Horseshoe Crab: the living fossil
Introduction
Witness to almost 500 million years of Earth's history, horseshoe crabs are some of the most ancestral species of animals still alive today. Their general morphology, featuring the iconic spine-like telson and horseshoe-shaped carapace, have remained virtually unchanged across their existence, awarding them the epithet "living fossils".[1] While their resemblance to a horseshoe is difficult to challenge, “crab” is a misnomer for the creatures considering they are not even crustaceans. The characteristic blue blood of the horseshoe crabs is used for endotoxin detection, making them indispensable for preserving human health. However, their existence is at threat owing to unsustainable harvesting, climate change, habitat loss, pollution, and more.[2]
Phylogeny and Evolution
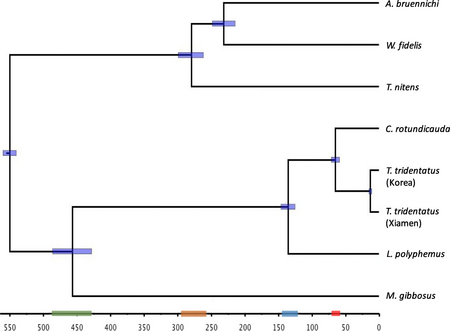
Modern-day horseshoe crabs are marine-dwelling chelicerate arthropods of the Limulidae family. Despite their name, they are not crustaceans at all and are, in fact, more closely related to arachnids. Of 88 formally described species, only 4 remain today and are the only surviving members of the order Xiphosura. The extant species include the Atlantic horseshoe crab Limulus polyphemus, and three Asian species: the Indo-Pacific horseshoe crab Tachypleus gigas, the tri-spine horseshoe crab Tachypleus tridentatus, and the mangrove horseshoe crab Carcinoscorpius rotundicauda.
Xiphosurans were considered to have first emerged in the Late Ordovician period of the Paleozoic era, some 450 million years ago, following the discovery and characterization of the Lunataspis aurora fossil in Canada back in 2007.[4] However, yet undescribed fossils from Morocco, discovered in 2010, established a deeper origin for the Xiphosurans, about 470 million years ago in the Lower Ordovician period.[5] The Limulids, the family of modern horseshoe crabs, first appeared around 250 years ago in the Triassic period. Limulus polyphemus is considered to be the sister taxon, or the closest relative to the three Asian species. The split between the Atlantic and Asian branches of the extant crab species is estimated to have occurred around 135 million years ago and is attributed to a whole-genome duplication event. The Asian species diverged in the Paleogene era, around 45 million years ago, via allopatric speciation.[6] Strikingly, none of the modern species have left a fossil record so far.[7] Horseshoe crabs are commonly dubbed living fossils owing to the little morphological variation they have undergone across their existence, a phenomenon termed evolutionary stasis. Two rounds of evolutionary stasis have been identified in the Xiphosurans, the conservation of prosomal and opisthosomal growth rates near their origin in the Lower Ordivician and the conservation of shape in the Jurassic period owing to the emergence of the Limulids in the Triassic. Notwithstanding physiological changes, present-day crabs are larger in size than their ancestral counterparts and have their abdominal segments fused together.[1]
Whole-genome Duplication
Horseshoe crabs have markedly large genomes for invertebrates; for instance, the species Carcinoscorpius rotundicauda and Tachypleus tridentatus each have genomes approximately 1.72 Gigabase pairs long. This size is attributed to at least three whole-genome duplication events. Whole-genome duplication is a mutation event, relatively rare in animals, which results in the doubling of an entire genome. The redundant copies of genes are often degraded but sometimes, they acquire novel functions and drive dramatic evolutionary changes.[8] Evidence of whole-genome duplication in horseshoe crabs comes from the existence of multiple fully duplicated hox gene clusters, which, so far, have only been observed in the genomes of species that have undergone whole-genome duplication. Invertebrates typically possess a single hox gene cluster and as such, the number of hox gene clusters in an invertebrate’s genome can be used to predict the number of whole-genome duplication events that might have occurred.[9] Hox genes refer to a class of homeobox genes, which encode transcription factors that specify species shape and regulate development in animals. All hox genes are homologs descended from an ancestral gene.[10] Researchers have identified five Hox clusters in Carcinoscorpius rotundicauda and Tachypleus gigas (the highest among any invertebrates), four in Limulus polyphemus and three in Tachypleus tridentatus. These findings have led researchers to propose three rounds of whole-genome duplication having occurred across the lineage of the crabs.[11]
Whole-genome duplication events are commonly associated with dramatic consequences like speciation and extraordinary morphological diversity. The evolutionary stasis observed in the horseshoe crabs is thus at odds with the several rounds of whole-genome duplication they have undergone. The curious case of the crabs suggests that whole-genome duplication events alone are not sufficient to bring about dramatic morphological changes across various species. As the crabs remained limited to their marine coastal habitats and faced no natural predation, they faced no significant evolutionary pressures which could have caused a shift in their perfectly suitable forms. Regardless, the events have had notable consequences for the crabs’ physiology. Scientists have identified a marked expansion of gene families with important roles in the crabs’ innate immunity, such as carcinolectin-5, carcinolectin-3, CEBP-PI, and FucTC. Horseshoe crabs are constantly exposed to pathogens in their coastal habitats and as such, building up strong defenses was crucial to the animals’ survival.[9]
Morphology
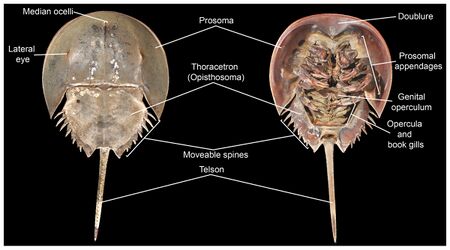
All horseshoe crabs share the same body plan: the cephalothorax or prosoma (head and chest fused together), the opisthosoma (the abdomen with the inclusion of the heart and respiratory organs, a distinctive feature of the chelicerates) and, the iconic feature of the horseshoe crab, the telson (the long tail-like spine jutting out from the abdomen). The prosoma is shrouded in a protective carapace bearing an uncanny resemblance to a horseshoe, giving the horseshoe crab its name. Unlike any other extant chelicerate, horseshoe crabs possess, among its ten eyes, two compound eyes that sit atop its carapace; they also have the largest rod and cone cells of any known animal. All extant species exhibit sexual dimorphism, with female individuals being nearly 1.3 times larger than males. This size difference suits the crabs' particular mating behavior, whereby males attach themselves onto the females' carapaces. While several hypotheses have been proposed for the evolution and maintenance of sexual dimorphism in horseshoe crabs, many researchers believe it is primarily because larger females have the capacity to lay more eggs.[13]
Gut Microbiome
The gut microbiome of any animal is dynamic and shaped by a myriad of factors: genetics, habitat, behavior, diet, interactions with environment, and more. The microorganisms, which co-evolve with the animal, play indispensable roles in the survival and function of said animal. As such, information about the gut microbiome like the composition and relative abundance of microbes at a specific time can be utilized to track the health of an individual.[14] The gut microbiome of horseshoe crabs has been studied in several experiments using 16s rRNA sequencing. Proteobacteria, Bacteroidetes, and Firmicutes are the dominant phyla of bacteria found across all four extant species. Composition of the horseshoe crab gut microbiome has been observed to shift due to stress from pollutants. Pollution of the world’s oceans and coastal areas has been, among other things, a major concern for the existence of marine species like the horseshoe crab. A 2024 research studied the adverse effects of antibiotics and plastic nanoplastics on the tri-spine horseshoe crab Tachypleus tridentatus.[15] A pronounced rise in the relative abundance of Bacteroidetes was observed in the gut microbiome of the groups exposed to the antibiotic norfloxacin, polystyrene nanoparticles, or both. Bacteroidetes in the gut break down polysaccharides and other sugars, producing vital nutrients. A rise in their numbers may signal an overdependence on these microbes for digestion, indicating compromised health. These findings corroborated results from a study done on Tachypleus tridentatus a year prior, which also found out an increase in the numbers of Bacteroidetes in response to nanoplastics.[16]
Blue Blood
Hemolymph, the fluid found in circulation in arthropods, is equivalent to blood in vertebrates. Horseshoe crab ‘blood’ is famously blue, owing to the presence of the copper-based oxygen transport molecule called hemocyanin. Oxygenated hemocyanin is a vibrant blue color. Motile blood cells called amebocytes present in the crab hemolymph secrete coagulatory proteins in response to lipopolysaccharide endotoxins from Gram-negative bacteria and beta-1,3-glucans from the cell walls of certain fungi. The pathogen is immobilized, preventing further spread of infection.[17] This remarkable component of the crabs’ innate immunity, among others, is considered to have contributed to the crabs’ remarkably long existence. Limulus amebocyte lysate (LAL) test, based on an aqueous extract of amebocytes from the Atlantic horseshoe crab Limulus polyphemus, has been the gold standard for endotoxin detection in parenteral drugs and equipments since 1977. Tachypleus amebocyte lysate (TAL), a similar test using amebocytes derived from the Asian species Tachypleus gigas or Tachypleus tridentatus, is performed as well.[18] An important class of anti-microbial peptides called Tachyplesin was first isolated from Tachypleus tridentatus hemolymph back in 1988.[19] Since then, three variants of the peptide have been identified.[20] The peptides possess just 17 amino acid residues and contain two disulfide linkages, which confers considerable stability. Tachyplesins function by disrupting membrane stability of invading pathogens. They have been also been shown to act against highly antibiotic-resistant bacteria and even target cancer cells.[21]
Horseshoe crabs are collected and bled from their pericardium; the crabs may, at times, be depleted of half their hemolymph during the bleeding process. Concerns have been raised regarding the ethics and sustainability of this practice. While most crabs recover well from the blood loss and are promptly returned to their habitat, the stress associated with transport and being outside of water for extended periods of time can be lethal.[22]
Factor C is the pro-enzyme in the crab hemolymph that initiates the clotting cascade in response to endotoxins. Factor C can be synthesized in recombinant organisms like yeast and bacteria, which is aptly termed rFC (recombinant factor C). rFC assays have been used alternatively for endotoxin detection alongside the traditional LAL/TAL tests since 2003. rFC is highly specific in its response and can be made in high quantities which would require a large number of crabs to be bled. However, the dependence on the conventional LAL/TAL tests continues; in fact, the issue has only worsened since the onset of the COVID-19 pandemic, whereby extensive numbers of crabs were exploited to ensure safety of surgical equipment and vaccines being made.[2]
Conclusion
While horseshoe crabs no longer possess the range and diversity of former times, their continued existence and survival for nearly 500 million years invite nothing short of marvel and wonder. Yet, their evolutionary success is being constantly threatened by human actions. We must become aware as soon as possible of the indispensability of these fascinating creatures, not just to ourselves but also the greater ecosystems of our world, and adopt sustainable practices to sustain their survival.
References
- ↑ 1.0 1.1 Bicknell RD, Kimmig J, Budd GE, Legg DA, Bader KS, Haug C, Kaiser D, Laibl L, Tashman JN, Campione NE. Habitat and developmental constraints drove 330 million years of horseshoe crab evolution. Biological Journal of the Linnean Society. 2022 May 1;136(1):155-72.
- ↑ 2.0 2.1 Gauvry GA, Uhlig T, Heed K. LAL/TAL and animal-free rFC-based endotoxin tests: their characteristics and impact on the horseshoe crab populations in the United States and Asia. InInternational Horseshoe Crab Conservation and Research Efforts: 2007-2020: Conservation of Horseshoe Crabs Species Globally 2022 Jul 14 (pp. 369-390). Cham: Springer International Publishing.
- ↑ Zhou Y, Liang Y, Yan Q, Zhang L, Chen D, Ruan L, Kong Y, Shi H, Chen M, Chen J. The draft genome of horseshoe crab Tachypleus tridentatus reveals its evolutionary scenario and well-developed innate immunity. BMC genomics. 2020 Dec;21:1-5.
- ↑ Rudkin DM, Young GA, Nowlan GS. The oldest horseshoe crab: a new xiphosurid from Late Ordovician Konservat‐Lagerstätten deposits, Manitoba, Canada. Palaeontology. 2008 Jan;51(1):1-9.
- ↑ Van Roy P, Briggs DE, Gaines RR. The Fezouata fossils of Morocco; an extraordinary record of marine life in the Early Ordovician. Journal of the Geological Society. 2015 Sep;172(5):541-9.
- ↑ Obst M, Faurby S, Bussarawit S, Funch P. Molecular phylogeny of extant horseshoe crabs (Xiphosura, Limulidae) indicates Paleogene diversification of Asian species. Molecular Phylogenetics and Evolution. 2012 Jan 1;62(1):21-6.
- ↑ Lamsdell JC. Evolutionary history of the dynamic horseshoe crab. International Wader Studies. 2019;21.
- ↑ Holland PW. More genes in vertebrates?. Genome Evolution: Gene and Genome Duplications and the Origin of Novel Gene Functions. 2003:75-84.
- ↑ 9.0 9.1 Shingate P, Ravi V, Prasad A, Tay BH, Garg KM, Chattopadhyay B, Yap LM, Rheindt FE, Venkatesh B. Chromosome-level assembly of the horseshoe crab genome provides insights into its genome evolution. Nature communications. 2020 May 8;11(1):2322.
- ↑ Alonso CR. Hox proteins: sculpting body parts by activating localized cell death. Current Biology. 2002 Nov 19;12(22):R776-8.
- ↑ Nong W, Qu Z, Li Y, Barton-Owen T, Wong AY, Yip HY, Lee HT, Narayana S, Baril T, Swale T, Cao J. Horseshoe crab genomes reveal the evolution of genes and microRNAs after three rounds of whole genome duplication. Communications Biology. 2021 Jan 19;4(1):83.
- ↑ Lamsdell JC. The phylogeny and systematics of Xiphosura. PeerJ. 2020 Dec 4;8:e10431.
- ↑ Smith MD, Brockmann HJ. The evolution and maintenance of sexual size dimorphism in horseshoe crabs: an evaluation of six functional hypotheses. Animal Behaviour. 2014 Oct 1;96:127-39.
- ↑ Xiong K, Miao F, Waiho K, Liu X, Fang JK, He J, Hu M, Wang Y. Effect of Probiotics on Juvenile Tachypleus tridentatus Gut Microbiota. Journal of Ocean University of China. 2022 Jun;21(3):564-72.
- ↑ Huang M, Ma Y, Qian J, Sokolova IM, Zhang C, Waiho K, Fang JK, Ma X, Wang Y, Hu M. Combined effects of norfloxacin and polystyrene nanoparticles on the oxidative stress and gut health of the juvenile horseshoe crab Tachypleus tridentatus. Journal of Hazardous Materials. 2024 Apr 15;468:133801.
- ↑ Pan Y, Qian J, Ma X, Huang W, Fang JK, Arif I, Wang Y, Shang Y, Hu M. Response of moulting genes and gut microbiome to nano-plastics and copper in juvenile horseshoe crab Tachypleus tridentatus. Marine Environmental Research. 2023 Oct 1;191:106128.
- ↑ Isakova V, Armstrong PB. Imprisonment in a death-row cell: the fates of microbes entrapped in the Limulus blood clot. The Biological Bulletin. 2003 Oct;205(2):203-4.
- ↑ Novitsky TJ. Biomedical applications of Limulus amebocyte lysate. Biology and conservation of horseshoe crabs. 2009:315-29.
- ↑ Nakamura T, Furunaka HT, Miyata T, Tokunaga F, Muta T, Iwanaga S, Niwa M, Takao T, Shimonishi Y. Tachyplesin, a class of antimicrobial peptide from the hemocytes of the horseshoe crab (Tachypleus tridentatus). Isolation and chemical structure. Journal of Biological Chemistry. 1988 Nov 15;263(32):16709-13.
- ↑ Vernen F, Harvey PJ, Dias SA, Veiga AS, Huang YH, Craik DJ, Lawrence N, Troeira Henriques S. Characterization of tachyplesin peptides and their cyclized analogues to improve antimicrobial and anticancer properties. International Journal of Molecular Sciences. 2019 Aug 26;20(17):4184.
- ↑ Chen Y, Xu X, Hong S, Chen J, Liu N, Underhill CB, Creswell K, Zhang L. RGD-Tachyplesin inhibits tumor growth. Cancer research. 2001 Mar 3;61(6):2434-8.
- ↑ Krisfalusi-Gannon J, Ali W, Dellinger K, Robertson L, Brady TE, Goddard MK, Tinker-Kulberg R, Kepley CL, Dellinger AL. The role of horseshoe crabs in the biomedical industry and recent trends impacting species sustainability. Frontiers in Marine Science. 2018 Jun 5;5:185.
Edited by Ifti Rahman, student of Joan Slonczewski for BIOL 116, 2024, Kenyon College.