Saccharibacteria
Classification
Higher Order Taxa
Domain: Bacteria; Phylum: Candidate Rhyla Radiation (CPR) phylum [1]; Class: Undetermined; Order: Undetermined; Family: Undetermined; Genus: Undetermined
Candidatus Saccharibacteria (TM7) is a member of the bacteria domain and the TM7 division within the Candidate Phyla Radiation (CPR) phylum [1]). Candidatus Saccharibacteria (‘Saccharibacteria) was recently discovered, so there is limited information available about its class, order, and family [1,2]. However, TM7 is currently undergoing further research, such as isolation of the pure culture, such as a culture without its host, to further identify the lower taxonomic levels within the phylum [2].
Description and Significance
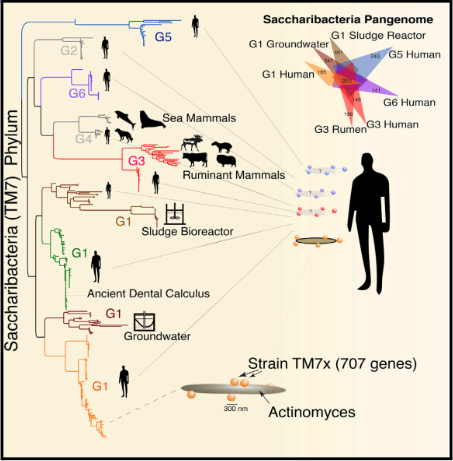
Candidatus Saccharibacteria (TM7) is a division under a large bacterial phylum (CPR). The bacterium lives as a parasite on the surface of various host cells, including mammalian, plant, and human, found in a variety of environments including soil, seawater, and many different mammalian body sites [3]. Its presence in the human oral, gastrointestinal, and urogenital microbiomes is cause for study as it may have human health effects [3]. Some of the human health effects include human oral diseases like gingivitis and periodontitis, cardiovascular disease, and indigestion in mammals [4-6]. Though there are implications of TM7’s effect on Saccharibacteria’s role as a parasite, there is a lack of understanding of TM7’s specific roles in the mammalian microbiomes and environmental impact. TM7 can purify certain contaminants in wastewater, which has indicated a potential for this group to be a key factor for environmental cleanup [7]. Currently, TM7 can only be studied with a host cell as it is resistant to culturing when isolated, presenting challenges in independent research [8].
Genome Structure
Saccharibacteria isolated from one of the common obligate hosts, Schaalia odontolytica, contained a guanosine-cytosine (G+C) content of around 42.9% [9]. The genome of this isolated Saccharibacteria contains a variety of genes that code for hundreds of proteins, and a variety of RNA molecules, such as tRNA and rRNA, both of which play a role in the translation and transcription of genes [9]. Saccharibacteria has six complete genomes: one from wastewater treatment plants [7], two from sediment [10], two from a thiocyanate remediation wastewater reactor [11], and one from the human oral cavity [12].
Across all TM7 genomes, there is conserved gene synteny, genes that lie in the same position of each chromosome. Many of the proteins show high sequence similarity across Candidatus Saccharibacteria in human saliva samples and the environment [12]. TM7 has the genomic capability to confer resistance to the antibiotic streptomycin [12]. TM7 has a high coding density genome and ranks among the smallest bacteria found in nature or the human body in both genome size and gene count. These traits align with its lifestyle as an obligate parasite in mammalian host bodies, distinguishing it from free-living bacteria [12]. TM7 lacks genes necessary for de novo biosynthesis of any essential amino acid. TM7 relies on XH00, the host bacterium of TM7 known as Actinomyces odontolyticus, metabolically due to its dependence on amino acid synthesis pathways [12].
The TM7 genome harbors a significant number of genes encoding proteins with transmembrane domains, potentially encompassing uncharacterized transporters to acquire nutrients from XH001 [12]. The TM7 genome exhibits a low percentage of genes encoding proteins with signal peptides [12].
Candidatus Saccharibacteria in the rhizosphere—the area around a plant inhabited by microorganisms like bacteria—have larger genomes relative to those that are not. These extra genes were associated with breaking down complex substances like sugars and amino acids [7]. Such genes code for enzymes utilized for substrate utilization for energy and metabolism, such as enzymes coding for the metabolism of glucose and fatty acids (oleic acid). Furthermore, Saccharibacteria contains genes that allow for energy production via the breakdown of sugar compounds such as glucose and N-acetylglucosamine, under a variety of different conditions, such as anaerobic, aerobic, and nitrate-reducing conditions [7]. Saccharibacteria contains a T4P gene cluster, which is a molecular machine for the assembly and retracting of pili for movement [13].
Cell Structure
Saccharibacteria is a small Gram-positive coccus about 200-300 nm in length [12]. ‘Saccharibacteria also contains rhodopsins, which serve as a clade of proton pumps, and ‘Saccharibacteria can transport protons across the cell membrane in response to light [14]. The presence of host bacteria triggers the movement of Saccharibacteria, via twitching motility, and the use of a T4P protein inhibitor inhibits this form of motility [15]. The capability of Saccharibacteria to utilize twitching motility via T4P proteins indicates that it is a motile bacteria [15].
TM7 is an uncultivated candidate phylum, meaning researchers have yet to replicate the growth conditions to sustain culturing of TM7 outside of their natural environment [12]. Thus, TM7 remains to be a mysterious bacterial phylum, and its pathogenicity of various other host bacteria remains an open research topic. Also, there is a lack of information describing the spore-forming abilities and the specific features of the cell wall or cell envelope of Saccharibacteria.
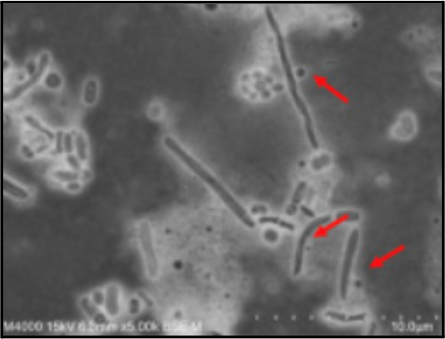
Metabolic Processes
Candidatus Saccharibacteria can ferment sugars, tolerate high oxygen concentrations, and take up molecules such as monomeric glucose, glucosamine, butyrate, oleic acid, and amino acids [7]. Different types of Saccharibacteria show diverse preferences for substrate molecules for energy, with some preferring fatty acids and N-acetylglucosamine. Candidatus Saccharibacteria cannot perform oxidative phosphorylation [7]. Candidatus Saccharibacteria plays an important role in the various metabolic pathways influencing amino acid and carbohydrate metabolism in Clanis bilineata tsingtauica larvae. Understanding alterations in the carbohydrate metabolism pathway has agricultural implications for pest control of diapause larvae or other insects [16,17].
Saccharibacteria’s ability to metabolize arginine contributes to the generation of ammonia and the alkalinity of the oral microbiome [6]. This is important for the maintenance of oral health and pH homeostasis, as a stable pH plays a role in preventing conditions such as dental caries [6]. The arginine deiminase system, which helps facilitate the breakdown of the amino acid arginine, is thought to have been acquired by Saccharibacteria during their transition from living in outdoor environments to the mammalian microbiome. Further, Saccharibacteria produces ammonia through the arginine deiminase system, which can protect its host bacteria, Actinomyces odontolyticus (XH001) [6]. Symbiosis of Saccharibacteria with its host XH001 can influence gene expression for peptidoglycan biosynthesis, cell cycle, and stress-related genes of the host. Furthermore, Saccharibacteria (TM7) shows increased expression of arginine breakdown/production genes during stable symbiosis with its host and displays higher levels of energy production and peptidoglycan production during stable symbiosis. This characteristic indicates the importance of adapting to a lifestyle in which TM7 lives on the surface of another organism [18]. There is a lack of information on TM7’s trophic category. However, TM7 has been discovered to use phototrophy by using rhodopsins and externally derived retinal [14]. Therefore, it is classified as a photoheterotroph as it utilizes phototrophy for energy but also metabolizes organic compounds as carbon sources. The lack of genes found in Candidatus Saccharibacteria for aerobic metabolic pathways, suggests that Candidatus Saccharibacteria undergoes anaerobic metabolism [7].
Ecology
TM7 is widely spread in the environment and can be found in aquatic environments such as freshwater lakes, seawater, groundwater, sediments, the rhizosphere, and sludge in wastewater treatment plants [7,19]. In soil ecosystems, certain Saccharibacteria appear to thrive in the rhizosphere, where they contribute to the breakdown of plant-derived carbon, showcasing their versatility in different ecological niches [20]. Rhizosphere Saccharibacteria also derive their energy from decaying plant matter and substances released by the plants [20]. They are also widely spread throughout the human microbiome and can be found in several niches within the body such as the oral, gastrointestinal, urogenital, and respiratory systems [3].
Genes in XH001, a bacteria closely associated with TM7, are activated or suppressed when these two bacteria are grown together. The results showed that 340 genes in XH001 changed their activity levels when they were in the presence of TM7 [12]. When XH001 and TM7 were physically close, 70 genes in XH001 were expressed at more than three times their usual levels [12]. The interaction with TM7 also caused XH001 to respond to a specific type of stress called turgor stress [12]. This stress response included an eightfold increase in a gene related to potassium leaving the cell and a threefold decrease in a gene related to potassium entering the cell [12]. These changes suggest that XH001 is trying to cope with increased pressure when it is physically connected to TM7.
When XH001 and TM7 are grown together for a long time without enough nutrients, the XH001 cells can break open (lysis). This could be linked to the changes seen in the genes and how the bacteria respond to stress. This has led to the conclusion that TM7 forms a parasitic rather than a commensal epibiont relationship with its host bacterium in the human body [12].
Pathology
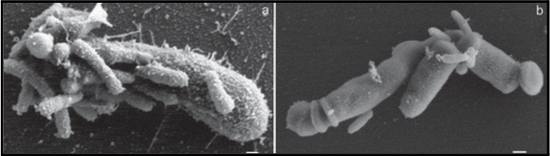
In the oral cavity, TM7 has been associated with periodontitis, a gum infection, and has been observed to modulate host bacterial pathogenicity [21]. TM7 was found to be parasitic, resulting in horizontally infecting the host bacteria [4]. Once TM7 has parasitized its host bacteria, it can down-regulate the host bacteria’s production of collagen-binding proteins. This mechanism can potentially be used to develop a treatment therapy for regulating inflammatory diseases in the dental cavity or the gastrointestinal system [4]. TM7’s virulence factor may also allow it to avoid the human body’s immune response, or detection by macrophages, which are immune cells that phagocytose pathogens [12].
Current Research
TM7 reduces the pathogenicity of host bacteria responsible for periodontitis in humans
In previous studies, TM7 was thought to initiate and exacerbate periodontal pathogenesis [22]. However, it is now known that TM7 thrives in inflammatory nutrient-rich conditions and decreases their parasitic host’s bacterial growth in the human oral microbiome [21]. In addition to preventing disease, TM7 bacteria can efficiently utilize nutrients from inflammatory tissue destruction without contributing to an inflammatory response [23]. In periodontitis patients, TM7 species showed reduced inflammation and consequential bone loss by modulating host bacterial pathogenicity by down-regulating collagen-binding proteins [21]. After stabilizing its relationship with its host bacteria, TM7 can reduce the inflammatory response caused by the host bacteria, allowing it to transition from a pathogenic to a commensal bacteria [21].
TM7 regulates the gut metabolic activity of the edible insect Clanis Bilineata tsingtauica
Based on 16S ribosomal RNA gene surveys, it is known that TM7 occurs in the rhizosphere; nevertheless, little is known about the metabolism of TM7 in various organisms [24]. diapause larvae of Clanis Bilineata tsingtauica (DLC), a unique edible insect originating in China, contains high nutritional, medicinal, and economic value due to its ability to alleviate the global food security crisis [25]. A study used CBT as a model to explore how gut microbiota can regulate the synthesis of nutrients and influence edible insects [17]. The results show that DLC maintained stable nutrition levels at an early diapause phase, and the intestinal enzyme activity in DLC fluctuated with diapause time. In addition to other bacteria, TM7 was utilized as a DLC marker species for gut microbial growth [17]. Gene function prediction analysis and Pearson correlation analysis show that TM7 in DLC was primarily involved in the biosynthesis of diapause-induced differential fatty acids, which were regulated by changing the activity of intestinal enzymes [17]. Metabolomics data revealed that TM7 was used to regulate significant differential metabolites using the metabolism of amino acid and carbohydrate pathways. By understanding these critical pathways for regulating nutrient synthesis and metabolisms in DLC, this study determined the nutrient composition of DLC larvae, which can be used to develop innovative food products [17].
Metagenomic Analysis Reveals That TM7 is Dependent on Other Bacteria for Basic Cellular Building Blocks
Previous genomic studies predicted that TM7 bacteria are anaerobic fermenters despite observing a genome encoding for a single subunit of NADH, an enzyme involved in the oxygen-scavenging mechanism of cellular respiration [11]. 13C labeling experiments have indicated that 13CO2 can be traced from plant fixation into the genetic information of rhizosphere-growing microbes [26-27]. A recent study found contradicting results [20]. Isotopic plant labeling was utilized to identify and thoroughly curate a 1.45-Mbp genome of the TM7 bacterium. The results showed that the TM7 genome lacks the necessary biosynthetic pathways to synthesize DNA de novo. The study found that the rhizosphere-associated TM7 bacteria recycle nucleotides from bacteria that feed on 13C from labeled plant exudates for DNA and RNA synthesis [20]. Isotopic labeling showed that the population was replicating during the six weeks of plant growth. The TM7 genome is about 30% larger than the genomes of non-rhizosphere TM7 bacteria [3]. Rhizosphere TM7 bacteria contain genes for complex carbon and amino acid metabolism. TM7 utilizes fermentation of soil dead organic matter to produce acetate and lactate. The genome encodes for a linear electron transport chain featuring a terminal oxidase, suggesting that this Saccharibacteria may respire aerobically [20].
References
[1] Castelle, C. J., and Banfield, J. F. “Major New Microbial Groups Expand Diversity and Alter our Understanding of the Tree of Life”. Cell. 2018. Volume 172(6). p. 1181–1197.
[2] McLean, J. S., Bor, B., Kerns, K. A., Liu, Q., To, T. T., Solden, L., Hendrickson, E. L., Wrighton, K., Shi, W., and He, X. “Acquisition and Adaptation of Ultra-small Parasitic Reduced Genome Bacteria to Mammalian Hosts.” Cell Reports. 2020. Volume 32(3).
[3] Naud, S., Valles, C., Abdourahim, A., Chacra, L. A., Mekhalif F., Ibrahim, A., Caputo, A., Baudoin, J. P., Gouriet, F., Bittar, F., Lagier, J. C., Ranque, S., Fenollar, F., Alou, M., and Raoult, D. “Preliminary landscape of Candidatus Saccharibacteria in the human microbiome.” Frontiers in Cellular and Infection Microbiology. 2023. Volume 13.
[4] Bor, B., Bedree, J. K., Shi, W., McLean, J. S., and He, X. “Saccharibacteria (TM7) in the Human Oral Microbiome.” Journal of Dental Research. 2019. Volume 98(5). p. 500–509.
[5] Schulz, S., Reichert, S., Grollmitz, J., Friebe, L., Kohnert, M., Hofmann, B., Schaller, H. G., Klawonn, F., and Shi, R. “The role of Saccharibacteria (TM7) in the subginival microbiome as a predictor for secondary cardiovascular events.” International Journal of Cardiology. 2021. Volume 331. p. 255–261.
[6] Tian, J., Utter, D. R., Cen, L., Dong, P.-T., Shi, W., Bor, B., Qin, M., McLean, J. S., and He, X. “Acquisition of the arginine deiminase system benefits epiparasitic Saccharibacteria and their host bacteria in a mammalian niche environment.” Proceedings of the National Academy of Sciences. 2022. Volume 119(2).
[7] Kindaichi, T., Yamaoka, S., Uehara, R., Ozaki, N., Ohashi, A., Albertsen, M., Nielsen, P. H., and Nielsen, J. L. “Phylogenetic diversity and ecophysiology of Candidate phylum Saccharibacteria in activated sludge.” FEMS Microbiology Ecology. 2016. Volume 92(6).
[8] Murugkar, P. P., Collins, A. J., Chen, T., and Dewhirst, F. E. “Isolation and cultivation of candidate phyla radiation Saccharibacteria (TM7) bacteria in coculture with bacterial hosts.” Journal of Oral Microbiology. 2020. Volume 12(1). p. 1814666.
[9] Cross, K. L., Klingeman, D. M., and Podar, M. Complete Genome Sequence of Human Oral Saccharibacterium “Candidatus Nanosynbacter sp. HMT352” Strain KC1. Microbiology Resource Announcements. 2022. Volume 11(2).
[10] Brown, C. T., Hug, L. A., Thomas, B. C., Sharon, I., Castelle, C. J., Singh, A., Wilkins, M. J., Wrighton, K. C., Williams, K. H., and Banfield, J. F. “Unusual biology across a group comprising more than 15% of domain bacteria.” Nature. 2015. Volume 523(7559). p. 208–211.
[11] Kantor, R. S., van Zyl, A. W., van Hille, R. P., Thomas, B. C., Harrison, S. T., and Banfield, J. F. “Bioreactor microbial ecosystems for thiocyanate and cyanide degradation unravelled with genome‐resolved metagenomics.” Environmental Microbiology. 2015. Volume 17(12). p. 4929–4941.
[12] He, X., McLean, J. S., Edlund, A., Yooseph, S., Hall, A. P., Liu, S.-Y., Dorrestein, P. C., Esquenazi, E., Hunter, R. C., Cheng, G., Nelson, K. E., Lux, R., and Shi, W. “Cultivation of a human-associated TM7 phylotype reveals a reduced genome and epibiotic parasitic lifestyle.” Proceedings of the National Academy of Sciences. 2015. Volume 112(1). p. 244–249.
[13] Xie, B., Wang, J., Nie, Y., & Du, W. “Type IV pili trigger episymbiotic association of Saccharibacteria with its bacterial host.” Proceedings of the National Academy of Science. 2022. Volume 119(49).
[14] Jaffe, A. L., Konno, M., Kawasaki, Y., Kataoka, C., Oded Béjà, Hideki Kandori, Inoue, K., and Banfield, J. F. “Saccharibacteria harness light energy using type-1 rhodopsins that may rely on retinal sourced from microbial hosts.” The ISME Journal. 2022. Volume 16(8). p. 2056–2059.
[15] He, X. “Culture-based approaches to studying ‘microbial dark matter’.” Proceedings of the National Academy of Sciences of the United States of America. 2022. Volume 120(2).
[16] Myers, P. Z. “Synteny: Inferring Ancestral Genomes.” 2008. Nature, https://www.nature.com/scitable/topicpage/synteny-inferring-ancestral-genomes-44022/
[17] Qian, L., Chen, B., Deng, P., Gui, F., Cao, Y., Qin, Y., and Liao, H. “TM7 (Saccharibacteria) regulates the synthesis of linolelaidic acid and tricosanoic acid, and alters the key metabolites in diapause Clanis bilineata tsingtauica.” Frontiers in Physiology. 2023. Volume 14.
[18] Nie, J., Utter, D. R., Kerns, K. A., Lamont, E. I., Hendrickson, E. L., Liu, J., Wu, T., He, X., McLean, J., and Bor, B. “Strain-Level Variation and Diverse Host Bacterial Responses in Episymbiotic Saccharibacteria.” MSystems. 2022. Volume 7(2).
[19] Ji, Y., Zhang, P., Zhou, S., Gao, P., Wang, B., and Jiang, J. “Widespread but poorly understood bacteria: Candidate phyla radiation.” Microorganisms. 2022. Volume 10(11). p. 2232.
[20] Starr, E., Shi, S., Blazewicz, S. J., Probst, A. J., Herman, D. J., Firestone, M. K., and Banfield, J. F. “Stable isotope informed genome-resolved metagenomics reveals that Saccharibacteria utilize microbially-processed plant-derived carbon.” Microbiome. 2018. 6(1).
[21] Chipashvili, O., Utter, D. R., Bedree, J. K., Ma, Y., Schulte, F., Mascarin, G., Alayyoubi, Y., Chouhan, D., Hardt, M., Bidlack, F., Hasturk, H., He, X., McLean, J. S., and Bor, B. “Episymbiotic Saccharibacteria suppresses gingival inflammation and bone loss in mice through host bacterial modulation.” Cell Host & Microbe. 2021. 29(11), 1649–1662.e7.
[22] Abusleme, L., Dupuy, A. K., Dutzan, N., Silva, N., Burleson, J. A., Strausbaugh, L. D., Gamonal, J., and Diaz, P. I. “The subgingival microbiome in health and periodontitis and its relationship with community biomass and inflammation.” The ISME Journal. 2013. 7(5), 1016–1025.
[23] Hajishengallis, G. “The inflammophilic character of the periodontitis‐associated microbiota.” Molecular Oral Microbiology. 2014. Volume 29(6). p. 248–257.
[24] Verneau, F., Amato, M., and La Barbera, F. L. “Edible Insects and Global Food Security.” Insects. 2021. Volume 12(5). p. 472.
[25] Zhu, H., and Wang, L. “Fauna sinica Insecta: Lepidoptera Sphingidae. Lepidoptera Sphingidae.” Science Press. 1997. Volume 11.
[26] Haichar, F. el Z., Marol, C., Berge, O., Rangel-Castro, J. I., Prosser, J. I., Balesdent, J., Heulin, T., and Achouak, W. “Plant host habitat and root exudates shape soil bacterial community structure.” The ISME Journal. 2008. Volume 2(12). p. 1221–1230.
[27] Hernández, M., Dumont, M. G., Yuan, Q., and Conrad, R. “Different bacterial populations associated with the roots and rhizosphere of rice incorporate plant-derived carbon.” Applied and Environmental Microbiology. 2015. Volume 81(6). p. 2244–2253.
Edited by Hyo Young Choi, Caleb Chung, Jan Louis Lopez, Arjan Niaki, Sanjana Prudhvi, student of Jennifer Bhatnagarfor BI 311 General Microbiology, 2023, Boston University.