaque tissues of our blood vessels, this causes blood flow to face increased resistance [44]. This increased resistance has a similar impact to vasoconstriction of the blood vessels. Therefore, it makes sense that patients diagnosed with periodontitis (from moderate to severe cases) have an increased likelihood of developing hypertension, or high systolic blood pressure [45]. This association is further supported by studies conducted with mice that have hypertensive responses to angiotensin II. Angiotensin II is a peptide responsible for raising blood pressure in the circulatory system. When these mice were injected with an intraperitoneal injection of P. gingivalis, their response to angiotensin II was exacerbated. Although it is suspected that the immune system and inflammatory response are responsible for the increased blood pressure, hypertension is the summation of many molecular signals and pathways [38]. This impact of P. gingivalis is especially concerning for the Black American community. Periodontitis is more prevalent in individuals living in poverty, and Black Americans currently have the highest poverty rate in the United States [1]. Non-Hispanic Black Americans also suffer from the largest death rates from heart disease. Although these death rates are largely a result of massive health disparities in the United States, it does not change the fact that Black Americans afflicted with poverty are particularly prone to cardiovascular diseases stemming from periodontitis [1].
Finally, P. gingivalis even plays a role in the progression of one of the most aggressive forms of cancer: esophageal cancer [46]. One study found that the presence of periodontal disease was positively correlated with the risk for esophageal cancer [47]. This same study also found that patients with esophageal cancer and gum disease simultaneously had reduced rates of survival [45]. A likely mechanism behind this relationship is rooted in a P. gingivalis dehydrogenation reaction [33]. P. gingivalis is capable of dehydrogenating ethanol into acetaldehyde [39], which is a carcinogen. This means that it is capable of causing DNA damage that can lead to cancerous cells [39] [47]
Conclusion
There is currently no reliable data on the trends of periodontitis prevalence across the United States, as surveys in the past included partial-mouth periodontal examinations, resulting in a significant portion of missed cases [48]. However, research into the molecular basis for periodontitis has become increasingly detailed, and every year new targets for targeting P. Gingivalis infections are being discovered. These targets could lead to the discovery of antibiotics that are better able to eliminate P. Gingivalis from the periodontal cavities, regardless of the numerous mechanisms it has developed to bypass our body's immune system. The quicker these medications are developed, the sooner the quality of life of the older population can be improved. The recent discoveries of the impact of P. Gingivalis are exciting to researchers, but they are a harsh reality for those prone to gum disease. Dental care is becoming increasingly less affordable, and people suffering from poverty will continue to receive inadequate care. As this trend continues, we can expect gum disease to become a condition relegated to marginalized communities, which are already at a severe disadvantage when it comes to access to and quality of healthcare.
Future directions for P. Gingivalis studies should include collaborations between periodontists, physicians, and microbiologists alike. The better society understands P. Gingivalis infections, the less they will be viewed as a localized phenomenon. With a focus on developing preventative measures and understanding P. Gingivalis's role in systemic diseases, healthcare providers can get ahead of the infection spread and potentially save lives.
References
1) Centers for Disease Control and Prevention. (2013, July 10). Periodontal disease. Centers for Disease Control and Prevention. Retrieved April 16, 2023, from https://www.cdc.gov/oralhealth/conditions/periodontal-disease.html#periodontal
2) Xie, H. (2015). Biogenesis and function of Porphyromonas gingivalis outer membrane vesicles. Future microbiology, 10(9), 1517-1527.
3) Hendrickson, E. L., Xia, Q., Wang, T., Lamont, R. J., & Hackett, M. (2009). Pathway analysis for intracellular Porphyromonas gingivalis using a strain ATCC 33277 specific database. BMC microbiology, 9, 1-10.
4) UniProt. (n.d.). Taxonomy - Porphyromonas gingivalis (species). UniProt BLAST. Retrieved April 16, 2023, from https://www.uniprot.org/taxonomy/837
5) Nelson, K. E., Fleischmann, R. D., DeBoy, R. T., Paulsen, I. T., Fouts, D. E., Eisen, J. A., ... & Fraser, C. M. (2003). Complete genome sequence of the oral pathogenic bacterium Porphyromonas gingivalis strain W83. Journal of bacteriology, 185(18), 5591-5601.
6) Dominy, S. S., Lynch, C., Ermini, F., Benedyk, M., Marczyk, A., Konradi, A., ... & Potempa, J. (2019). Porphyromonas gingivalis in Alzheimer’s disease brains: Evidence for disease causation and treatment with small-molecule inhibitors. Science advances, 5(1), eaau3333.
7) Kuboniwa, M., Amano, A., Inaba, H., Hashino, E., & Shizukuishi, S. (2009). Homotypic biofilm structure of Porphyromonas gingivalis is affected by FimA type variations. Oral microbiology and immunology, 24(3), 260-263.
8) Hamada, N., Watanabe, K., Sasakawa, C., Yoshikawa, M., Yoshimura, F., & Umemoto, T. (1994). Construction and characterization of a fimA mutant of Porphyromonas gingivalis. Infection and immunity, 62(5), 1696-1704.
9) Gerits, E., Verstraeten, N., & Michiels, J. (2017). New approaches to combat Porphyromonas gingivalis biofilms. Journal of oral microbiology, 9(1), 1300366.
10) Chung, W. O., Park, Y., Lamont, R. J., McNab, R., Barbieri, B., & Demuth, D. R. (2001). Signaling system in Porphyromonas gingivalis based on a LuxS protein. Journal of bacteriology, 183(13), 3903-3909.
11) Grenier, D. (1992). Nutritional interactions between two suspected periodontopathogens, Treponema denticola and Porphyromonas gingivalis. Infection and immunity, 60(12), 5298-5301.
12) Yamada, M., Ikegami, A., & Kuramitsu, H. K. (2005). Synergistic biofilm formation by Treponema denticola and Porphyromonas gingivalis. FEMS microbiology letters, 250(2), 271-277.
13) Sakanaka, A., Takeuchi, H., Kuboniwa, M., & Amano, A. (2016). Dual lifestyle of Porphyromonas gingivalis in biofilm and gingival cells. Microbial pathogenesis, 94, 42-47.
14) Amano, A. (2007). Disruption of epithelial barrier and impairment of cellular function by Porphyromonas gingivalis. Frontiers in Bioscience-Landmark, 12(10), 3965-3974.
15) Lamont, R. J., & Yilmaz, O. (2002). In or out: the invasiveness of oral bacteria. Periodontology 2000, 30(1), 61-69.
16) Casadevall, A. (2008). Evolution of intracellular pathogens. Annu. Rev. Microbiol., 62, 19-33.
17) Tsuda, K., Furuta, N., Inaba, H., Kawai, S., Hanada, K., Yoshimori, T., & Amano, A. (2008). Functional analysis of α5β1 integrin and lipid rafts in invasion of epithelial cells by Porphyromonas gingivalis using fluorescent beads coated with bacterial membrane vesicles. Cell structure and function, 33(1), 123-132.
18) Takeuchi, H., Furuta, N., Morisaki, I., & Amano, A. (2011). Exit of intracellular Porphyromonas gingivalis from gingival epithelial cells is mediated by endocytic recycling pathway. Cellular microbiology, 13(5), 677-691.
19) How, K. Y., Song, K. P., & Chan, K. G. (2016). Porphyromonas gingivalis: an overview of periodontopathic pathogen below the gum line. Frontiers in microbiology, 7, 53.
20) Roier, S., Zingl, F. G., Cakar, F., Durakovic, S., Kohl, P., Eichmann, T. O., ... & Schild, S. (2016). A novel mechanism for the biogenesis of outer membrane vesicles in Gram-negative bacteria. Nature communications, 7(1), 10515.
21) Hajishengallis, G., & Lambris, J. D. (2016). More than complementing Tolls: complement–Toll‐like receptor synergy and crosstalk in innate immunity and inflammation. Immunological reviews, 274(1), 233-244.
22) Bayly-Jones, C., Bubeck, D., & Dunstone, M. A. (2017). The mystery behind membrane insertion: a review of the complement membrane attack complex. Philosophical Transactions of the Royal Society B: Biological Sciences, 372(1726), 20160221.
23) Hajishengallis, G., Liang, S., Payne, M. A., Hashim, A., Jotwani, R., Eskan, M. A., ... & Curtis, M. A. (2011). Low-abundance biofilm species orchestrates inflammatory periodontal disease through the commensal microbiota and complement. Cell host & microbe, 10(5), 497-506.
24) Abe, T., Hosur, K. B., Hajishengallis, E., Reis, E. S., Ricklin, D., Lambris, J. D., & Hajishengallis, G. (2012). Local complement-targeted intervention in periodontitis: proof-of-concept using a C5a receptor (CD88) antagonist. The Journal of Immunology, 189(11), 5442-5448.
25) Hajishengallis, G., & Lamont, R. J. (2014). Breaking bad: Manipulation of the host response by P orphyromonas gingivalis. European journal of immunology, 44(2), 328-338.
26) Vaure, C., & Liu, Y. (2014). A comparative review of toll-like receptor 4 expression and functionality in different animal species. Frontiers in immunology, 5, 316.
27) Beniaris, K. (2018, April 25). Demi Moore just revealed how she lost her front two teeth. Woman's Day. Retrieved April 16, 2023, from https://www.womansday.com/life/a59311/demi-moore-teeth-stress/
28) Mayo Foundation for Medical Education and Research. (2023, February 24). Periodontitis. Mayo Clinic. Retrieved April 16, 2023, from https://www.mayoclinic.org/diseases-conditions/periodontitis/symptoms-causes/syc-20354473
29) Subbarao, K. C., Nattuthurai, G. S., Sundararajan, S. K., Sujith, I., Joseph, J., & Syedshah, Y. P. (2019). Gingival crevicular fluid: an overview. Journal of pharmacy & bioallied sciences, 11(Suppl 2), S135.
30) Axtelius, B., Edwardsson, S., Theodorsson, E., Svensäter, G., & Attström, R. (1998). Presence of cortisol in gingival crevicular fluid. A pilot study. Journal of clinical periodontology, 25(11 Pt 1), 929–932. https://doi.org/10.1111/j.1600-051x.1998.tb02392.x
31) Akcalı, A., Huck, O., Buduneli, N., Davideau, J. L., Köse, T., & Tenenbaum, H. (2014). Exposure of Porphyromonas gingivalis to cortisol increases bacterial growth. Archives of oral biology, 59(1), 30–34. https://doi.org/10.1016/j.archoralbio.2013.09.003
32) Periodontal diseases and stress: a brief review. Journal of oral rehabilitation, 40(1), 60–68. https://doi.org/10.1111/j.1365-2842.2012.02341.x
33) Forner, L., Larsen, T., Kilian, M., & Holmstrup, P. (2006). Incidence of bacteremia after chewing, tooth brushing and scaling in individuals with periodontal inflammation. Journal of clinical periodontology, 33(6), 401–407. https://doi.org/10.1111/j.1600-051X.2006.00924.x
34) Kozarov, E. V., Dorn, B. R., Shelburne, C. E., Dunn Jr, W. A., & Progulske-Fox, A. (2005). Human atherosclerotic plaque contains viable invasive Actinobacillus actinomycetemcomitans and Porphyromonas gingivalis. Arteriosclerosis, thrombosis, and vascular biology, 25(3), e17-e18.
35) Gnanasekaran, J., Binder Gallimidi, A., Saba, E., Pandi, K., Eli Berchoer, L., Hermano, E., ... & Nussbaum, G. (2020). Intracellular porphyromonas gingivalis promotes the tumorigenic behavior of pancreatic carcinoma cells. Cancers, 12(8), 2331.
36) El-Awady, A. R., Miles, B., Scisci, E., Kurago, Z. B., Palani, C. D., Arce, R. M., ... & Cutler, C. W. (2015). Porphyromonas gingivalis evasion of autophagy and intracellular killing by human myeloid dendritic cells involves DC-SIGN-TLR2 crosstalk. PLoS pathogens, 11(2), e1004647.
37) Mei, Feng, Mengru Xie, Xiaofei Huang, Yanlin Long, Xiaofeng Lu, Xiaoli Wang, and Lili Chen. "Porphyromonas gingivalis and its systemic impact: Current status." Pathogens 9, no. 11 (2020): 944.
38) Chen, C. K., Wu, Y. T., & Chang, Y. C. (2017). Association between chronic periodontitis and the risk of Alzheimer’s disease: A retrospective, population-based, matched-cohort study. Alzheimer's research & therapy, 9, 1-7.
39) Watts, A., Crimmins, E. M., & Gatz, M. (2008). Inflammation as a potential mediator for the association between periodontal disease and Alzheimer’s disease. Neuropsychiatric Disease and Treatment, 4(5), 865-876.
40) Alexander, C., & Rietschel, E. T. (2001). Invited review: Bacterial lipopolysaccharides and innate immunity. Journal of endotoxin research, 7(3), 167-202.
41) Jain, S., & Darveau, R. P. (2010). Contribution of Porphyromonas gingivalis lipopolysachharide to periodontitis. Periodontology 2000, 54(1), 53.
42) Wu, Z., Ni, J., Liu, Y., Teeling, J. L., Takayama, F., Collcutt, A., Ibbett, P., & Nakanishi, H. (2017). Cathepsin B plays a critical role in inducing Alzheimer's disease-like phenotypes following chronic systemic exposure to lipopolysaccharide from Porphyromonas gingivalis in mice. Brain, behavior, and immunity, 65, 350–361. https://doi.org/10.1016/j.bbi.2017.06.002
43) Nie, R., Wu, Z., Ni, J., Zeng, F., Yu, W., Zhang, Y., Kadowaki, T., Kashiwazaki, H., Teeling, J. L., & Zhou, Y. (2019). Porphyromonas gingivalis Infection Induces Amyloid-β Accumulation in Monocytes/Macrophages. Journal of Alzheimer's disease : JAD, 72(2), 479–494. https://doi.org/10.3233/JAD-190298
44) Munoz Aguilera, E., Suvan, J., Buti, J., Czesnikiewicz-Guzik, M., Barbosa Ribeiro, A., Orlandi, M., ... & D’Aiuto, F. (2020). Periodontitis is associated with hypertension: a systematic review and meta-analysis. Cardiovascular research, 116(1), 28-39.
45) Czesnikiewicz-Guzik, M., Nosalski, R., Mikolajczyk, T. P., Vidler, F., Dohnal, T., Dembowska, E., Graham, D., Harrison, D. G., & Guzik, T. J. (2019). Th1-type immune responses to Porphyromonas gingivalis antigens exacerbate angiotensin II-dependent hypertension and vascular dysfunction. British journal of pharmacology, 176(12), 1922–1931. https://doi.org/10.1111/bph.14536
46) (n.d.). Science-In-Brief Feature: Understanding Health Disparities in Cardiovascular Disease. Retrieved April 2023, from https://www.cdc.gov/dhdsp/pubs/docs/sib_feature_nov2014.pdf.
47) Gao, S., Li, S., Ma, Z., Liang, S., Shan, T., Zhang, M., Zhu, X., Zhang, P., Liu, G., Zhou, F., Yuan, X., Jia, R., Potempa, J., Scott, D. A., Lamont, R. J., Wang, H., & Feng, X. (2016). Presence of Porphyromonas gingivalis in esophagus and its association with the clinicopathological characteristics and survival in patients with esophageal cancer. Infectious agents and cancer, 11, 3. https://doi.org/10.1186/s13027-016-0049-x
48) Yokoyama, A., Muramatsu, T., Ohmori, T., Higuchi, S., Hayashida, M., & Ishii, H. (1996). Esophageal cancer and aldehyde dehydrogenase-2 genotypes in Japanese males. Cancer epidemiology, biomarkers & prevention : a publication of the American Association for Cancer Research, cosponsored by the American Society of Preventive Oncology, 5(2), 99–102.
49) Eke, P. I., Borgnakke, W. S., & Genco, R. J.. (2020). Recent epidemiologic trends in periodontitis in the USA. Periodontology 2000, 82(1), 257–267. https://doi.org/10.1111/prd.12323
Authored for BIOL 238 Microbiology, taught by Joan Slonczewski, 2023, Kenyon College
- ↑ 1.0 1.1 1.2 1.3 1.4 1.5 [Centers for Disease Control and Prevention. (2013, July 10). Periodontal disease. Centers for Disease Control and Prevention. Retrieved April 16, 2023, from https://www.cdc.gov/oralhealth/conditions/periodontal-disease.html#periodontal
] Cite error: Invalid
<ref>
tag; name "CDC" defined multiple times with different content - ↑ 2.0 2.1 [Xie, H. (2015). Biogenesis and function of Porphyromonas gingivalis outer membrane vesicles. Future microbiology, 10(9), 1517-1527.]
- ↑ [Hendrickson, E. L., Xia, Q., Wang, T., Lamont, R. J., & Hackett, M. (2009). Pathway analysis for intracellular Porphyromonas gingivalis using a strain ATCC 33277 specific database. BMC microbiology, 9, 1-10.]
- ↑ 4.0 4.1 [UniProt. (n.d.). Taxonomy - Porphyromonas gingivalis (species). UniProt BLAST. Retrieved April 16, 2023, from https://www.uniprot.org/taxonomy/837]
- ↑ 5.0 5.1 5.2 [Nelson, K. E., Fleischmann, R. D., DeBoy, R. T., Paulsen, I. T., Fouts, D. E., Eisen, J. A., ... & Fraser, C. M. (2003). Complete genome sequence of the oral pathogenic bacterium Porphyromonas gingivalis strain W83. Journal of bacteriology, 185(18), 5591-5601.]
- ↑ 6.0 6.1 6.2 6.3 Kuboniwa, M., Amano, A., Inaba, H., Hashino, E., & Shizukuishi, S. (2009). Homotypic biofilm structure of Porphyromonas gingivalis is affected by FimA type variations. Oral microbiology and immunology, 24(3), 260-263.
- ↑ 7.0 7.1 Hamada, N., Watanabe, K., Sasakawa, C., Yoshikawa, M., Yoshimura, F., & Umemoto, T. (1994). Construction and characterization of a fimA mutant of Porphyromonas gingivalis. Infection and immunity, 62(5), 1696-1704.
- ↑ 8.0 8.1 Gerits, E., Verstraeten, N., & Michiels, J. (2017). New approaches to combat Porphyromonas gingivalis biofilms. Journal of oral microbiology, 9(1), 1300366.
- ↑ [Chung, W. O., Park, Y., Lamont, R. J., McNab, R., Barbieri, B., & Demuth, D. R. (2001). Signaling system in Porphyromonas gingivalis based on a LuxS protein. Journal of bacteriology, 183(13), 3903-3909. ]
- ↑ 10.0 10.1 Grenier, D. (1992). Nutritional interactions between two suspected periodontopathogens, Treponema denticola and Porphyromonas gingivalis. Infection and immunity, 60(12), 5298-5301.
- ↑ [Yamada, M., Ikegami, A., & Kuramitsu, H. K. (2005). Synergistic biofilm formation by Treponema denticola and Porphyromonas gingivalis. FEMS microbiology letters, 250(2), 271-277.]
- ↑ Lamont, R. J., Chan, A., Belton, C. M., Izutsu, K. T., Vasel, D., & Weinberg, A. (1995). Porphyromonas gingivalis invasion of gingival epithelial cells. Infection and immunity, 63(10), 3878-3885.
- ↑ [Sakanaka, A., Takeuchi, H., Kuboniwa, M., & Amano, A. (2016). Dual lifestyle of Porphyromonas gingivalis in biofilm and gingival cells. Microbial pathogenesis, 94, 42-47.]
- ↑ [Amano, A. (2007). Disruption of epithelial barrier and impairment of cellular function by Porphyromonas gingivalis. Frontiers in Bioscience-Landmark, 12(10), 3965-3974. ]
- ↑ [Lamont, R. J., & Yilmaz, O. (2002). In or out: the invasiveness of oral bacteria. Periodontology 2000, 30(1), 61-69.]
- ↑ [Tsuda, K., Furuta, N., Inaba, H., Kawai, S., Hanada, K., Yoshimori, T., & Amano, A. (2008). Functional analysis of α5β1 integrin and lipid rafts in invasion of epithelial cells by Porphyromonas gingivalis using fluorescent beads coated with bacterial membrane vesicles. Cell structure and function, 33(1), 123-132.]
- ↑ [Takeuchi, H., Furuta, N., Morisaki, I., & Amano, A. (2011). Exit of intracellular Porphyromonas gingivalis from gingival epithelial cells is mediated by endocytic recycling pathway. Cellular microbiology, 13(5), 677-691.]
- ↑ [Takeuchi, H., Furuta, N., Morisaki, I., & Amano, A. (2011). Exit of intracellular Porphyromonas gingivalis from gingival epithelial cells is mediated by endocytic recycling pathway. Cellular microbiology, 13(5), 677-691.]
- ↑ [How, K. Y., Song, K. P., & Chan, K. G. (2016). Porphyromonas gingivalis: an overview of periodontopathic pathogen below the gum line. Frontiers in microbiology, 7, 53.]
- ↑ [Roier, S., Zingl, F. G., Cakar, F., Durakovic, S., Kohl, P., Eichmann, T. O., ... & Schild, S. (2016). A novel mechanism for the biogenesis of outer membrane vesicles in Gram-negative bacteria. Nature communications, 7(1), 10515.]
- ↑ 21.0 21.1 21.2 The mystery behind membrane insertion: a review of the complement membrane attack complex. Philosophical Transactions of the Royal Society B: Biological Sciences, 372(1726), 20160221.
- ↑ 22.0 22.1 Hajishengallis, G., Liang, S., Payne, M. A., Hashim, A., Jotwani, R., Eskan, M. A., ... & Curtis, M. A. (2011). Low-abundance biofilm species orchestrates inflammatory periodontal disease through the commensal microbiota and complement. Cell host & microbe, 10(5), 497-506.
- ↑ 23.0 23.1 23.2 23.3 23.4 Abe, T., Hosur, K. B., Hajishengallis, E., Reis, E. S., Ricklin, D., Lambris, J. D., & Hajishengallis, G. (2012). Local complement-targeted intervention in periodontitis: proof-of-concept using a C5a receptor (CD88) antagonist. The Journal of Immunology, 189(11), 5442-5448.
- ↑ 24.0 24.1 24.2 Hajishengallis, G., & Lamont, R. J. (2014). Breaking bad: Manipulation of the host response by P orphyromonas gingivalis. European journal of immunology, 44(2), 328-338.
- ↑ Beniaris, K. (2018, April 25). Demi Moore just revealed how she lost her front two teeth. Woman's Day. Retrieved April 16, 2023, from https://www.womansday.com/life/a59311/demi-moore-teeth-stress/
- ↑ Mayo Foundation for Medical Education and Research. (2023, February 24). Periodontitis. Mayo Clinic. Retrieved April 16, 2023, from https://www.mayoclinic.org/diseases-conditions/periodontitis/symptoms-causes/syc-20354473
- ↑ 27.0 27.1 27.2 27.3 Subbarao, K. C., Nattuthurai, G. S., Sundararajan, S. K., Sujith, I., Joseph, J., & Syedshah, Y. P. (2019). Gingival crevicular fluid: an overview. Journal of pharmacy & bioallied sciences, 11(Suppl 2), S135.
- ↑ 28.0 28.1 Axtelius, B., Edwardsson, S., Theodorsson, E., Svensäter, G., & Attström, R. (1998). Presence of cortisol in gingival crevicular fluid. A pilot study. Journal of clinical periodontology, 25(11 Pt 1), 929–932. https://doi.org/10.1111/j.1600-051x.1998.tb02392.x
- ↑ 29.0 29.1 Akcalı, A., Huck, O., Buduneli, N., Davideau, J. L., Köse, T., & Tenenbaum, H. (2014). Exposure of Porphyromonas gingivalis to cortisol increases bacterial growth. Archives of oral biology, 59(1), 30–34. https://doi.org/10.1016/j.archoralbio.2013.09.003
- ↑ Periodontal diseases and stress: a brief review. Journal of oral rehabilitation, 40(1), 60–68. https://doi.org/10.1111/j.1365-2842.2012.02341.x
- ↑ Forner, L., Larsen, T., Kilian, M., & Holmstrup, P. (2006). Incidence of bacteremia after chewing, tooth brushing and scaling in individuals with periodontal inflammation. Journal of clinical periodontology, 33(6), 401–407. https://doi.org/10.1111/j.1600-051X.2006.00924.x
- ↑ Kozarov, E. V., Dorn, B. R., Shelburne, C. E., Dunn Jr, W. A., & Progulske-Fox, A. (2005). Human atherosclerotic plaque contains viable invasive Actinobacillus actinomycetemcomitans and Porphyromonas gingivalis. Arteriosclerosis, thrombosis, and vascular biology, 25(3), e17-e18.
- ↑ 33.0 33.1 Gnanasekaran, J., Binder Gallimidi, A., Saba, E., Pandi, K., Eli Berchoer, L., Hermano, E., ... & Nussbaum, G. (2020). Intracellular porphyromonas gingivalis promotes the tumorigenic behavior of pancreatic carcinoma cells. Cancers, 12(8), 2331.
- ↑ El-Awady, A. R., Miles, B., Scisci, E., Kurago, Z. B., Palani, C. D., Arce, R. M., ... & Cutler, C. W. (2015). Porphyromonas gingivalis evasion of autophagy and intracellular killing by human myeloid dendritic cells involves DC-SIGN-TLR2 crosstalk. PLoS pathogens, 11(2), e1004647.
- ↑ Mei, Feng, Mengru Xie, Xiaofei Huang, Yanlin Long, Xiaofeng Lu, Xiaoli Wang, and Lili Chen. "Porphyromonas gingivalis and its systemic impact: Current status." Pathogens 9, no. 11 (2020): 944.
- ↑ 36.0 36.1 Centers for Disease Control and Prevention. (2020, October 26). What is alzheimer's disease? Centers for Disease Control and Prevention. Retrieved April 16, 2023, from https://www.cdc.gov/aging/aginginfo/alzheimers.htm#:~:text=Alzheimer's%20disease%20is%20the%20most,thought%2C%20memory%2C%20and%20language
- ↑ 37.0 37.1 Chen, C. K., Wu, Y. T., & Chang, Y. C. (2017). Association between chronic periodontitis and the risk of Alzheimer’s disease: A retrospective, population-based, matched-cohort study. Alzheimer's research & therapy, 9, 1-7.
- ↑ 38.0 38.1 38.2 38.3 Watts, A., Crimmins, E. M., & Gatz, M. (2008). Inflammation as a potential mediator for the association between periodontal disease and Alzheimer’s disease. Neuropsychiatric Disease and Treatment, 4(5), 865-876.
- ↑ 39.0 39.1 39.2 Fiorillo, L., Cervino, G., Laino, L., D’Amico, C., Mauceri, R., Tozum, T. F., Gaeta, M., & Cicciù, M.. (2019). Porphyromonas gingivalis, Periodontal and Systemic Implications: A Systematic Review. Dentistry Journal, 7(4), 114. https://doi.org/10.3390/dj7040114
- ↑ ["Jain, S., & Darveau, R. P. (2010). Contribution of Porphyromonas gingivalis lipopolysachharide to periodontitis. Periodontology 2000, 54(1), 53."]
- ↑ ["Alexander, C., & Rietschel, E. T. (2001). Invited review: Bacterial lipopolysaccharides and innate immunity. Journal of endotoxin research, 7(3), 167-202."]
- ↑ 42.0 42.1 42.2 Wu, Z., Ni, J., Liu, Y., Teeling, J. L., Takayama, F., Collcutt, A., Ibbett, P., & Nakanishi, H. (2017). Cathepsin B plays a critical role in inducing Alzheimer's disease-like phenotypes following chronic systemic exposure to lipopolysaccharide from Porphyromonas gingivalis in mice. Brain, behavior, and immunity, 65, 350–361. https://doi.org/10.1016/j.bbi.2017.06.002
- ↑ 43.0 43.1 Nie, R., Wu, Z., Ni, J., Zeng, F., Yu, W., Zhang, Y., Kadowaki, T., Kashiwazaki, H., Teeling, J. L., & Zhou, Y. (2019). Porphyromonas gingivalis Infection Induces Amyloid-β Accumulation in Monocytes/Macrophages. Journal of Alzheimer's disease : JAD, 72(2), 479–494. https://doi.org/10.3233/JAD-190298
- ↑ Cite error: Invalid
<ref>
tag; no text was provided for refs namedSubbarao
- ↑ 45.0 45.1 Munoz Aguilera, E., Suvan, J., Buti, J., Czesnikiewicz-Guzik, M., Barbosa Ribeiro, A., Orlandi, M., ... & D’Aiuto, F. (2020). Periodontitis is associated with hypertension: a systematic review and meta-analysis. Cardiovascular research, 116(1), 28-39.
- ↑ Gao, S., Li, S., Ma, Z., Liang, S., Shan, T., Zhang, M., Zhu, X., Zhang, P., Liu, G., Zhou, F., Yuan, X., Jia, R., Potempa, J., Scott, D. A., Lamont, R. J., Wang, H., & Feng, X. (2016). Presence of Porphyromonas gingivalis in esophagus and its association with the clinicopathological characteristics and survival in patients with esophageal cancer. Infectious agents and cancer, 11, 3. https://doi.org/10.1186/s13027-016-0049-x
- ↑ 47.0 47.1 Yokoyama, A., Muramatsu, T., Ohmori, T., Higuchi, S., Hayashida, M., & Ishii, H. (1996). Esophageal cancer and aldehyde dehydrogenase-2 genotypes in Japanese males. Cancer epidemiology, biomarkers & prevention : a publication of the American Association for Cancer Research, cosponsored by the American Society of Preventive Oncology, 5(2), 99–102.
- ↑ Eke, P. I., Borgnakke, W. S., & Genco, R. J.. (2020). Recent epidemiologic trends in periodontitis in the USA. Periodontology 2000, 82(1), 257–267. https://doi.org/10.1111/prd.12323