Flooded Soils
Introduction
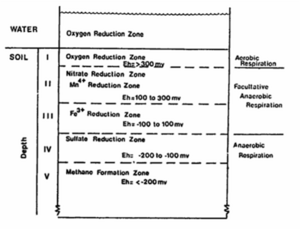
Flooded soils occur with complete water saturation of soil pores, and generally result in anoxic conditions of the soil environment. Flooded soil environments may include such ecosystems as: rice paddies; wetlands (swamps, marshes, and bogs); compacted soils; and post-rain soils (Scow, 2008). Additionally, similar redox conditions (where oxygen is lacking) can also be found within soil aggregates and along pollutant plumes, and thus many of the concepts discussed in this section may be applied to those environments.
Oxygen is only sparingly soluble in water and diffuses much more slowly through water than through air (Schlesinger, 1997). What little oxygen that is present in saturated soils in the form of dissolved O2 is quickly consumed through metabolic processes. Oxygen is used as terminal electron acceptor via respiration by roots, soil microbes, and soil organisms (Sylvia, 2005), and is lost from the soil system in the form of carbon dioxide (CO2). Heterotrophic respiration may completely deplete oxygen in flooded soils; and these effects may be observed within only a few millimeters of the soil surface (Schlesinger, 1997).
Due to the deficiency of oxygen in flooded soils, those organisms inhabiting flooded soils must be able to survive with little to no oxygen. Although energy yields are much greater with oxygen than with any other terminal electron acceptor (see electron tower theory, section 2.1.1), under anoxic conditions anaerobic and facultative microbes can use alternative electron acceptors such as nitrate, ferric iron (Fe III), manganese (IV) oxide, sulfate, and carbon dioxide to produce energy and build biomass.
Microbial transformations of elements in anaerobic soils play a large role in biogeochemical cycling of nutrients and in greenhouse gas emissions. Changes in the oxidation state of terminal electron acceptors may result in nutrient loss from the system via volatilization or leaching. Anaerobic microbial processes including denitrification, methanogenesis, and methanotrophy are responsible for releasing greenhouse gases (N2O, CH4, CO2) into the atmosphere (Schlesinger, 1997).
Processes
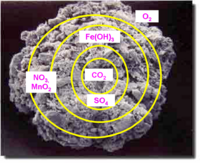

In general, flooded soil condition occurs due to seasonal flooding or agricultural activity. The flooded soils condition can be often converted into non-flooded soil condition by the water level fluctuation and drainage. Through this variation of soil condition, various gases are emitted into the atmosphere or environmental factors, such as redox potential (Eh), pH, acidity, alkalinity, and salinity, are continuously changed. As explained in the introduction, microorganism can use alternative terminal electron acceptor when dissolved oxygen is absent. They successively use electron acceptor according to the order of electron acceptor utilization based on electron tower. The order change of electron acceptor utilization is observed in soil aggregates and pollutant plume.
Oxidation/reduction (redox) reaction
Electron tower
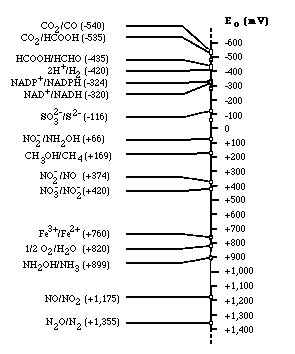
Electron tower theory explains the utilization order of electron acceptor for respiration. Depending on the type of electron acceptors used by microorganisms, microbes can be classified into the strict aerobes, obligate anaerobes, and facultative anaerobes. The strict aerobes can not live under anoxic condition; on the contrary, obligate anaerobes can never use oxygen as electron acceptor. However, facultative anaerobes can live in both aerobic and anaerobic condition. If oxygen is plentiful, they tend to use oxygen because microorganisms gain much energy from reducing oxygen rather than other electron acceptors. When there is no more available oxygen in solution, they start to use nitrate as electron acceptor. Thus, obligate anaerobes and facultative anaerobes use alternative electron acceptor in the order of electron acceptor having more reducing energy. Oxygen is most efficient electron acceptor, while carbon dioxide has the less reducing energy.
Recover from flooded soils
Variation of pH and Eh
pH
Neutral pH soil
When soil is saturated with water, pH drops at first due to organic acid produced from fermentation. Then, pH gradually starts to rise because H+ is consumed via respiration of the aerobes and anaerobes. The half reactions of hydrogen consumption are as follow;
Aerobic respiration: ½ O2 + 2e- + 2H+ -> H2O (by facultative anaerobe and aerobes)
Iron reduction: Fe(OH)3 + 3 H+ + 2e- -> Fe2+ + 2H2O (by Iron reducing bacteria)
Denitrification: 2NO3- + 12 H+ +10e- -> N2+6H2O (by Denitrifier)
Sulfate reduction: SO42- + 10H+ +8e- -> H2S + 4H2O (by sulfate reducing bacteria)
Methane production: CO2 + 8 H+ + 8e- -> CH4 +2 H2O (by Methanogeous)
Manganese reduction: MnO2 + 4H+ + 2e- ->Mn2+ + 2H2O (by Manganese reducing bacteria)
Eh
During the succetion of anaerobic oxidation processes, the redox potential (Eh) of the flooded soil will decrease as a result of the reduced products formed. The approximate redox potential values that indicate the start and end of a specific reduction oxidation process are as follows: (How to make a table??)
Observation Eh (mV)
Disappearance of oxygen +330
Disappearance of nitrate +220
Appearance of manganese ions +200
Appearance of ferrous ions +120
Disappearance of sulfate -150
Appearance of methane -250
Solubility/mobility of mineral
Since the toxicity, solubility, mobility,and bioavailability of a given element or compounds are mainly influenced by soil solution redox potenial and pH, flooded soil condition plays an important role in mobility of trace metal, nutrients, and mineral.
Plant nutrient availability
Flooded soils can prevent efficient gas exchange between the plant root and the soil. pH plays a main role in a healthy plant growth process. In flooded soils, under anaerobic conditions the pH value wil tend to rise. Denitrification of soil nitrate to nitrogen gas plays a major role in the rise of pH levels. Flooding results in poor soil aeration because the supply of oxygen to flooded soil is severely limited. Oxygen deficiency is likely the most important environmental factor that triggers growth inhibition and injury in flooded plants. Microoganisms will begin to use the available plants nutrients as alternative electron acceptors, such as sulfate, nitrate and iron(III). Experiments have been done on soybean plants to show the effects of flooded soils. Flood duration effects on the soybean plant were manifested in yellowing and abscission of leaves at the lower nodes, stunting, and reduced dry weight and seed yield. Canopy height and dry weight decreased linearly with duration of the flood at both growth stages. The growth rates were 25 to 35% less when soybean was flooded (3)
What over watering looks like in a common house plant
Key microbial processes and organisms involved
The role of microorganisms under flooded soil
Microbial processes
Microorganism activity
In anaerobic respiration oxygen is replaced by other compounds as TEA (terminal electron acceptors). Some important terminal electron acceptors include iron, nitrate, sulfate, and manganese. These processes occur because of microoganism activity. Energy yields of these alternative electron acceptors are lower than for aerobic respiration. In flooded anaerobic conditions, microorganisms will have to use other lower energy yielding compounds besides oxygen. As the available oxygen drops organisms that thrive under anoxic conditions begin to grow using alternative electron acceptors. The order in which these available elctron acceptors is consumed can be partially predicted by the electron tower. Changes in the oxidation-reduction status over time reflect the activities of a succession of microorganisms able to use these alternative electron acceptors. Flooding alters the microbial flora in soil by decreasing the O2 concentration. Fermentation is one of the major biochemical processes responsible for organic matter decomposition in flooded soils. Eh levels can affect the compounds that are fermented. These levels will tend to gradually drop in flooded soils
fermentation under anoxic condition
Organisms involved in flooded soil
nitrate reducing bacteria
Denitrification is carried out by obligate respiratory bacteria belonging to the genra Agrobactterium, Alcaligenes, Bacillus, Paracoccus, Pesudomonas and Thiobacillus (Knowles, 1982). Nitrate ammonification found in facultative anaerobe bacteria belonging to the genera Bacillus, Citrobacter and Aeromonas, or in the memebers of the Enterobacteriaceae (Cole adn Brown, 1980; Smith adn Zimmerman, 1981; MacFarlane and Herbert, 1982). Strictly anaerobic bacteria belonging to the genus Clostridium are also able to reduce nitrate to ammonia (Hasan and Hall, 1975). Pure culture studies show evidance that nitrate reduction does occure in presence of oxygen (Kuenen and and Robertson, 1987).
Iron reducing bacteria
Ferrous iron is used as electron acceptor by iron-reducing bacteria such as G. metallireducens, G. sulfurreducens, and Shewanella putrefaciens.Different forms of ferric oxides exist in aerobic drained as well as in waterlogged soils. Not all of these ferric oxides are equally suitable for reduction by ferric oxide reducer bacteria (Gotoh and Patrick, 1974; Schwertmann and Taylor, 1977). In general, amorphous forms are more efficient for ferric reducer bacteria than crystalline forms (Lovely adn Phillips, 1986). The reduction of ferric oxide may release phosphate and trace elements that are adsorbed to the amorphous ferric oxide and thus enhance availablity of these compounds in the soil (Lovely and Phillips, 1986).
Manganese reducing bacteria
Sulfate reducing bacteria
Bacteria can use acetate as electron donor and sulfate as electron acceptor. This reaction is as follow;
CH3COO- + SO42- + 3 H+ ---> 2CO2 + H2S + 2 H2O
This reaction is carried out by sulfate-reducing bacteria such as Desulfobacterales, Desulfovibrionales, and Syntrophobacterales (Langston and Bebiano 1998). Hydrogen sulfide gas produced via anaerobic respiration cause the rotten egg odor.
Methanogen bacteria
Methanogen (e.g Methanobacterium formicum, Methanobacterium bryantii, Methanobacterium thermo-autrotrophicum, and etc ) can use CO2 and produce methane (Langston and Bebiano 1998)
Greenhouse Gas Emissions from Flooded Soils
Flooded soils are dynamic ecosystems that play an important role in biogeochemical cycling and in the production of greenhouse gases. Methane (CH4) and nitrous oxide (N2O) are produced as byproducts of anaerobic metabolism in the low-redox zones characteristic of flooded soils, where oxygen is lacking. Carbon dioxide (CO2), which receives widespread attention as a greenhouse gas and potential source of global warming, may also be produced at the interface of anaerobic-aerobic zones through the consumption of methane gas. However, it should be noted that from a global standpoint methane and nitrous oxide on a per molecule basis have the potential to contribute 25x and 300x more to global warming over the next century than carbon dioxide, respectively (Schlesinger, 1997). Thus the conversion of methane gas to carbon dioxide essentially reduces the greenhouse gas effect by 25x per molecule per 100 years. According to Matthews and Fung (1987), an estimated 3.6% of terrestrial land is classified as wetlands, and although this number continues to decline (Schlesinger, 1997) the effect of flooded soils to the global climate is clear.
Methane production; methanogenesis
Methane production occurs exclusively in anaerobic conditions by a group of Archaea known as methanogens. These microbes are obligatory, and require extremely low redox conditions in the range of -100mV (see electron tower theory, section 2.1.1) (Sylvia, 2005). If oxygen is introduced into the system, methanogenesis ceases; thus, the process of methanogenesis depends on saturated soil conditions.
Methanogenesis can occur via one of two pathways: either by 1) CO2 reduction or by 2) acetate fermentation.
1) CO2 + H2 --> CH4 (CO2 reduction)
and
2) CH3COOH --> CH4 + CO2 (acetate fermentation)
Both acetate and hydrogen are byproducts of anaerobic fermentation.
Because the process of methanogenesis is “fed” byproducts produced from a complex series of degradation processes which are themselves “fed” complex organic matter, rates of methane production are highly sensitive to changes in temperature. Methanogenesis has a Q10 value in the range of 30-40, which is substantially higher than most biochemical process (Sylvia, 2005).
Despite the clear effect of increasing temperatures on the rate of methanogenesis, the actual impact of global warming on methane production rates in wetlands and permafrost regions is highly unpredictable. Because methanogenesis requires anoxic conditions, any drying of flooded soil environments would both decrease methane production and increase methane oxidation, reducing overall methane emissions. Alternatively, warmer climates could increase growing seasons, which would increase methane emissions (Sylvia, 2005).
CO2 production via methane consumption: Methanotrophy
Some of the methane produced via methanogenesis in flooded soils may be consumed and oxidized to CO2 at the interface of the anaerobic-aerobic zones. This process occurs primarily by a group of bacteria known as methanotrophs. These microbes can be found in surface layers of wetland soils and unsaturated upland soils, and may be exposed to very high concentrations of methane gas, sometimes amounting to 10% or more of the dissolved gases. Methane is thought to be the only source of C and energy for these bacteria.
Methanotrophy occurs in the following reaction:
CH4 + 2O2 --> CO2 + 2H2O
Methane is similar in size and shape to ammonium; and there is some evidence that nitrifiers (ammonium oxidizers) can also oxidize methane (Sylvia, 1998). Because they are molecularly similar, NH4+ competes at the enzyme’s active site, inhibiting methane oxidation. As a result, methanotrophy is generally inhibited by the addition of fertilizer or excess nitrogen in the system, when ammonium levels are high.
Alternatively, if nitrogen is extremely limiting the addition of nitrogen will stimulate methanotrophy and actually increase methane consumption. So although it is generally expected that adding N-fertilizer will decrease CH4 consumption and lead to increased global warming potential, sometime the opposite effect may occur. (Sylvia, 2005).
Nitrous oxide; denitrification
Denitrification is an anaerobic process in which nitrate serves as the terminal electron acceptor, and generally some source of organic carbon is the electron donor (also H2 may serve as a donor).
In this process, nitrate is oxidized to nitric oxide, then nitrous oxide, and then fully oxidized to dinitrogen:
NO3- --> NO --> N2O --> N2
However, under certain conditions the full oxidation of NO3- to N2 does not occur and nitrous oxide (N2O) is produced.
Microbes responsible include both organotrophs and lithotrophs, and this process occurs primarily by facultative anaerobes.
Although a low redox potential is important for denitrification to occur (oxygen must not be present or it will “out-compete” nitrate as a terminal electron acceptor), redox requirements are not so low that this process cannot occur within anaerobic microsites of soil aggregates.
Factors affecting nitrous oxide production include oxygen, pH, and the ratio of nitrate to available C. Although denitrification rates decrease with increasing oxygen, the proportion of N evolved as nitrous oxide actually increases with increasing oxygen. Low pH generally inhibits the reduction of N2O to N2; thus at low pH, N2O will likely dominate. However, highly acidic soils have low N availability and low nitrification and denitrification rates. Thus, the highest rate of nitrous oxide production from denitrification occurs in moist soils that cycle N rapidly (Sylvia, 2005).
References
(1) Lecture 5 of Kate Scow. 2008. Microbial Metabolism. Unpublished, University of California, Davis.
(2) Silvia, D.M., et al. 2005. Principles and Applications of Soil Microbiology. 2nd ed. Pearson Prentice Hall, New Jersey.
(3) Flood Duration Effects on Soybean Growth and Yield http://agron.scijournals.org/cgi/content/abstract/81/4/631
(4) Schlesinger, W.H. 1997. Biogeochemistry: An Analysis of Global Change. 2nd ed. Elsevier Academic Press, Amsterdam.
(5) Matthews, E. and I. Fung. 1987. Methane Emission from Natural Wetlands: Global Distribution, Area, and Environmental Characteristics of Sources. Global Biogeochemical Cycles 1: 61-86.
Edited by students of Kate Scow