Listeria monocytogenes Preservative Resistance
Section
Other examples:
Bold
Italic
Subscript: H2O
Superscript: Fe3+
Introduction
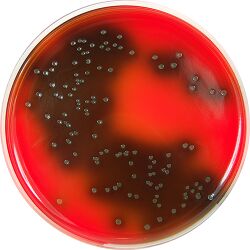
By Iris Pardue
Listeria monocytogenes is a facultatively anaerobic Gram-positive food pathogen of the phylum Bacillota capable of surviving and growing at low temperatures. As a result, it is responsible for infections in deli meats, cheeses, and other refrigerated products.[1] Outbreaks are relatively frequent, and serious cases have an especially high fatality rate of 15.26% over recorded cases in the US in the last decade. Unsurprisingly, much research has been conducted into control of Listeria as a result. Many different methods are used by food supply companies to inhibit bacterial growth, and chief among these methods are chemical preservatives. There are several different types of preservatives commonly used[2], and many have different mechanisms of action that will be discussed later. Having a diverse portfolio of antimicrobial preservatives available is especially important given the ability of pathogens to quickly evolve resistance to common mechanisms. This article explores the variety of commonly used preservatives relevant to controlling listeriosis along with the susceptibilities and resistances possessed by Listeria to each.
Cold Resistance
To fully understand the resistance of Listeria to preservatives, it is important to first consider its most importance resistance to cold. Listeria is a psychrophile, capable of growing at freezing temperatures as well as at human body temperatures. [3] Numerous mechanisms exist to aid resistance to cold, but chief among them is adaptations in the content of the phospholipid membrane. This adaptation is mainly achieved via the use of the two primary fatty acids in the lipid membrane, anteiso-17-0 and anteiso-15-0.[4] The proportion between these two fatty acids is modulated in response to low temperatures until the more flexible anteiso-15-0 dominates, reaching 80% of the total fatty acid profile. This adaptation allows it to maintain the crucial "liquid-crystal" state, which is necessary for cell function[5] by lowering the melting point of the membrane. However, the focus on lipids alone can paint an oversimplified picture of the cell membrane's temperature stability. In many species, cholesterol acts as a cellular antifreeze to aid membrane stability[6], and Listeria employs a similar mechanism using isoprenoid quinones[4]. Menaquinone-7 (MK-7), also known as vitamin K2, is composed of a napthaquinone ring fixed to a long chain of 7 isoprene units, which allows it to integrate into the membrane, a structure shared with the quinones used in electron transport. Flegler et al. found that strains containing higher levels of these molecules in their membranes better survived temperature stresses and did not adapt their fatty acid composition to the same degree as other strains. However, product feedback inhibition of the shikimate pathway responsible for synthesis of aromatic amino acids that are processed into quinones did reduce the ability of high MK-7 strains to adapt to the lower temperatures, providing a possible target for future preservatives. Indeed, a drug targeting chorismate synthase, an enzyme that catalyzes a crucial transformation in the shikimate pathway[7] reportedly showed promising antimicrobial activity against Listeria and other species, and could be developed into a future food additive.[8]
Acid Preservatives
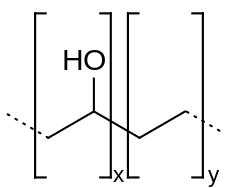
A large number of food preservatives are organic acids capable of antimicrobial activity while maintaining levels safe for human consumption, such as sorbic acid, benzoic acid, lactic acid, and ascorbic acid.[2] It is generally accepted that they pass easily through the membrane as neutral, protonated molecules that dissociate to toxic anions within the cell that exhibit a variety of antimicrobial effects; however, transport of organic acids via anion channels is also possible.[9] Additionally, the change in external pH induced by these preservatives can force Listeria to activate its acid tolerance responses. Studies have found that proton stress has a notable effect on the C15:C17 ratio of the membrane, potentially compromising the methods used to resist colder environments.[10] Use of organic acids in particular resulted in numerous additional membrane changes, including a further decrease in the C15:C17 ratio and a higher level of C18 fatty acids that could further compromise the cold stress response. However, these results were collected at pH 6.0, which is well above the pKa of most organic acids used for preservatives.[11] As a result, these acids were primarily in their anionic state in which they cannot pass the membrane, and the "toxic anion" effect may not be as intense as it is in foods like yogurt that primarily exist at pH 4.6 or below.[12] Further research at lower pH might reveal a more intense version of these same effects or perhaps a different set of effects entirely. A different study conducted at pH 5.5 revealed that the membrane changes induced by organic acid stress do in fact increase the melting point of the membrane, possibly affecting its ability to remain in a liquid-crystal state.[13] However, from the resilience of Listeria to continue growing on acidic substrates in refrigerated environments, there is evidently some mechanism of resistance to acid that allows it to survive, despite potential membrane adaptations that could harm its cold resistance. Listeria does not form spores[14], and thus cannot stay dormant in these conditions in a similar fashion to other Bacillota. Indeed, research indicates that Listeria is capable of habituating to acids, a trait necessary for both survival in acidic foods and infecting the human gut.[15] However, the mechanisms of action of the toxic organic anions are yet to be elucidated in detail.
Soluble or volatile acids are not the only way for these acids to exercise an antimicrobial effect. The active ethylene vinyl alcohol copolymer (EVOH) is already widely used in food preservation as a gas-impermeable layer that prevents oxidation of a food and inhibits aerobic microbe growth[16] This alone is not enough to impede Listeria growth due to its facultatively anaerobic nature, but research has been done on combining EVOH with other materials to enhance antimicrobial properties. Sorbic acid-chitosan microcapsules mixed evenly into 38% ethylene EVOH showed increased antimicrobial ability against E. coli, Salmonella enteriditis and Listeria, although the effect on Listeria was less pronounced.[17] The sorbic acid was capable of diffusing into multiple food simulants over the course of several days, potentially extending the shelf life of Listeria-susceptible foods by a significant amount. A variety of different chemicals could be packaged into capsules in this way, which may open up effective antimicrobial agent delivery to new molecules or improve effectiveness of common organic acid preservatives.
Acid Preservatives
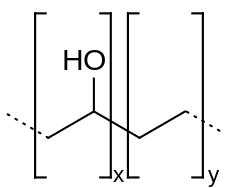
A large number of food preservatives are organic acids capable of antimicrobial activity while maintaining levels safe for human consumption, such as sorbic acid, benzoic acid, lactic acid, and ascorbic acid.[2] It is generally accepted that they pass easily through the membrane as neutral, protonated molecules that dissociate to toxic anions within the cell that exhibit a variety of antimicrobial effects; however, transport of organic acids via anion channels is also possible.[18] Additionally, the change in external pH induced by these preservatives can force Listeria to activate its acid tolerance responses. Studies have found that proton stress has a notable effect on the C15:C17 ratio of the membrane, potentially compromising the methods used to resist colder environments.[10] Use of organic acids in particular resulted in numerous additional membrane changes, including a further decrease in the C15:C17 ratio and a higher level of C18 fatty acids that could further compromise the cold stress response. However, these results were collected at pH 6.0, which is well above the pKa of most organic acids used for preservatives.[19] As a result, these acids were primarily in their anionic state in which they cannot pass the membrane, and the "toxic anion" effect may not be as intense as it is in foods like yogurt that primarily exist at pH 4.6 or below.[20] Further research at lower pH might reveal a more intense version of these same effects or perhaps a different set of effects entirely. A different study conducted at pH 5.5 revealed that the membrane changes induced by organic acid stress do in fact increase the melting point of the membrane, possibly affecting its ability to remain in a liquid-crystal state.[21] However, from the resilience of Listeria to continue growing on acidic substrates in refrigerated environments, there is evidently some mechanism of resistance to acid that allows it to survive, despite potential membrane adaptations that could harm its cold resistance. Listeria does not form spores[22], and thus cannot stay dormant in these conditions in a similar fashion to other Bacillota. Indeed, research indicates that Listeria is capable of habituating to acids, a trait necessary for both survival in acidic foods and infecting the human gut.[23] However, the mechanisms of action of the toxic organic anions are yet to be elucidated in detail.
Soluble or volatile acids are not the only way for these acids to exercise an antimicrobial effect. The active ethylene vinyl alcohol copolymer (EVOH) is already widely used in food preservation as a gas-impermeable layer that prevents oxidation of a food and inhibits aerobic microbe growth[24] This alone is not enough to impede Listeria growth due to its facultatively anaerobic nature, but research has been done on combining EVOH with other materials to enhance antimicrobial properties. Sorbic acid-chitosan microcapsules mixed evenly into 38% ethylene EVOH showed increased antimicrobial ability against E. coli, Salmonella enteriditis and Listeria, although the effect on Listeria was less pronounced.[25] The sorbic acid was capable of diffusing into multiple food simulants over the course of several days, potentially extending the shelf life of Listeria-susceptible foods by a significant amount.
Inorganic Preservatives

Nitrites are a common inorganic preservative in meat processing, often added as sodium nitrite (NaNO2) during the curing process to reduce oxidation, limit microbe growth (especially of Clostridium), and provide the unique flavor of cured meats.[27] However, their levels must be tightly controlled due to their formation of carcinogenic nitrosamines within the body[28] and thus must be used in limited concentrations. Nitrite does reduce growth of Listeria on spreadable sausages at marketable concentrations, an effect that increased significantly with lower pH until growth was completely ablated at pH 5.0.[29] When the bacteria were allowed to adapt, the transcriptome was largely modified to favor stress-related processes as opposed to central cellular processes such as membrane expansion, translation and genome repair. Interestingly, many of these genes are similar to those upregulated when the cells are phagocytosed by macrophages, an environment where oxidative and acidic stressors are common in the lysosome. The majority of differentially expressed genes were poorly characterized, which further highlights the need for more research on the mechanism of action of Listeria habituation to preservatives. The number of listeriosis infections has increased in recent years[30], which may be due to a developing resistance to traditional preservation methods like nitrite curing. Nitrite's mechanism of action is mainly due to highly oxidative radical forms of the molecule that exist via reactions with superoxide (a radical form of dioxygen) to form peroxynitrate.[31] Listeria is capable of detoxifying superoxide with an oxygen- and salt-inducible superoxide dismutase[32], which would inhibit peroxynitrite formation. This combined with the ability of Listeria to grow anaerobically where superoxide cannot activate nitrites to their radical forms suggests that it likely possess a strong innate resistance to nitrite, as evidenced by continued contamination of cured meats. This resistance could be provided by catalase or superoxide dismutase, both of which are known to be present in Listeria[32] and contain metal ion centers that could catalyze a reaction to detoxify peroxynitrite, as suggested at right.
Regulation
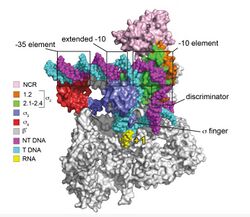
In bacteria, the genetic response to stress is controlled by a variety of factors, but chief among these is the change in the sigma factor used to initiate transcription. In Listeria and other Bacillota, this is controlled mainly by the sigma b (σB) factor associated with its general stress response.[34][35] σB, like all sigma factors, binds to the bacterial core promoter in order to recruit RNA polymerase by mediating the attachment between preserved DNA elements and the β' subunit of the polymerase, forming the RNA polymerase holoenzyme. A number of different sigma factors are expressed, but σB specializes in transcription of genes involved in the starvation and stress response. In Bacillus subtilis, it is involved in regulating the sporulation cascade, but presumably regulates a different group of genes in Listeria due to its lack of sporulation.[36]. ΔsigB mutants are known to be more susceptible to cold and organic acid stresses, and a total of 198 genes show lower expression compared to wild-type strains grown at 3° C.[37] The σB regulon appears to overlap largely with the σL regulon under these conditions, and a ΔsigBL double knockout mutant showed even higher susceptibility to the aforementioned stressors. Some biosynthetic genes such as components of the phosphoryltransferase system, purine ribonucleotide synthesis genes, and ribosomal genes remained downregulated at 3° C. It is unclear whether this is due to a loss of sigma factors or a result of other stress-related transcription factors slowing growth-related biosynthesis to preserve other functions. Interestingly, flagella-associated genes including some flagellins were downregulated in ΔsigBL mutants, resulting in a significant loss of motility.
Conclusion
References
- ↑ Ranjan K. Mohapatra, Snehasish Mishra, Lawrence Sena Tuglo, Ashish K. Sarangi, Venkataramana Kandi, Amani Ahmed AL Ibrahim, Hussain A. Alsaif, Ali A. Rabaan, Md. Kudrat-E Zahan. Recurring food source-based Listeria outbreaks in the United States: An unsolved puzzle of concern? Health Science Reports 2024 7:2.
- ↑ 2.0 2.1 2.2 Elisabeth Anderson. Preservatives – Keeping our foods safe & fresh.
- ↑ Jones GS, D'Orazio SEF. Listeria monocytogenes: cultivation and laboratory maintenance. Curr Protoc Microbiol. 2013 Nov 5;31:9B.2.1-9B.2.7.
- ↑ 4.0 4.1 Alexander Flegler, Vanessa Kombeitz & André Lipski. Menaquinone-mediated regulation of membrane fluidity is relevant for fitness of Listeria monocytogenes. Arch Microbiol 203, 3353–3360.
- ↑ Richard Templer and John Seddon. The World of Liquid Crystals. NewScientist 18 May 1991.
- ↑ Singer SJ, Nicolson GL. The fluid mosaic model of the structure of cell membranes. Science. 1972 Feb 18;175(4023):720-31.
- ↑ Macheroux P, Schmid J, Amrhein N, Schaller A. A unique reaction in a common pathway: mechanism and function of chorismate synthase in the shikimate pathway. Planta. 1999 Jan;207(3):325-34.
- ↑ Hossain, M.M., Roy, P.K., Mosnaz, A.T.M., Shakil, S.K., Hasan, M.M. Prodhan, S.H. Structural analysis and molecular docking of potential ligands with chorismate synthase of Listeria monocytogenes: A novel antibacterial drug target. Indian Journal of Biochemistry and Biophysics 52(1) 45-59 February 2015.
- ↑ Theron, MM and Lues, JFR 2005. Organic acids: promising modern preservatives. Interim 4(2) 151-161.
- ↑ 10.0 10.1 Yimin Zhang, Charles E. Carpenter, Jeff R. Broadbent, Xin Luo. Influence of habituation to inorganic and organic acid conditions on the cytoplasmic membrane composition of Listeria monocytogenes. Food Control 55 49-53 September 2015.
- ↑ Ionization Constants of Organic Acids. Michigan State University
- ↑ FDA Amends Standard of Identity for Yogurt, 13 April 2023
- ↑ Ioannis Diakogiannis, Anita Berberi, Eleni Siapi, Angeliki Arkoudi-Vafea, Lydia Giannopoulou, Sofia K. Mastronicolis. Growth and membrane fluidity of food-borne pathogen Listeria monocytogenes in the presence of weak acid preservatives and hydrochloric acid. Front. Microbiol. 4, 13 June 2013.
- ↑ Chakraborty T. Molecular and cell biological aspects of infection by Listeria monocytogenes. Immunobiology. 1999 Dec;201(2):155-63.
- ↑ Yimin Zhang, Charles E. Carpenter, Jeff R. Broadbent, Xin Luo. Habituation to organic acid anions induces resistance to acid and bile in Listeria monocytogenes. Meat Science 96(3) 1152-1157 March 2014.
- ↑ Impact Plastics. What is EVOH and How is it Used in Food Packaging? 7 September 2018.
- ↑ Shuaifeng Hu, Jie Yu, Zhe Wang, Li Li, Yunfei Du, Liping Wang, Yuan Liu. Effects of Sorbic Acid-Chitosan Microcapsules as Antimicrobial Agent on the Properties of Ethylene Vinyl Alcohol Copolymer Film for Food Packaging. Journal of Food Science 82(6) 1451-1460 June 2017.
- ↑ Theron, MM and Lues, JFR 2005. Organic acids: promising modern preservatives. Interim 4(2) 151-161.
- ↑ Ionization Constants of Organic Acids. Michigan State University
- ↑ FDA Amends Standard of Identity for Yogurt, 13 April 2023
- ↑ Ioannis Diakogiannis, Anita Berberi, Eleni Siapi, Angeliki Arkoudi-Vafea, Lydia Giannopoulou, Sofia K. Mastronicolis. Growth and membrane fluidity of food-borne pathogen Listeria monocytogenes in the presence of weak acid preservatives and hydrochloric acid. Front. Microbiol. 4, 13 June 2013.
- ↑ Chakraborty T. Molecular and cell biological aspects of infection by Listeria monocytogenes. Immunobiology. 1999 Dec;201(2):155-63.
- ↑ Yimin Zhang, Charles E. Carpenter, Jeff R. Broadbent, Xin Luo. Habituation to organic acid anions induces resistance to acid and bile in Listeria monocytogenes. Meat Science 96(3) 1152-1157 March 2014.
- ↑ Impact Plastics. What is EVOH and How is it Used in Food Packaging? 7 September 2018.
- ↑ Shuaifeng Hu, Jie Yu, Zhe Wang, Li Li, Yunfei Du, Liping Wang, Yuan Liu. Effects of Sorbic Acid-Chitosan Microcapsules as Antimicrobial Agent on the Properties of Ethylene Vinyl Alcohol Copolymer Film for Food Packaging. Journal of Food Science 82(6) 1451-1460 June 2017.
- ↑ Szabó, C., Ischiropoulos, H. & Radi, R. Peroxynitrite: biochemistry, pathophysiology and development of therapeutics. Nat Rev Drug Discov 6, 662–680 (2007).
- ↑ Shakil MH, Trisha AT, Rahman M, Talukdar S, Kobun R, Huda N, Zzaman W. Nitrites in Cured Meats, Health Risk Issues, Alternatives to Nitrites: A Review. Foods. 2022 Oct 25;11(21):3355.
- ↑ Tricker AR, Preussmann R. Carcinogenic N-nitrosamines in the diet: occurrence, formation, mechanisms and carcinogenic potential. Mutat Res. 1991 Mar-Apr;259(3-4):277-89.
- ↑ Stefanie Müller-Herbst, Stefanie Wüstner, Jan Kabisch, Rohtraud Pichner, Siegfried Scherer. Acidified nitrite inhibits proliferation of Listeria monocytogenes — Transcriptional analysis of a preservation method. International Journal of Food Microbiology 226 33-41 2 June 2016
- ↑ Choi MH, Park YJ, Kim M, Seo YH, Kim YA, Choi JY, Yong D, Jeong SH, Lee K. Increasing Incidence of Listeriosis and Infection-associated Clinical Outcomes. Ann Lab Med. 2018 Mar;38(2):102-109.
- ↑ Didier Majou, Souad Christieans. Mechanisms of the bactericidal effects of nitrate and nitrite in cured meats. Meat Science 145 273-284 November 2018.
- ↑ 32.0 32.1 Dallmier AW, Martin SE. Catalase, superoxide dismutase, and hemolysin activities and heat susceptibility of Listeria monocytogenes after growth in media containing sodium chloride. Appl Environ Microbiol 56 1990.
- ↑ Paget, M.S. Bacterial Sigma Factors and Anti-Sigma Factors: Structure, Function and Distribution. Biomolecules 2015, 5, 1245-1265.
- ↑ Yichang Liu, Renato H Orsi, Ahmed Gaballa, Martin Wiedmann, Kathryn J Boor, Veronica Guariglia-Oropeza. Systematic review of the Listeria monocytogenes σB regulon supports a role in stress response, virulence and metabolism. Future Microbiology 14(9) 4 July 2019.
- ↑ Thulandi Sibanda and Elna M. Buys. Listeria monocytogenes Pathogenesis: The Role of Stress Adaptation. Microorganisms 10(8). 31 May 2022.
- ↑ Gruber TM, Gross CA. Multiple sigma subunits and the partitioning of bacterial transcription space. Annu Rev Microbiol. 2003;57:441-66.
- ↑ Mattila, Mirjami, Panu Somervuo, Hannu Korkeala, Roger Stephan, and Taurai Tasara. Transcriptomic and Phenotypic Analyses of the Sigma B-Dependent Characteristics and the Synergism between Sigma B and Sigma L in Listeria monocytogenes EGD-e. Microorganisms 8(11) 28 September 2020.
Authored for BIOL 238 Microbiology, taught by Joan Slonczewski,at Kenyon College,2024