Coral bleaching and climate change
Coral bleaching is a loss of pigment in corals that is due to the loss of the symbiotic microbe zooxanthellae, and the photosynthetic products they provide (Knowlton, 2001).
Introduction
Coral reefs and zooxanthellae
Coral falls under the phylum Cnidaria, which also includes jellyfish and sea anenome. Coral polyps form colonies strengthened by calcium carbonate skeletons. These colonies form the base of reef ecosystems. While corals are part of the animal kingdom, they are able to fix carbon dioxide from the water by photosynthesis through the microbes that live within them (Daly, et al., 2003).
Zooxanthellae are dinoflagellates that are found within the corals. The zooxanthellae and the coral have a symbiotic relationship. The corals provide the algae with excretion products from respiration such as carbon dioxide, and the algae in turn provide the coral with photosynthetic products like glucose and oxygen. However, the balance between the relationship of the two organisms is very sensitive, and therefore can be easily disrupted by environmental changes. This is because the basis of a symbiotic relationship is for both sides to maximize their benefits while at the same time minimizing their costs. If an imbalance occurs within the relationship, then one of the organisms can end it. In the case of zooxanthellae and coral, imbalances in their symbiotic relationship are caused by environmental factors such as increased water temperature or UV radiation. If the imbalance becomes too much, coral bleaching will occur (Knowlton, 2001).
Coral bleaching

Coral bleaching is the reduction of photosynthetic pigment that is due to either the death or expulsion of zooxanthellae from the coral polyps. As seen in the figure to the right, bleached corals turn white or pale yellow. After bleaching, a coral may continue to live, however growth and reproduction rates are decreased significantly. Bleaching can be due to a number of factors including increased water temperatures, increased radiation, disease, and changing sea levels (Knowlton, 2001). There are three mechanisms that are thought to account for the loss of zooxanthellae during coral bleaching. These mechanisms are: the zooxanthellae within the coral are degraded, the coral expels the zooxanthellae through exocytosis, and the coral releases endodermal cells that contain the zooxanthellae (Brown, 1997).
The survival of corals are important, because they provide the base of a major type of marine ecosystem. Specifically, corals provide food and shelter for many types of reef fish, which in turn recruit larger carnivores to the ecosystem. This makes the continuation of coral reefs very important for balancing the food chain in these ecosystems, which in turn makes coral bleaching that much more detrimental (Graham, et al., 2007).
Climate Change and Coral Bleaching
Coral bleaching events have been attributed to certain types of environmental changes. Specifically, changes in the water temperature and the light intensity are known to induce coral bleaching events. Studies have looked at these factors separately, but it is thought that coral bleaching is mostly due to a combination of the two (Brown, 1997).
Photosynthesis in zooxanthellae

Like in plants, zooxanthellae contain chloroplasts with thylakoid membranes and two photosystems. These organelles allow zooxanthellae undergo photosynthesis through light dependent and light independent reactions. The reactions take place in the chloroplast, as seen in the figure on the right. These reactions are timed by Circadian rhythms, so that the light dependent reactions of Photosystem II occur during the day, when light is available (Sorek, et al., 2013).
Heat stress on zooxanthellae
One particular study explored the effects of heat stress on the photochemistry of zooxanthellae. They wanted to determine the mechanism that damages zooxanthellae during heat stress, and ultimately causes coral bleaching. The study was conducted at One-Tree Island Research Station on the coral Stylophora pistillata. Branches of the coral were taken and placed in tanks in both natural light and UV screened light that ranged from 28 to 34°C. A second experiment was conducted at 28 and 33°C with varying levels of irradiance (Jones, et al., 2002).
After the first experiment took place, the zooxanthellae within the corals were counted. The results of the study showed that, when the corals were screened from UV light, no bleaching occurred, but there was a notable decrease in the amount of zooxanthellae present in the 34°C corals compared to the control corals. There was a significant decrease in the rate of respiration in the corals at 32 and 34°C, as well as an increase in nonphotochemical quenching at 34°C. Corals exposed to natural light at 34°C waters had a significant decrease in zooxanthellae numbers. Like in the UV screened corals, the respiration rate was decreased in the corals at 34°C. Corals in the 34°C waters also experienced an increase in nonphotochemical quenching, while photochemical quenching remained constant (Jones, et al., 2002).
In the second experiment, corals that were at cooler temperatures such as at 28°C had higher rates of respiration than the corals at 33°C. Corals that were illuminated by the light had lower rates of respiration than the shaded corals at both 28 and 33°C.
These findings showed that early in heat stress, Photosystem II is damaged. This is a secondary effect of damage done to the electron flow in the very first steps of the Calvin cycle. Three responses towards heat stress were observed in the zooxanthellae: an increase in the nonphotochemical quenching of chlorophyll that was unaccompanied by a significant decrease of photochemical quenching, a decrease in the quantum yield of Photosystem II, and a decrease in photosynthetic oxygen (Jones, et al., 2002). The collapse of the quenching system in heat stressed zooxanthellae corresponds with many other studies, including those that examined heat stress in leaves (Schreiber and Bilger, 1987). Because this was seen as a response to low levels of oxygen, the researchers believed that the oxygen dependent MAP-cycle was the mechanism for energizing the thylakoid. Low oxygen levels also decrease respiration, which changes the ratios of ATP/ADP and NAD(P)H/NAD(P)+. These changing ratios were believed to be the reason for decreases in the respiration rate (Jones, et al., 2002).
The overall pathway for coral bleaching is that heat stress damages the zooxanthellae in blocking the electron flow to NADP reductase. This increases the electron flow in the MAP cycle, and increases the rate of charge separation in Photosystem II. When it exceeds the capacity of electron flow for the MAP cycle, the pH increases. This induces the non-photchemical quenching of excess heat from the excess light energy, which offers a small amount of photoprotection. However, this is limited by the capacity of the electron transport chain, and if that is exceeded, then Photosystem II suffers damage, which leads to a decrease in photosynthesis and coral bleaching (Jones, et al., 2002).
In the light of global climate change, this is especially concerning, because an increase in water temperature by just 1°C over the seasonal high is enough to induce coral bleaching (Knowlton, 2001).
Heat Resistance in Zooxanthellae
In a world that is undergoing climate change, the future of coral reefs seem bleak. In a few cases, recovery after bleaching has been seen. However, this is not the norm when it comes to the fate of bleached corals. There is, however, some hope.
Zooxanthellae clades
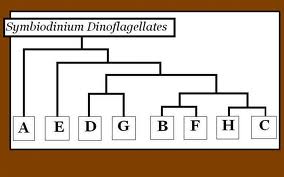
There are eight different clades of zooxanthellae that are labelled A through H as seen in the figure to the right. Each clade of zooxanthellae contains multiple species. Studies have shown that there are different tolerances for heat within each clade. Zooxanthellae that belong to clade D are known to be the most heat tolerant. In 2006, a study was conducted on Acropora millepora in order to determine the level of heat tolerance for the two types of zooxanthellae that are found within the polyps: types C and D (Berklemans and van Oppen, 2006).
The researchers transplanted corals from North Keppel Island and Davies Reef to Magnetic Island, which had a warmer climate. The corals were tested for zooxanthellae type using the nuclear ribosomal DNA internal transcriber spacer 1 during the transplant, and before running temperature experiments. Cuttings from native and transplanted corals were placed in tanks of 27.5, 30, 31, and 32°C for 15 days. The quality of the zooxanthellae was measured through the flourescence yield.
Corals from Davies Reef contained C2 zooxanthellae during transplantation, while the other transplanted corals were dominated by type D zooxanthellae with type C2 found in lower abundances. All of the transplanted North Keppel corals bleached pale to white, and seven of the colonies died. The colonies that survived all recovered and contained only type D zooxanthellae. Nine of the transplanted Davies Reef coral colonies died, and those that survived bleached pale to white, and recovered still containing C2 zooxanthellae. The researchers noted that the strain of C2 zooxanthellae were different than those found in the North Keppel corals. Corals that were native to Magnetic Island contained type D zooxanthellae, and did not bleach.
The native North Keppel corals were dominated by C2 zooxanthellae, as were the Davies Reef corals. This showed that there was a natural population drift towards the type C zooxanthellae, and similar drifts were noted to occur in coral species in the Florida Keys. Population drifts in type of zooxanthellae show that some coral species and their symbionts are more stable than others.
When the thermal tolerance of the corals were tested, the researchers saw that there was a strong link in between thermal tolerance and location. The native North Keppel corals bleached quicker and at lower temperatures, due to the climate being cooler than Davies Reef and Magnetic Island. Similarly, native and transplant corals from Davies Reef were more sensitive to heat than the Magnetic Island corals and the transplanted North Keppel corals. The researchers also found no difference in the thermal tolerance of these last two. However, the North Keppel corals had a significantly lower zooxanthellae density than the Magnetic Island corals in all temperatures except at 32°C.
These results showed that there was a link between zooxanthellae type and heat tolerance of corals. Because of the differences in the transplanted corals from Davies Reef and from North Keppel reef, thermal tolerance is believed to be determined entirely by zooxanthellae type. Because of the symbiotic relationship between zooxanthellae and coral, the zooxanthellae is the weakest link when it comes to heat and light stress. This experiment showed that some corals have the ability to switch zooxanthellae types in an attempt to adapt to warmer environments. However, this ability is limited as seen in the Davies Reef corals. And even with this switch, corals are not completely immune to heat stress. The North Keppel coral transplants gained a tolerance of waters that were about one degree warmer than their native waters. If the environment had been even warmer, the switch of zooxanthellae would not have protected the corals (Berkelmans and van Oppen, 2006).
Therefore, the type of zooxanthellae that is present within coral determines the level of tolerance that coral has for heat. Some corals are able to recover after bleaching events containing a new type of zooxanthellae that would help increase its heat tolerance in the future. However, this tolerance is limited. These findings seem to correlate with many other studies that noted that corals that recover from bleaching tend to be less sensitive to increased water temperatures, and are therefore more resistant to future bleaching events (Guest, et al., 2012).
Post Bleaching
Due to the immediate effect that coral bleaching has upon the coral itself, it is only expected that these effects would then cascade throughout the ecosystem. Because coral provide the base of the food chain in coral reef habitats, the loss of coral through bleaching events has detrimental effects, both in the short term and in the long term (McClanahan, et al., 2009).
When massive bleaching events occur, a good amount of the coral in the habitat die due to the lack of photosynthetic products. This, in turn leads to a great decrease in the amount of coral cover, which has a number of consequences. The first of these consequences is that specialized fish begin to die out as well. The fish that would be affected the most would be the corallivores, or coral eaters. These fish would experience a sharp decrease in food resources. The corallivores would then either die out due to starvation, or would migrate to areas with more coral. Loss of corallivores would ultimately lead to an imbalance in the food chain. This is because, on coral reefs, the corallivores, like herbivores, are the prey for carnivorous reef species. The loss of the corallivores would lead to a decrease in the food supply for these carnivores. Again, these carnivores would either die out or leave the affected habitat (Graham, et al., 2007). Along with decreases in fish populations, decreased coral cover also leads to sharp decreases in both reproduction and coral recruitment (McClanahan, et al., 2009).
Coral Recovery
In the long term, the ecosystem of the bleached coral changes drastically. In some cases, coral can recover, and take zooxanthellae back in. However, recovery of corals post bleaching varies between species. One study looked at the coral bleaching in the Java Sea in 1990. By 1991, the coral had recovered, however the assemblage of coral was very different after the recovery than before the bleaching event had occurred. This was also seen in a study conducted recently examined the recovery of corals in the South Andaman Islands after a bleaching event in 2010. In the summer of 2010, water temperatures reached up to 34°C, resulting in bleaching in 74% of corals in Havelock Island and 77% of the corals in Port Blair Bay. Out of these bleached corals, 21.1% of the corals in Havelock recovered, and 13.29% of corals in Port Blair Bay recovered. Coral recovery was seen in many of the massive corals such as Diploastrea heliopora, but branching corals like Acropora aspera, were still severely damaged (Marimuthu, et al., 2013). However, in order to recover, bleached corals need to be in an environment with the appropriate amount of sunlight and a small amount of sedimentation in the area (Mariumuthu, et al., 2011).
Coral Death
If the environment is not suitable for coral recovery, then bleached coral often end up dying. One example of this took place in the Ryukyus Islands in Japan in 1998. That summer, there was a major bleaching event, and most of the corals in the outer reef had died by September. By October, the corals were unable to recover, and therefore died, leaving behind calcium carbonate skeletons. The skeletons then became the home of algae, which grew to form mats on the skeletal structures. Unlike in the outer reef, the corals near the moat did not experience major coral bleaching, and therefore did not become overrun by algal mats (Shibuno, et al., 2000).
If bleaching events lead to the death of corals, then not only do we see a tremendous decrease in coral reefs, but we also see a huge change in the fish assemblages and populations on that reef. In the study at the Ryukyus Islands, the death of the corals on the outer reef led to a huge change in the types of fish that were present. The number of fish per transect did not change, however the number of species and the diversity of species greatly decreased. The number of coral-polyp feeders dwindled, while the number of herbivores greatly increased. These changes were due to the fact that much of the coral that had been bleached had later died and was overrun by algal mats. This decreased the food source for the coral polyp feeders, while increasing the food source for herbivores, leading to a change in the numbers of species that were present at the outer reef (Shibuno, et al.,1998). A similar study was conducted in 2007 in Seychelles in order to see if marine protected areas (MPAs) were effective at speeding up the recovery of coral reefs post bleaching, and if this was enough to protect the fish assemblages within reef areas. After the bleaching event, it was seen that, while there was little to no change in the numbers of larger fish, there was a great decrease in smaller fish. This was due to the decrease in coral cover, which meant a decrease in protection and food. In the long term, this would decrease the recruitment of fish to the reef, which could have negative effects for both the reef and for humans. The reef would experience greater stress from algal cover with a decrease in herbivores in the coming years, which could lead to further bleaching and coral death. For humans, decreases in recruitment of many fish can change the fishing business, making reef fish harder to find on the market (Graham, et al., 2007).
Conclusion
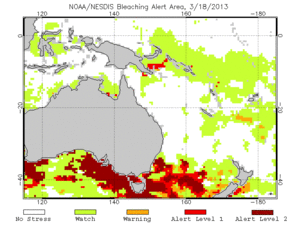
Coral bleaching has become very common and detrimental to reef environments. Elevated water temperatures and radiation are due to increased greenhouse gases in the atmosphere (Marimuthu, 2013). Increases in greenhouse gases is mostly due to human influences (Brown, 1997). In 2001, a study had noted that the diversity of coral species had decreased in the last fifteen years by 25%, and that this decrease was mostly due to increased human activity, which increased the stress for the corals (Knowlton, 2001).
In 2011, the Global Coral Reef Monitoring Network released a report on the statuses of the coral reefs in the Pacific. While currently the reefs are looking healthy and strong, the long term outlook for coral health is poor. This means that many conservation efforts are needed in order to keep the reefs from going into decline. There are a number of threats that could severely damage corals in a short time. These threats include storms, outbreaks of coral predators like the crown of thorns or Drupella, overfishing, and coral bleaching due to climate change (GCRMN, 2011 and Knowlton, 2001). Of these threats, the most troubling for the coral reefs is coral bleaching due to climate change. These threats are already common in most countries around the Pacific, and are only expected to increase in the next twenty years. While some previously damaged reefs have shown recovery, like in the South Andaman Islands, other reefs show signs of long-term decline (GCRMN, 2011 and Marimuthu, 2013). The NOAA provides up-to-date information on the health of coral reefs worldwide, and provides maps like the one on the right with information oh bleaching probabilities due to thermal stress. These maps are updated daily. (NOAA, 2013). Because there is very little data available on how corals could survive warming sea temperatures, it is hard to know how the reefs will respond to the pressures of climate change (Knowlton, 2001).
Not all hope is lost for the survival of coral reefs. Because the biggest threat to the health of coral reefs is coral bleaching due to climate change, the number one priority for reef preservation is to attack climate change at its source: human influence. Carbon dioxide emissions and other greenhouse gases are main contributors to recent climate change. By minimizing these emissions, the rate that the climate changes will start to slow, which will give the coral reefs a better chance at survival (GCRMN, 2001).
References
Berkelmans, R. and van Oppens M.J.H. "The role of zooxanthellae in the thermal tolerance of corals: a 'nugget of hope' for coral reefs in an era of climate change". Proc. R. Soc. B. 2006. Volume 273, Issue 1599. p. 2305-2312.
Brown, B.E. "Coral bleaching: causes and consequences". Coral Reefs. 1997. Volume 16, Issue 1. p. S129-S138.
Daly, M., Fautin, D.G., Cappola, V.A. "Systematics of Hexacorallia (Cnidaria: Anthozoa)". Zoological Journal of the Linnean Society. 2003. Volume 139, Issue 3. p. 419-437.
GCRMN. " Status of Coral Reefs of the Pacific and Outlook: 2011". International Coral Reef Initiative. 2011. p. 1-261
Graham, N.A.J., Wilson, S.K., Jennings, S., Polunin, N.V.C., Robinson, J., Bijoux, J.P., and Daw, T.M. "Lag effects in the impacts of mass coral bleaching on coral reef fish, fisheries, and ecosystems". Conservation Biology. 2007. Volume 21, Issue 5. p. 1291-1300.
Guest, J.R., Baird, A.H., Maynard, J.A., Muttaqin, E., Edwards, A.J., Campbell, S.J., Yewdall, K., Affendi, Y.A., and Chou, L.M. "Contrasting patterns of coral bleaching susceptibility suggest an adaptive response to thermal stress". PLoS ONE. 2012. Volume 7, Issue 3. 2012. p. 1-8.
Jones, R.J., Hoegh-Guldberg, O., Larkum, A.W.D., and Schreiber, U. "Temperature-induced bleaching of corals begins with impairment of CO2 fixation mechanism in zooxanthellae". Plant, Cell, and Environment. 2002. Volume 21, Issue 12. p. 1219-1230.
Knowlton, N. "The future of coral reefs". PNAS 2001. Volume 98, Issue 10. p. 5419-5425.
Marimuthu, N., Dharani, G., Vinithkumar, N.V., Vijayakumaran, M., and Kirubagaran, R. "Recovery status of sea anemones from bleaching event of 2010 in the Andaman waters". Current Science. 2011. Volume 101, Issue 6. p. 734-736.
Marimuthu, N., Wilson, J.J., Vinithkumar, N.V., and Kirubagaran, R. "Coral reef recovery status in south Andaman Islands after the bleaching event 2010". Journal of Ocean University of China. 2013. Volume 12, Issue 1. p. 91-96.
McClanahan, T.R., Weil, E., Cortés, J., Baird, A.H., and Ateweberhan, M. "Consequences of coral bleaching for sessile reef organisms". Ecological Studies. 2009. Volume 205. p. 121-138.
Shibuno, T., Abe, O., Hashimoto, K., and Takada, Y. "Effects of coral bleaching on the benthos and fish community in Urasoko Bay, Ishigaki Island". 2000.
Schreiber, U. and Bilger W. "Rapid assessment of stress effects on plant leaves by chlorophyll fluorescence measurement". In: Plant Response to Stress. 1987. Springer-Verlag, Berlin. p. 27-53.
Sorek, M. Yacobi, Y.Z., Roopin, M., Berman-Frank, I., and Levy, O. "Photosynthetic circadian rhythmicity patterns of Symbiodium the coral endosymbiotic algae". Proc. R. Soc. B 2013. Volume 280, Number 1759. p. 1-9.