Plasmodium
A Microbial Biorealm page on the genus Plasmodium
Classification
Higher order taxa
Eukaryota; Alveolata; Apicomplexa; Haemosporida
Species
Plasmodium falciparum
Plasmodium ovale
Plasmodium vivax
Plasmodium malariae
Description and Significance
Plasmodium, the parasite responsible for human malaria, is among the most researched genera of parasites in the world. Despite extensive studies on possible control methods, infection in humans continues to grow in tropic and sub-tropic areas. Currently there are an estimated 500,000,000 infected persons, with 1-2 million dying annually. There are four types of Plasmodium which cause human malaria: Plasmodium falciparum, Plasmodium ovale, Plasmodium vivax, and Plasmodium malariae. All of these are transmitted to human hosts solely by way of Anophele mosquito vectors. Plasmodium is one of the oldest known parasites; its long history suggests a long, adaptive relationship with the human host. Today cases of the disease are increasing in non-malarious countries as more people travel to Africa, India, Brazil, and some Asian nations, where the mosquito vectors are most prevalent. Symptoms of the disease may go unnoticed or misdiagnosed; clinical signs include fever, chills, weakness, headache, vomiting, diarrhea, anemia, pulmonary and renal dysfunction, neurologic changes. Untreated malaria may result in death.
Genome Structure
The genome of the most common form of Plasmodium which causes human malaria, Plasmodium falciparum, has been sequenced completely, yielding 14 chromosomes and 5,300 genes--a large number of which are responsible for dodging the host's immunities. The average gene density is approximately 1 gene/4,338 base pairs. The mapping of this genome sequence provides new avenues for research on possible vaccines. Click here for a complete analysis of Plasmodium falciparum's genome sequence.
Cell Structure and Metabolism
While the four major species of Plasmodium differ in some ways from each other, they all share the same complex life cycle involving the insect (mosquito) vector and the human host. When an infected Anophele mosquito bites a human, sporozoites are injected with the saliva. The sporozoites are 10 -15 µm in length and about 1 µm in diameter. They have a thin outer membrane, a double inner membrane below which lies the subpelicular microtubules. They have 3 polar rings and the rhoptries are long, extending half the length of the body. The micronemes, convoluted elongate bodies, run forward to the anterior of the sporozoite entering a common duct with the rhoptries. Mitochondria are located at the posterior end. After entering the circulatory system, the sporozoites make quick work of invading liver cells using the apical organelles (characteristic of all apicomplexans; for more details, see Apicomplexa).
Inside the host's liver cell the Plasmodium cell undergoes asexual replication. The products of this replication, called merozoites, are released into the circulatory system. The merozoites invade erythrocites and become enlarged ring-shaped trophozoites. In this stage the cells ingest the host cytoplasm and proteolyze hemoglobin into amino acids. Several rounds of nuclear divison yield a schizont. From these schizonts merozoites bud, which are released after rupturing the erythrocites. More erythrocites are invaded, and the cycle is reinitiated.
Sometimes instead of schizogony (as the schizont cycle is known) the parasites will reproduce sexually into micro- or macrogametocytes. Through gametogenesis these micro- or macrogametocytes morph into micro- or macrogametes. This may only occur after the gametocytes have been ingested by a mosquito. After said ingestion, the microgametocyte undergoes three nuclear divisions; the resulting eight nuclei become associated with thrashing flagella (this process is called exflagellation). The highly motile microgametes fuse with macrogametes and produce a zygote, which then develops into an ookinete. Once reaching the space between the epithelial cells and the basal lamina of the host, the ookinete develops into an oocyst. Asexual replication results in the production of a large number of sporozoites, which are released into the body cavity of the mosquito vector upon the maturation of the oocyst. The sporozoites are able to recognize the salivary gland of the vector, and are injected into the vertebrate host during the mosquito's blood meal, thus beginning the process over again. The four species of Plasmodium that affect humans are different morphologically, slightly in terms of their life cycles, in terms of their host erythrocite preferences, and varying clinical symptoms.
Images from the College of Biological Medicine, Ohio State University
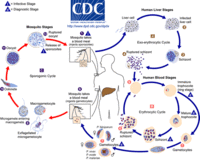
Malaria
Ecology of Malaria
Plasmodium is a parasite which is widely distributed all over the world. Because it requires warm, humid environments for replication in the insect vector, malaria-generating species of Plasmodium are generally limited to tropical and sub-tropical locations. Global warming and population migrations do have a bearing on Plasmodium's distribution. Plasmodium falciparum is the most widespread in tropical and sub-tropical areas. Plasmodium ovale is most prevalent in the west coast region of Africa. Plasmodium malariae has a widespread distribution area but is fairly scattered within this area. Plasmodium vivax, like falciparum, ranges over a wide area, but in relatively rare in African countries. A number of methods of control have been tested and some, including use of DDT, have proved worthwhile, but drug resistance and other health concerns make some of these methods undesirable.
History of Malaria
History
The first apparent mention of malaria-like symptoms was recorded in the ancient Nei Ching (The Canon of Medicine) about 4700 BCE. These writings also included many malaria treatments, among them the use of the Qinghao plant to reduce fever (1). Writings can also be found in cuneiform script on clay tablets and ancient Indian, Sumerian and Egyptian texts (2). Hippocrates recorded manifestations of malaria in the 4th century BCE as well as the time of year and place where the victim fell ill. Hippocrates was apparently the first to distinguish the intermittent fever of malaria from the continual fever of other infectious diseases. Malaria was long known to be associated with swamps. It was thought to be transmitted by wind, and its modern name in English is derived from the Italian mal'aria from mala aria, literally "bad air." The name was possibly first used by an Italian physician Francisco Torti (1658-1741). In 1880, Charles Louis Alphonse Laveran, a French physician, discovered the parasite that causes malaria in humans, receiving the Nobel Prize for Medicine and Physiology in 1907. Later, Laveran went on to investigate the existence of the parasites outside the human body. He deduced that “the marsh fever parasite must undergo one phase of its development in mosquitoes, and be inoculated into humans by their bites” (3). In 1886 Camillo Golgi, who won the Nobel prize in 1906 for his discoveries in neurophysiology also studied malaria and found that there are at least two forms of malaria. One form produced a fever every other day and was called tertian. The other produced a fever every third day and was called quartan. In 1890, Giovanni Batista Grassi and Raimondo Filetti, Italian investigators, introduced the names Plasmodium vivax and P. malariae ‘ for the two of the four malaria parasites which are now known to affect humans. In 1897, American William H. Welch revisited Laveran’s work and named a third malaria parasite P. falciparum. Finally in 1922, John W. W. Stephens found a fourth malaria parasite affecting humans, P. ovale. Ronald Ross, a British officer, discovered in 1897 that humans are able to pass the malaria parasite into mosquitoes by releasing malaria-free mosquitoes into the rooms of malaria patients. The actual life or sporogonic cycle of the parasites was first described in 1899 by Giovanni Batista Grassi (4).
A worldwide effort to eradicate endemic malaria was begun in 1955 by the World Health Organization. Their efforts included the spraying of houses with residual insecticides, anti-malarial drug treatment, and continual surveillance (4). This effort was successful in countries with a temperate climate and seasonal transmission of malaria, including Europe and Australia. Many other countries including India and Sri Lanka initially had marked reductions in cases. However these and other countries had little success long term, including all of sub-Saharan Africa. Failure to eradicate malaria derives from many causes. The most important are lack of funding, especially for long term efforts, wars and resulting population disturbances and emerging strains of drug resistant malaria, including resistance of mosquitoes to insecticides. Of particular concern currently is the rise in the Mekong region along the Thai-Cambodian border of Plasmodium strains resistant to the highly effective artem/sinin drugs (5).
References
1. Hsu, E. (2005) “The history of qing hao in the Chinese materia medica,” Trans. Royal Soc. Trop. Med. Hygiene 100:505-508.
2. http://www.malariasite.com/malaria/history_literature.htm
3. Sundberg, C. (1907) Presentation Speech for the Nobel Prize in Physiology or Medicine, http://nobelprize.org/nobel_prizes/medicine/laureates/1907/press.html
4. http://www.cdc.gov/malaria/history/index.htm
5. WHO press release, http://www.wpro.who.int/media_centre/press_releases/pr20090424.htm
Diagnosis
The diagnosis of malaria is based on multiple symptoms but eventually must be made from detection of the parasite via laboratory tests.6 Early symptoms include chills, sweats, fever, muscle pain, nausea, and vomiting which may appear 7 to 30 days after initial contraction of the parasite. The incubation period varies depending on the strain of the parasite. Since these symptoms resemble the flu, malaria can be difficult to diagnose in places where it is not endemic, such as the United States. With severe malaria, caused by P. falciparum, victims begin to experience more intense symptoms including neurological focal signs, anemia, confusion, dypsnea, and even coma. Again, these symptoms are shared with many other diseases. Consequently, the only way to diagnose malaria is to conduct laboratory tests. For definitive diagnosis, a drop of the patient’s blood is stained (usually with Giemsa stain) and examined under a microscope for the presence of parasites. Diagnostic kits using a dye-labeled antibody that binds to the parasite are also available and are especially useful in the field and for rapid diagnoses. These tests are becoming more important in the diagnosis of malaria. They do not require a microscope or a skilled technician. In Africa and other countries without adequate medical infrastructure, these tests are an essential tool in detecting the parasite.
Treatment of Malaria
Treatment of malaria (1) depends on the species of the infecting parasite and the density of that parasite in the bloodstream. Other relevant factors for treatment include the parasite’s drug resistance status and the country where the parasite was contracted (2). Treatment also depends on any accompanying illnesses and drug allergies. The World Health Organization suggests that treatment begin within 24 hours after symptoms appear, particularly with a Plasmodium falciparum infection because of its rapid progression towards severe malaria. If the species of parasite cannot be immediately identified, the patient should be treated as if infected with P. falciparum until the infecting species can be identified.
The drug(s) administered depends on the identified parasite species and drug resistance in the region where the parasite was acquired. Travel history is especially crucial in the identification and drug resistance process (3). Anti-malaria drugs can be given orally, intravenously, or as a suppository determined by the severity of the infection. Most drugs are given when the parasite has a high density in the bloodstream. For example, patients who have P. falciparum are given continuous IV infusions because oral medication will not be effective.
For initial treatment of P. falciparum, chloroquine remains the treatment of choice in areas without chloroquine-resistant strains. In areas with chloroquine resistance, three different treatment options may be given: a) a combination of atovaquone and proguanil, b) oral quinine plus tetracycline, doxycycline, or clindamycin or c) 3 –mefloquine (4).
For P. vivax and P. ovale, primaquine is used for initial treatment. These two species become latent in the liver, but primaquine is effective in preventing the spread of the parasite into the bloodstream (4). The parasite may become active and spread into the bloodstream from the liver causing the patient to have a relapse of malaria. This occurs in about 20% of patients. For P. vivax infections, chloroquine can be used with hydroxychloroquine as a second-line alternative.
The most effective new drugs are the artemisinins. They are currently the most effective agents against multi-resistant malaria strains. Ironically, they are derived from compounds from the most ancient treatment known, qinghao tree (annual Chinese wormwood, Artemesia annu). Artemisinins clear parasites more rapidly than quinine during the first 24 hours of treatment (5). However, these drugs are very expensive and are not yet widely available. And, as with all antibiotics, resistance has already arisen (6).
Severe malaria requires a different approach because of the risk of the explosive release of parasites into the blood. There are two treatments for severe malaria: quinidine or an exchange transfusion. Quinidine is currently the recommended treatment in the United States, but treatment is problematic because quinidine is cardiotoxic (4). The drug is usually administered in an intensive care unit with continuous electrocardiographic and frequent blood pressure monitoring because it can cause arrhythmia. Sometimes because of the severity of malaria, a drug cannot be used at all. The patient must receive an exchange transfusion, where a patient’s red blood cells and platelets are replaced with transfused blood products. This is then followed by appropriate drug treatment.
References
1. http://www.cdc.gov/malaria/diagnosis_treatment/diagnosis.htm,
2. http://www.cdc.gov/malaria/diagnosis_treatment/treatment.htm
3. http://www.cdc.gov/malaria/travel/index.htm
4. Griffith, K.S, Lewis, L.S., Mali, S. and Parise, M.E. (2007) “Treatment of Malaria in the United States,” J Amer Med Assoc 297:2264-2277
5. Gomes, M., Ribeiro, I., Warsame, M., Karunajeewa, H. and Petzold, M. (2008) “Rectal artemisinins for malaria: a review of efficacy and safety from individual patient data in clinical studies” BMC Infectious Diseases 8:39
6. 5. WHO press release, http://www.wpro.who.int/media_centre/press_releases/pr20090424.htm
Prevention
The Centers for Disease Control advises travelers going into malaria endemic countries to take prophylactic measures. Chemoprophylaxis should be started before travel and taken continuously while in malaria-endemic areas. Once a traveler leaves a malaria endemic area, continued drug treatment should be undertaken, but with different drugs to minimize the possibility of drug resistance. The CDC publishes the “Travelers’ Health Yellow Book” to provide information on the potential risk of malaria, and other diseases, in each country (http://www.cdc.gov/malaria/travel/index.htm)
The Socioeconomic Link of Malaria
Traditionally, malaria has been thought to represent a “disease of the poor” or a “disease of poverty.” This is primarily a simple correlation with inadequate access to health care and poor public health infrastructure (1-3).
Incidence rates and vulnerability to malaria are linked to low socioeconomic status. Areas with concentrated populations of people with low socioeconomic status typically do not have resources for effective prevention and subsequent treatment. For example, a study conducted by Gwatkin and Guillot showed “58% of malaria deaths occurred in the poorest 20% of the world’s population, a higher percentage than any other disease of major public health importance” (1). Public health interventions intended to reach areas with people of low socioeconomic status may not be reaching their target population. Barat et al. note that in developing countries, the poor “often live in the most remote areas and are socially or culturally marginalized” (2). Consequently, while programs in support of malaria prevention and treatment are sometimes available, they are not reaching the people who need them the most.
Another link to increased vulnerability is the lower level of education associated with lower socioeconomic status. Without knowledge of the threat of malaria, preventative measures, possible treatment options, and undiagnosed and/or untreated infection may persist” (3). Individuals with lower socioeconomic status may also be less likely to seek treatment because of the belief that they are not eligible, either because of availability or cost of treatment options. The distance from health services and costs of lost work time may also deter people with low socioeconomic status from seeking treatment (2).
References
1. Worrall, E., Basu, S. and Hanson, K. (2005) “Is malaria a disease of poverty? A review of the literature,” Tropical Med and Intl Health 10:1047-1059.
2. Barat, L.M., Palmer, N., Basu, S., Worrall, E., Hanson, K. and A. Mills (2004). Do malaria control interventions reach the poor? A view through the equity lens,” Amer J Tropical Med Hygeine 71 (Suppl 2):174-178.
3. Worrall, E., Basu, S., and Hanson, K. (2003) “The relationship between socioeconomic status and malaria: a review of the literature” SES and Malaria 1-46, accessed at http://siteresources.worldbank.org/INTMALARIA/Resources/SESMalariaBackgroundPaper.pdf
References
Malaria Introduction. The Walter and Eliza Hall Institute of Medical Research.
Malaria. Parasites and Health. Centers for Disease Control and Prevention.
spp. Parasites and Parasitological Resources. College of Biological Medicine, Ohio State University.
Smith, James. Malaria. The World of Parasites. McGill University.
Wiser, Mark F. Plasmodium Species Infecting Humans. Tulane University. Last updated 18 May 2004.