Prochlorococcus and Climate Change
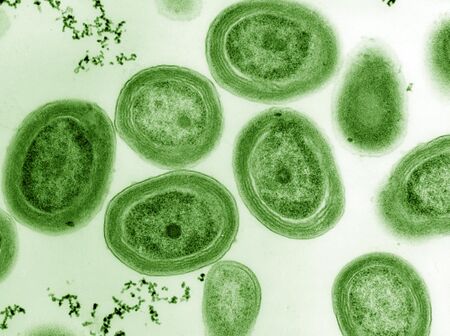
By Zachary Aronson-Paxton
What are Prochlorococcus?
Prochlorococcus is a gram negative, coccus bacteria species in the genus cyanobacteria. It is an aquatic autotroph, found throughout the planet's oceans.[1] Earth’s oxygen-rich atmosphere is the result of Cyanobacteria and their extensive amounts of photosynthesis. Specifically, Prochlorococcus is one of the most abundant autotrophic bacteria in the planet’s oceans.[1] Prochlorococcus can number up to 70,000 cells in a milliliter of ocean water.[2] These vast quantities produce a significant percentage of Earth’s entire photosynthetic output and up to 79 percent of the North Atlantic Ocean’s entire primary production of organic chemical energy.[1] [3] As a result of their large photosynthetic potential, they are a key organism in the fight against climate change and the sequestration of atmospheric carbon. Prochlorococcus uptake more carbon dioxide to undergo photosynthesis than they use up in respiration, creating a stock of carbon inside each organism.[3] When Prochlorococcus dies, they sink to the bottom of the ocean and is buried, eventually forming oil in what is known as the ocean biological pump, a transfer of organic carbon in the hydrosphere to the geosphere.[4] In this way, Prochlorococcus serve as a sink for atmospheric carbon and have a positive impact on climate change.
The Effect of Climate Change on Prochlorococcus
How does climate change influence the number and efficacy of Prochlorococcus microbes throughout the planet's oceans?
Anthropogenic change is affecting the planet in many ways, most of them unanticipated by scientists. Since Prochlorococcus is one of the most abundant sinks of atmospheric carbon dioxide, modeling how it is affected by planetary changes can predict unanticipated environmental changes. A decrease in Prochlorococcus and other autotrophic microbes could result in a larger than expected build-up of greenhouse gasses as a result of these photosynthetic organisms’ carbon sequestration potential. Greenhouse gas build-up, the main cause of global warming, is an essential factor in ecological dynamics across the planet. While Prochlorococcus has evolved for photosynthesis in environments with various different light availability and depths, the rate of anthropogenic change far outpaces Prochlorococcus’ evolution. Various human changes have different effects on Prochlorococcus; they are projected to both increase and decrease the productivity of Prochlorococcus in the Atlantic Ocean.
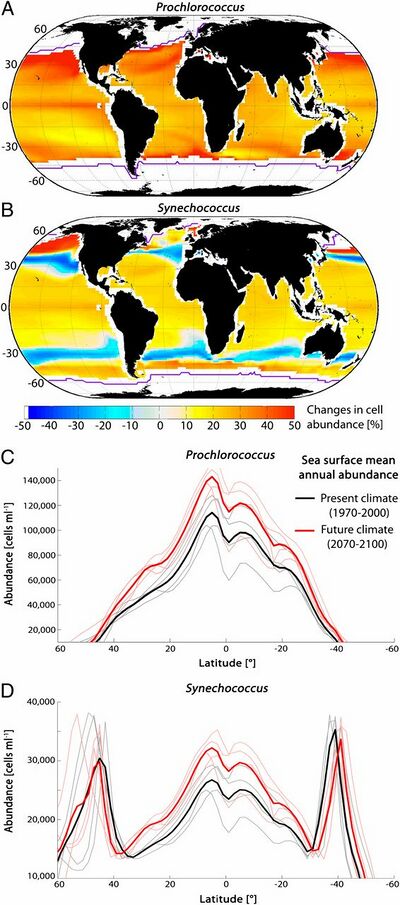
Decrease in Prochlorococcus Functionality
Rapid global change is expected to decrease some aspects of Prochlorococcus functionality in the environment. The increase of atmospheric carbon dioxide can actually increase the vulnerability of Prochlorococcus to oxygen.[5] While carbon dioxide is a reactant of photosynthesis and is commonly seen as a mechanism for increased phototrophic production, an increased carbon dioxide concentration can alter Prochlorococcus’ metabolism and microbial interactions with Alteromonas.[5] These shifts, a result of ocean acidification related to carbon dioxide levels, could eventually lead to oxidative stress. While oxygen is essential for cellular respiration, it is toxic to many cellular components due to its redox potential. Oxidative stress would lead to an overall decrease in Prochlorococcus productivity, meaning less carbon sequestration and a significant increase in global temperatures.
In addition to oxidative stress, Prochlorococcus functionality is also reduced by human produced plastic litter in the ocean.[6] Plastic bags made out of high-density polyethylene (HDPE) and other plastics made out of polyvinyl chloride (PVC) degrade to plastic leachates in the ocean.[6] The leachates, present in the marine ecosystem, directly affect the microbial communities around them. Concentration increases in plastic leachates cause decreased levels of photosynthesis in Prochlorococcus.[6] The higher the concentration of plastics, the less oxygen is produced and the less carbon dioxide is taken up by Prochlorococcus. This decreased Prochlorococcus productivity has large implications on the amount of global photosynthesis and atmospheric carbon dioxide. Exposure to plastic leachates also decreases the growth rate of Prochlorococcus.[6] Plastic pollution not only impairs photosynthesis in Prochlorococcus, but it also decreases the growth rate, leading to a smaller population size of cells and a further decrease in primary production. Anthropogenic change such as plastic pollution and high atmospheric carbon dioxide result in lower primary production levels of the most abundant aquatic phototroph.
Increase in Range and Population Size
While some aspects of anthropogenic disturbance decrease the functionality of Prochlorococcus, others are shown to increase Prochlorococcus’ range and population size. In accordance with models of projected global warming from the Intergovernmental Program on Climate Change, the Prochlorococcus population is projected to increase by over 40% in a high emission scenario, 25% in a medium emission scenario, and 20% in a low emission scenario.[7] The rising surface temperature creates more habitable space for Prochlorococcus across the Earth’s oceans.[7] However, ecological interactions are difficult to predict due to the large amount of unknown factors that could be at play.[7] Despite this relative uncertainty, a new ecological niche model designed to predict the effects of climate change on oceanic phototrophs predicted that Prochlorococcus would increase in population size and ecosystem niche dominance, confirming the increase in population size.[8] As a result, a larger population size leads to more primary production and more carbon sequestration.
Microbe Interaction
Anthropogenic change is predicted to shift Prochlorococcus’ microbial interactions in its surrounding ecosystem. Other oceanic bacteria are also affected by human change, creating a novel dynamic ecosystem. [9] Increasing temperatures and atmospheric carbon dioxide from global warming are projected to affect strains of autotrophic microbes differently.[9] While Prochlorococcus is projected to display some increased population size due to human change, Synechococcus is modeled to be even better suited to the changing climate.[9] This shift in niche dominance would alter the microbial community dynamics, potentially decreasing the prominence in Prochlorococcus in the ocean. While Prochlorococcus may be outcompeted in the future by Synechococcus, both of these bacteria are oceanic phototrophs, taking up carbon dioxide and releasing oxygen back into the atmosphere. While these interactions are projected to fit the changing environmental conditions, ecosystem modeling has a high degree of error because it must overlook many variables that could be essential to the ecosystem.
Conclusion
Widespread anthropogenic change is currently occurring across the planet today, altering the temperature, species dynamics, and ecosystems of almost all life on Earth. Prochlorococcus the oceanic autotroph that is responsible for almost all of the primary production in the planet's oceans, is also experiencing anthropogenic change.[1] This primary production of Prochlorococcus provides an essential carbon sink for the planet, potentially altering the trajectory of global climate change. Some aspects of anthropogenic change, including plastic leachate pollution (PVC and HDPE) and oxidative stress, are projected to decrease Prochlorococcus’ population size and photosynthetic potential.[6] These factors would then lead to less primary production in the oceans, less atmospheric carbon dioxide taken up by these microbes, and a compounding effect on greenhouse gasses and climate change. However, fortunately for the planet, the increased surface temperature caused by widespread global warming is actually predicted to increase Prochlorococcus’ range and population size, counteracting some of the negative effects of human change on these microbes.[7] While the increase is beneficial in the fight against climate change, ecosystem dynamics are extremely difficult to predict so this potentially large carbon sink should not be depended upon. An example of how variable ecosystem dynamics can be is seen in the projected competition between Synechococcus and Prochlorococcus. While both of these microbes have a similar impact on climate change, Synechococcus is projected to eventually outcompete Prochlorococcus as the most dominant oceanic autotroph.[9] While many changes are projected to occur in Prochlorococcus’ ecosystem, climate change is projected to have both positive and negative effects on this important microbe.
Prochlororcoccus’ Impact on the Oceans
How does Prochlorococcus’ large scale photosynthetic processes affect ocean ecosystems?
Due to Prochlorococcus’ impact on the surrounding ecosystem, large-scale effects on Prochlorococcus as a result of climate change are amplified across the entire ecosystem. Despite Prochlorococcus’ small size, it has an enormous impact on the environment around it. Since Prochlorococcus is a phototroph, it makes up the primary producer base of the food system, sequestering carbon dioxide and converting it into glucose that can be metabolized into energy by heterotrophs. The vitality of the marine ecosystem is largely dependent on autotrophs such as Prochlorococcus. Prochlorococcus impacts the surrounding ecosystem through diverse interspecific interactions, both mutualistic and predatory, as well as through its own intraspecific variation.
Interspecific Interactions
Interspecific interactions are the building blocks of ecosystems, contributing strongly to population dynamics, biodiversity, and species distribution.[10] Prochlorococcus is at the base of the trophic cascade, meaning that it is often preyed upon by oceanic heterotrophs. One such interaction occurs between Prochlorococcus and zooplankton.[11] While Prochlorococcus is often found nearer to the equator than the poles, it is predicted that climate change will expand its viable range and increase its population size.[7] However, the predatory relationship with zooplankton has been modeled to constrict the range of Prochlorococcus.[11] This community dynamic has complex consequences on the future of Prochlorococcus and the entire oceanic ecosystem. If Prochlorococcus’ range is constricted based on predation and not surface temperature, as was previously predicted, then global warming would not increase the number of Prochlorococcus and primary production would not increase in the oceans.[11]
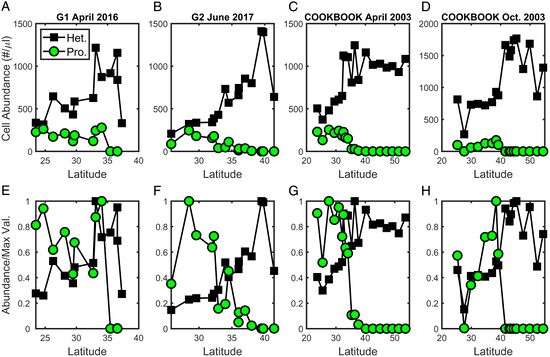
In addition, Prochlorococcus influences the ecosystem by forming mutualistic relationships with oceanic heterotrophs. While Prochlorococcus uses the carbon they sequester through photosynthesis to produce glucose and undergo respiration, Prochlorococcus sequester more carbon than they actually need.[12] Prochlorococcus have the tendency to release some of their extra carbon into their surroundings (from 9 to 24% of their total sequestered carbon), especially when exposed to nitrogen and other nutrient limited environments (Bertilsson et al. 2005).[12] Some of the excreted carbon, mainly carboxylic acids, is attractive to oceanic heterotrophs (Bertilsson et al. 2005). Prochlorococcus and the organic carbon it releases can form a community of dependent heterotrophs, increasing biodiversity and demonstrating the importance of Prochlorococcus in the oceanic ecosystems (Bertilsson et al. 2005). Prochlorococcus has a large impact on the ecosystem around it, putting it at risk for large disruptions if Prochlorococcus is dramatically affected by climate change.
Intraspecific Variation
Prochlorococcus is present throughout the planet's oceans, in many different ecosystems. As a result of its ecosystem variation, Prochlorococcus has evolved different strains suited to fill many specific niches (Biller et al. 2015). In fact, while these strains are still Prochlorococcus, they contain streamlined genomes that differ from each other (Biller et al. 2015). These strains, such as MED4, a strain adapted to high light intensities and found in the upper levels of the ocean, and MIT9313, a low light strain found deeper in the oceans, serve different ecosystem functions (Sher et al. 2011). As a result of MIT9313 being adapted to low light levels, it forms greater mutualistic relationships with heterotrophic bacteria than the high-light adapted MED4 (Sher et al. 2011). The ecological differences between Prochlorococcus strains is important for ecosystem health, especially when considering the future impacts of climate change. Prochlorococcus strains respond differently to changes in temperature, light availability, and oceanic currents (Malstrom et al. 2010). A large change in these variables, like what is predicted for climate change could result in a shift in Prochlorococcus ecotype prevalence, disturbing ecological relationships and the surrounding ecosystem.
Conclusion
Overall, Prochlorococcus is responsible for a large part of oceanic ecosystem dynamics due to their relationships with other microbes and their intraspecies variation. Prochlorococcus’ ability to photosynthesize makes them a base of the ocean’s ecosystem; they are essential in order for other ocean life to survive. While currently their ecological relationships act as a stabilizing force in the oceans, they are dependent on abiotic factors. The main abiotic factors that Prochlorococcus ecology is dependent on, temperature, light, and currents, are predicted to change dramatically through climate change. This dramatic change could potentially disrupt many of Prochlorococcus’ relationships, causing large scale change across the oceans.
Prochlororcoccus’ Impact on the Changing Planet
Does Prochlorococcus have a direct impact on the planet’s atmosphere and the process of climate change?
Anthropogenic climate change is threatening the world. The buildup of greenhouse gasses, such as carbon dioxide, in the atmosphere warms the planet, melts glaciers and ice sheets, and threatens global biodiversity (Weiskopf et al. 2020). All of these environmental changes dramatically shift ecosystem and biological interactions (Weiskopf et al. 2020). As these effects of climate change worsen, scientists struggle to develop human-induced solutions. Prochlorococcus, the most abundant oceanic phototroph, undergoes a significant amount of the planet’s primary production (Biller et al. 2015). If Prochlorococcus’ primary production could be increased further, it would become an even bigger sink of carbon dioxide and potentially ameliorate some of the effects of climate change. Due to Prochlorococcus’ mitigation potential, scientists have researched its ability to sequester carbon naturally and through human-induced techniques.
Natural Effects
Climate change is predicted to cause large scale shifts throughout the planet’s oceans. A decreased amount of available marine nutrients is projected to be one of these changes (Martiny et al. 2022). While the reduced levels of nutrients in the oceans would harm many organisms, Prochlorococcus is predicted to be relatively resistant to these low levels in this scenario (Martiny et al. 2022). While resilience would not increase the amount of carbon sequestration and lessen the effects of climate change, resilience would stabilize sequestration at an even rate (Martiny et al. 2022). The base level rates of Prochlorococcus primary production are very large. As a result, losing the carbon sink would be potentially devastating for the climate.
Human-Induced Effects
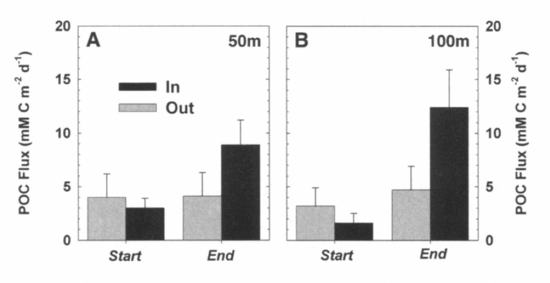
Due to the looming threat of climate change, scientists and engineers have discussed techniques to facilitate greater marine carbon sequestration. One of these techniques aims at creating a cyanobacteria bloom in the ocean, dramatically increasing the population size of Prochlorococcus and other autotrophic microbes. The population boom would be designed to absorb as much carbon as possible (Buesseler et al. 2004). This technique intentionally dumps large amounts of iron (Fe) into the oceans (Buesseler et al. 2004). Prochlorococcus and other cyanobacteria need iron in order to complete photosynthesis and the ocean has very limited natural quantities of iron (Jiang et al. 2015). While this process would increase the amount of marine primary production, it would also have massive, potentially disastrous impacts on oceanic ecosystems. Iron fertilization works in theory, yet its potential consequences outweigh the benefits. In addition to iron dumping, scientists have attempted to genetically modify Prochlorococcus and other cyanobacteria to undergo greater rates of photosynthesis (Laurenceau et al. 2020). However, despite their efforts, Prochlorococcus resisted the genetic modification (Laurenceau et al. 2020). Overall, current human ingenuity is largely ineffective at increasing the carbon sequestration potential of Prochlorococcus.
Conclusion
While there are projected to be increases in Prochlorococcus population size, the negative effects of increased atmospheric carbon dioxide on primary production leave Prochlorococcus’ potential for climate change mitigation as relatively unknown (Flombaum and Martiny 2021) (Hennon et al. 2018). However, despite the uncertainty, Prochlorococcus is a resilient bacteria that has various strains adapted to many different environments, possibly able to withstand many of the effects of climate change (Martiny et al. 2022). Unfortunately, human ingenuity (in the forms of genetic engineering and ecosystem management) has thus far not resulted in any safe techniques to increase Prochlorococcus’ carbon sequestration (Coale et al. 1998) (Laurenceau et al. 2020). Therefore, while Prochlorococcus is the most abundant phototroph in the oceans, it does not provide a solution to the planet’s growing threat of climate change.
Conclusion
Prochlorococcus bacteria is one of the most common bacteria species present in the planet’s oceans (Biller et al. 2015). As a result of its vast population size, Prochlorococcus is responsible for up to 79 percent of the North Atlantic Ocean’s primary production of chemical energy in organic molecules, and a significant portion of planetary primary production (Li 1994) (Biller et al. 2015). In addition to being one of the most widespread oceanic bacteria, Prochlorococcus interacts with many other microbes present in the ecosystem around it. From forming mutualistic relationships with microbes such as Alteromonas to being preyed upon by zooplankton, Prochlorococcus bacteria are the base phototroph in the ecosystems around them (Hennon et al. 2018) (Follett et al. 2022). However, with the introduction of anthropogenic change, many species (including Prochlorococcus) are being threatened by altered environments and interactions.
Despite Prochlorococcus’ relative resilience to some effects of climate change (such as limited nutrient availability), other environmental changes are predicted to reshape the way Prochlorococcus functions (Martiny et al. 2022). Humans releasing carbon dioxide into the environment not only warms the planet through the greenhouse effect, but also has a direct impact on Prochlorococcus. Despite carbon dioxide being a reactant for photosynthesis, its role in ocean acidification alters the metabolism of Prochlorococcus, putting it at risk for oxygen stress and decreased photosynthetic activity (Hennon et al. 2018). Plastic leachate pollution is also a direct inhibitor to Prochlorococcus’ primary production (Tetu et al. 2019). However, while some factors caused by climate change decrease Prochlorococcus’ photosynthetic activity, other factors (such as increasing global surface temperatures) are modeled to potentially expand Prochlorococcus’ range and population size (Flombaum and Martiny 2021). As a result of these conflicting effects, there is not a definitive answer as to whether Prochlorococcus will be negatively affected, positively affected, or show no effect of climate change.
Since Prochlorococcus shows both positive and negative effects in response to climate change, it can not be relied upon for consistent primary production and carbon sequestration. While a large increase in Prochlorococcus primary production would potentially ameliorate some of the effects of planetary change, there is no secure prediction that shows this result is naturally possible. Recognizing the importance of Prochlorococcus, scientists have studied ways to increase its primary production. Two main forms of these climate engineering techniques are marine iron dumping and genetic modification (Coale et al. 1998) (Laurenceau et al. 2020). Unfortunately, both techniques are either unsafe or unsuccessful in increasing Prochlorococcus primary production. Therefore, as the planet faces the threat of climate change, Prochlorococcus should not be relied upon for a large amount of carbon sequestration. Instead, solutions for increasing concentrations of atmospheric carbon dioxide should target the sources of climate change, such as limiting carbon dioxide emissions.
References
- ↑ 1.0 1.1 1.2 1.3 Biller, S., Berube, P., Lindell, D. et al. "Prochlorococcus: the structure and function of collective diversity." Nature Review Microbiology 13, 13:27
- ↑ Campbell L, Landry MR, Constantinou J, Nolla HA, Brown SL, Liu H, Caron DA. "Response of microbial community structure to environmental forcing in the Arabian Sea." Deep-Sea Res II 45: 2301–2325
- ↑ 3.0 3.1 Li WKW (1994). "Primary production of prochlorophytes, cyanobacteria, and eukaryotic ultraphytoplankton: measurements from flow cytometric sorting." Limnol Oceanogr 39: 169–175
- ↑ Resplandy, L., Lévy, M., & McGillicuddy, D. J. Jr. (2019). Effects of eddy-driven subduction on ocean biological carbon pump. Global Biogeochemical Cycles, 33, 1071– 1084
- ↑ 5.0 5.1 Hennon, G., Morris, J., Haley, S. et al.(2018) "The impact of elevated CO2 on Prochlorococcus and microbial interactions with ‘helper’ bacterium Alteromonas." ISME J 12, 520–531
- ↑ 6.0 6.1 6.2 6.3 6.4 Tetu, S.G., Sarker, I., Schrameyer, V. et al. (2019) "Plastic leachates impair growth and oxygen production in Prochlorococcus, the ocean’s most abundant photosynthetic bacteria." Commun Biol 2, 184.
- ↑ 7.0 7.1 7.2 7.3 7.4 Flombaum, P. and Martiny, A. (2021) "Diverse but uncertain responses of picophytoplankton lineages to future climate change." Limnology and Oceanography 66, 4171-4181.
- ↑ Xiao, W., Laws, E. , Xie, Y., Wang, L., Liu, X., Chen, J., Chen, B., and Huang B., (2019) “Responses of marine phytoplankton communities to environmental changes: New insights from a niche classification scheme,” Water Research, Volume 166.
- ↑ 9.0 9.1 9.2 9.3 Fu, F.-X., Warner, M.E., Zhang, Y., Feng, Y. and Hutchins, D.A. (2007). “Effects of Increased Temperature and CO2 On Photosynthesis, Growth, and Elemental Ratios in Marine Synechococcus and Prochlorococcus (Cyanobacteria).” Journal of Phycology, 43: 485-496.
- ↑ Ovaskainen, O., Skorokhodova, S., Yakovleva, M., Sukhov, A., Kutenkov, A., Kutenkova, N., Shcherbakov, A., Meyke, E., & del Mar Delgado, M. (2013). “Community-level phenological response to climate change.” Proceedings of the National Academy of Sciences of the United States of America, 110(33), 13434–13439.
- ↑ 11.0 11.1 11.2 Follett, C., Dutkiewicz, S., Ribalet, F. et al. (2022). “Trophic interactions with heterotrophic bacteria limit the range of Prochlorococcus.” Earth, Atmospheric, and Planetary Sciences, Vol. 119, No. 2.
- ↑ 12.0 12.1 Szul, M., Dearth, S., Campagna, S., Zinser, E. (2019). “Carbon Fate and Flux in Prochlorococcus under Nitrogen Limitation.” American Society for Microbiology, Vol. 4, Issue 1.
Authored for BIOL 238 Microbiology, taught by Joan Slonczewski, 2023, Kenyon College