Genetics of Amyotrophic Lateral Sclerosis
Introduction
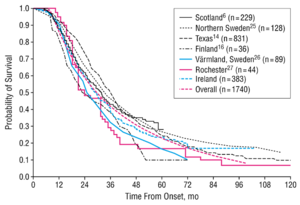
Amyopathic lateral sclerosis (ALS) is an age-related progressive neurodegenerative disorder of both upper motor neurons (UMN) and lower motor neurons (LMN).[1] ALS is also referred to as Motor Neuron Disease (MND) in European countries such as the United Kingdom the disease affects motor neuron functions in the human motor system.[2] ALS gradually degrades neurons that are responsible for controlling voluntary muscle movements. The degenerations cause the links between the brain, spinal and voluntary muscles to degrade leading to the loss of control of motor functions, eventually leading to paralysis. The muscles continue to weaken until fasciculation occurs and eventually atrophy of the voluntary muscles.[3] There is no effective treatment that cures the disease or reverses the progression of the degeneration. Non-invasive ventilation, alongside physical and speech therapy, can prolong the life of patients and improve the quality of life. Most patients die from respiratory failure, within 3 to 5 years after initial symptoms (Figure 1).[3]
There are two types of ALS: sporadic ALS and familial ALS. About 90% to 95% cases of ALS are sporadic, however, the causes of sporadic ALS are still unknown.[3][4] There are no known risk factors associated with sporadic ALS and patients have no family history with the disease. The remaining 5% to 10% of cases are familial.[4] Familial ALS only requires one parent to carry the gene responsible for the disease as the gene is autosomal dominant. Mutations in 25 genes have been associated with the cause of ALS.[5] 25% to 40% of familial cases are caused by the defect in a gene known as “chromosome 9 open reading frame 72” (C9OFR72). Some patients carrying C9OFR72 may show symptoms of both ALS and dementia. 12% to 40% of familial cases are as a result of a defect in the gene that codes instructions for the production for the enzyme copper-zinc superoxide dismutase 1 (SOD1).[3] Researchers have associated various environmental factors with ALS, however, there is still uncertainty which, if any, factors are linked to the causal factors of ALS. Researchers suggest that even certain infections in childhood can onset ALS in adulthood.[2]
Section 1: Genetics of ALS
There are 25 genes known to be associated with familial ALS yet there is still great ambiguity of precise roles each of the genes in the development of ALS. [6] There are three main genes that have been studied: C9OFR72, SOD1 and TARDBP.
SOD1
The SOD1 gene encodes for the enzyme Copper-zinc Superoxide Dismutase 1 (SOD1) enzyme. SOD1 is an antioxidant that protects cells from damage caused by superoxide, a toxic-free radical produced in the mitochondria, by binding to copper 2+ ions and regulating copper homeostasis in cells.[7] There are over 180 different mutations identified in SOD1 gene.[7][8] The mutations in the SOD1 gene are primarily single base substitutions and nonsense mutations, the insertion of a base that leads to a premature stop codon.[8] Mutations in the SOD1 gene can lead to either a gain or loss in function. SOD1 mutant proteins onset ALS through the gain in toxicity, causing cell degeneration, but the physiological function still remains. The mechanism to the gain in toxicity remains unknown.[6] Overexpression of SOD1 mutant proteins leads to abnormal accumulation of copper 2+ ions in the spinal cord due to the enzyme becoming inactive and losing function.[7] A specific prognosis is difficult to give based on SOD1 mutations as there are many other factors that can onset ALS and mutations cannot predict the severity of ALS.[8] A change on the SOD1 gene sequence can lead to clinical symptoms of ALS whereas, complete deletion of the gene does not produce symptoms of the disease.[7]
C9OFR72
Up to 40% of familial cases of ALS are caused by mutations in C9OFR72.[3][9] Mutations in C9OFR72 gene leads to the inhibition of the production of a protein involved in trafficking of autophagosomes during autophagy. Autophagy is a process that allows for degenerated components of cells to be removed and recycled.[10]
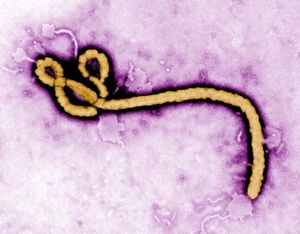
Researchers looking at family histories of patients with ALS found mutations were linked to chromosome 9 and found mutations to be pathogenic. A hexanucleotide repeat expansion (GGGGCC) in the non-coding region of the C9OFR72 gene with hundreds and thousands of repeats is seen in commonly familial ALS cases and in some sporadic ALS cases. The precise length of the expansion is still unknown.[9] The expanded repeats within the C9OFR72 gene causes a variety of downstream effects on RNA processing. RNA transcripts produced by the mutant gene form discrete nuclear structures. These structures can cause a rise in toxicity by interacting with the RNA binding proteins, impairing the protein's function causing RNA expression to be altered.[11]
TARDBP
TARDBP gene encodes for TAR DNA-binding protein 43 (TDP – 43), a DNA/RNA binding protein regulating several processes in RNA expression. There are over 48 mutations in TARDBP that have been associated with ALS.[11] The mutations in the TARDBP gene are key mediators in the development of ALS, they lead to protein inclusions and TDP – 43 inclusions are a common pathological feature of nearly all patients on sporadic and familial ALS.[12] Both loss and overexpression of the TARDBP gene can cause ALS, the protein must be strictly regulated. The overexpression of the TARDBP gene leads to fragmentation of the protein, producing fragments that have been observed in ALS patients.[11] Mutations are not a passive marker of neuronal death but play a crucial role in the ALS pathway (Figure 2). The mechanism behind mutations in TDP – 43 protein causing the neurodegenerative diseases is still unknown.[12]
Other genes that have been associated with ALS include: FUS, ANG, TBK1 and OPTN.[11]
Section 2: Neuroepigenetics of ALS
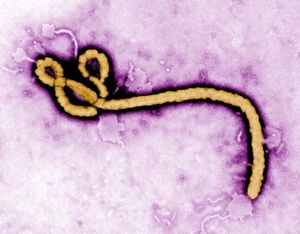
DNA modification at different transcriptional sites has been associated with ALS. DNA methylation is used in gene silencing catalysed by DNA Methyltransferase (DNMT). Methylated DNA changes the chromatin structure, inhibiting transcription factors to bind to a particular section of DNA and inhibiting transcription of genes in this region.[13] The overexpression of DNMT, in particular DNMT3A (methylating agent), leads to degeneration and apoptosis of motor neuron cells and induces DNA damage.[13][14] DNMT3A is present in the central nervous system of patients with ALS.[13] A key site regulated by epigenetic mechanisms is in the hexanucleotide expansion repeat in C9OFR72, which has been linked to ALS. The overexpression of DNMT leads to hypermethylation of the cytosine–phosphate–guanine (CpG) dinucleotide islands on C9OFR72. Hypermethylation causes downregulation of the C9OFR72 gene expression reducing transcription and leads to apoptosis of motor neuron cells in sporadic ALS (Figure 3).[13][14] Higher degrees of methylation correlates to short disease duration, indicating increased severity of ALS.[14] Chromatin modification is linked to many key genes involved in ALS; however, specific genes associated with DNA methylation that cause the deaths of motor neuron cells have not been identified yet. Recent studies suggest that epigenetic mechanisms, such as DNA methylation, could be relevant to the therapeutic treatment of ALS.[13]
Section 3: Gut Microbiome
Researchers have found links between the human gut microbiome and ALS pathogenesis.[15][16] In a recent study, researchers found 11 distinct bacteria associated with ALS, suggesting that the gut microbiome could influence the severity of ALS.[15] The vast majority of human gut intestinal bacteria were from the Firmicutes and Bacteroidetes phyla.[17]
Researchers observed degeneration of the gut in ALS patients. The loss of the gut’s structural integrity, through increased permeability and damage tight function barrier, reducing function. The structural and physiological changes coincide with the population shift of the gut microbiome.[18] Recent studies show the effects of different species of bacteria in the gut in the development of ALS. For example, an increase in Akkermansia muciniphila improves the symptoms of ALS whereas an increase in Ruminococcus torques and Parabacteroides distasonis exacerbate symptoms of the neurological disease.[15] A structural change that occurs in the gut is increased permeability of the lining and along with a shifted microbiome profile, the change decreases levels of butyrate-producing bacteria. Butyrate relieves symptoms of ALS and lengths both survival time and the onset of weight loss.[18] The decrease in symptoms were caused by the reduction of neuroinflammation.[17][18] Altered gut microbiome and metabolite products in ALS provide a deep insight into the development of ALS, with the potential of establishing therapeutic treatments.[17] Researchers suggest the interactions between the gut microbiome and brain have an important influence on ALS.[16]
The gut microbiome-brain connection is seen in other neurodegenerative diseases, such as Alzheimer’s and frontotemporal dementia, emphasising the importance of the link.[15] The mechanism of microbiota–gut-brain axis is still not fully known; however, a deeper understanding could lead to the development of microbial treatments to relieve symptoms in patients with ALS.[17]
Conclusion
In the last 10 years, our understanding of the causes behind ALS has drastically increased. Despite the progress, the exact cause of ALS is still unknown. Researchers have found a variety of possible causal factors for familial and sporadic ALS: from genes, such as SOD1 and TARDBP; neuroepigenetics, including methylation of C9OFR72, and associations with the human gut microbiome. The improved knowledge of genetic mutations of familial ALS will aid the understanding of the interactions genetics has with the environment such as with the variety of bacteria within the gut microbiome. Despite the increased understanding of familial ALS, there is still much unknown in regard to causal factors of sporadic ALS. The advancing discovery of new information associated with causation of the disease will provide opportunities for developing therapeutic treatment for patients with ALS.
At right is a sample image insertion. It works for any image uploaded anywhere to MicrobeWiki. The insertion code consists of:
Double brackets: [[
Filename: PHIL_1181_lores.jpg
Thumbnail status: |thumb|
Pixel size: |300px|
Placement on page: |right|
Legend/credit: Electron micrograph of the Ebola Zaire virus. This was the first photo ever taken of the virus, on 10/13/1976. By Dr. F.A. Murphy, now at U.C. Davis, then at the CDC.
Closed double brackets: ]]
References
- ↑ Bryan J. Traynor, M. (2000, August 01). Clinical Features of Amyotrophic Lateral Sclerosis According to the El Escorial and Airlie House Diagnostic Criteria. Retrieved December 08, 2020, from https://jamanetwork.com/journals/jamaneurology/article-abstract/777262
- ↑ 2.0 2.1 Fang, F., Kamel, F., Sandler, D., Sparén, P., & Ye, W. (2008, March 25). Maternal Age, Exposure to Siblings, and Risk of Amyotrophic Lateral Sclerosis. Retrieved December 08, 2020, from https://academic.oup.com/aje/article/167/11/1281/131930
- ↑ 3.0 3.1 3.2 3.3 3.4 Amyotrophic Lateral Sclerosis (ALS) Fact Sheet. (n.d.). Retrieved December 08, 2020, from https://www.ninds.nih.gov/Disorders/Patient-Caregiver-Education/Fact-Sheets/Amyotrophic-Lateral-Sclerosis-ALS-Fact-Sheet
- ↑ 4.0 4.1 Chiò A;Traynor BJ;Lombardo F;Fimognari M;Calvo A;Ghiglione P;Mutani R;Restagno G;. (2008). Prevalence of SOD1 mutations in the Italian ALS population. Retrieved December 08, 2020, from https://pubmed.ncbi.nlm.nih.gov/18268245/
- ↑ Hguyen et al. (2018, June 1). ALS Genes in the Genomic Era and their Implications for FTD. Retrieved December 08, 2020, from https://doi.org/10.1016/j.tig.2018.03.001
- ↑ 6.0 6.1 Vargas, M., Pehar, M., Díaz‐Amarilla, P., Beckman, J., & Barbeito, L. (2008, August 05). Transcriptional profile of primary astrocytes expressing ALS‐linked mutant SOD1. Retrieved December 08, 2020, from https://onlinelibrary.wiley.com/doi/full/10.1002/jnr.21797?casa_token=3PfmWq1lJ8kAAAAA%3AkgsLfKO2YCfmIr-GTnSalyJXaK8QyReOHJSB2GXrGcfeQAYysuLReG9LWCNDgQDdqIjTjRECAm1p
- ↑ 7.0 7.1 7.2 7.3 Tokuda, E., & Furukawa, Y. (2016, April 28). Copper Homeostasis as a Therapeutic Target in Amyotrophic Lateral Sclerosis with SOD1 Mutations. Retrieved December 08, 2020, from https://www.ncbi.nlm.nih.gov/pubmed/27136532
- ↑ 8.0 8.1 8.2 Orrell, R., Habgood, J., Malaspina, A., Mitchell, J., Greenwood, J., Lane, R., & DeBelleroche, J. (1999, December 20). Clinical characteristics of SOD1 gene mutations in UK families with ALS. Retrieved December 08, 2020, from https://www.sciencedirect.com/science/article/abs/pii/S0022510X99002166
- ↑ 9.0 9.1 C. Amador-Ortiz, W., T. Arai, M., AL. Boxer, I., H. Braak, E., NJ. Brandmeir, F., M. Broe, J., . . . H. Zhang, C. (1970, January 01). Clinical and neuropathologic heterogeneity of c9FTD/ALS associated with hexanucleotide repeat expansion in C9ORF72. Retrieved December 08, 2020, from https://link.springer.com/10.1007/s00401-011-0907-y
- ↑ I;, W. (2016). Common Molecular Pathways in Amyotrophic Lateral Sclerosis and Frontotemporal Dementia. Retrieved December 08, 2020, from https://pubmed.ncbi.nlm.nih.gov/27498188/
- ↑ 11.0 11.1 11.2 11.3 Mejzini, R., Flynn, L., Pitout, I., Fletcher, S., Wilton, S., & Akkari, P. (2019, November 22). ALS Genetics, Mechanisms, and Therapeutics: Where Are We Now? Retrieved December 08, 2020, from https://www.frontiersin.org/articles/10.3389/fnins.2019.01310/full
- ↑ 12.0 12.1 Author links open overlay panel Sarah Y.Ebstein13IlonaYagudayeva13Neil A.Shneider124, Y.Ebstein13, S., 1, 3, IlonaYagudayeva13, A.Shneider124, N., . . . TARDBP, S. (2019, January 08). Mutant TDP-43 Causes Early-Stage Dose-Dependent Motor Neuron Degeneration in a TARDBP Knockin Mouse Model of ALS. Retrieved December 08, 2020, from https://reader.elsevier.com/reader/sd/pii/S2211124718319752?token=6AC3F22A0137F51071E0025C9A03C215EF77B6FEC0FEED74657D2B46D4B59C121D4640EA9352BFC36738822918D4B342
- ↑ 13.0 13.1 13.2 13.3 13.4 Chestnut, B., Chang, Q., Price, A., Lesuisse, C., Wong, M., & Martin, L. (2011, November 16). Epigenetic regulation of motor neuron cell death through DNA methylation. Retrieved December 08, 2020, from https://www.ncbi.nlm.nih.gov/pmc/articles/PMC3238138/
- ↑ 14.0 14.1 14.2 Xi, Z., Zinman, L., Moreno, D., Schymick, J., Liang, Y., Sato, C., . . . Rogaeva, E. (2013, June 6). Hypermethylation of the CpG island near the G4C2 repeat in ALS with a C9orf72 expansion. Retrieved December 08, 2020, from https://www.ncbi.nlm.nih.gov/pubmed/23731538
- ↑ 15.0 15.1 15.2 15.3 Blacher, E., Bashiardes, S., Shapiro, H., Rothschild, D., Mor, U., Dori-Bachash, M., . . . Elinav, E. (2019, July 22). Potential roles of gut microbiome and metabolites in modulating ALS in mice. Retrieved December 08, 2020, from https://www.nature.com/articles/s41586-019-1443-5
- ↑ 16.0 16.1 Lau, J. (2020, May 14). Harvard scientists identify gut-brain connection in ALS. Retrieved December 08, 2020, from https://news.harvard.edu/gazette/story/2020/05/harvard-scientists-identify-gut-brain-connection-in-als/
- ↑ 17.0 17.1 17.2 17.3 Wu, S., Yi, J., Zhang, Y., Zhou, J., & Sun, J. (2015, April 06). Leaky intestine and impaired microbiome in an amyotrophic lateral sclerosis mouse model. Retrieved December 08, 2020, from https://physoc.onlinelibrary.wiley.com/doi/full/10.14814/phy2.12356
- ↑ 18.0 18.1 18.2 Zeng, Q., Shen, J., Chen, K., Zhou, J., Liao, Q., Lu, K., . . . Bi, F. (2020, August 03). The alteration of gut microbiome and metabolism in amyotrophic lateral sclerosis patients. Retrieved December 08, 2020, from https://www.nature.com/articles/s41598-020-69845-8
Edited by Smrami Patel, student of Joan Slonczewski for BIOL 116 Information in Living Systems, 2020, Kenyon College.