Adenovirus-based Gene Therapy: a Promising Novel Cancer Therapy
A Viral Biorealm page on the family Adenovirus-based Gene Therapy: a Promising Novel Cancer Therapy
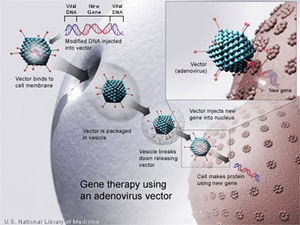
Introduction
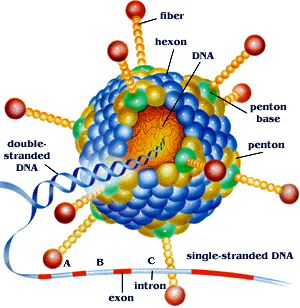
Recent scientific research has established gene therapy as a new and promising approach to traditional therapies in order to combat severe forms of cancer. Existing treatments, such as radiation, require large toxic dosages administered to patients causing a wide-range of long and short-term side-effects for many[1]. The mechanism behind gene delivery is to “correct” and rebuild broken down and infected tissue within the body[1]. Essentially, DNA is used as a "pharmaceutical agent," as it supplements and alters genes within cells[1]. Most effective forms of gene therapy today use DNA that encodes for a therapeutic gene to function in the place of one that is mutated[1]. Another common form involves using DNA that encodes for a protein which serves itself as a drug[1]. Adenovirus (Ad) based cancer gene therapy is a common form of the treatment, as the Ad vector is second to lentivirus vectors in gene based therapies[1]. Treatment has been used as an alternative in clinical trials today and proved to be effective; it is also commonly co-administered with other anti-tumoral drugs, known as "cocktail" drug therapy.
Ad is unique in its viral structure; it is a medium sized (90-100nm), non-enveloped, icosahedral, which includes a nucleocapsid[2]. It is the largest of the non-enveloped viruses, and contains a single double stranded (ds) DNA genome ~36 to 38 kilobases in size[3]. About 57 serotypes exist in humans and cause 5-10% of upper respiratory infections in children; it is also highly prevalent among adults. Ad infects various species of vertebrates and was first found and isolated in human adenoids, pharyngeal tonsil or nasopharyngeal tonsil tissue, in the mid 1950s[2].
Ad is an extremely durable virus, ubiquitous in human and animal populations as it can survive long periods of time out of the host and is endemic year round[4]. Infection has been found in multiple organ systems, yet most remain asymptomatic. Most adults have measurable titers of anti-Ad antibodies, indicating a prior infection or exposure[3]. The virus is oncogenic in rodent infections only[3].
The viral capsid consists of 252 proteins, involving three distinct types: fiber, penton and hexon based proteins (fig 2)[2]. There are 240 hexon proteins (blue) and 12 penton proteins (green). Both fiber and penton base proteins are key in receptor binding and internalization[2], while hexon composes most of the viral capsid. The “spikes” or fibers (yellow) are associated with each penton base protein of the capsid and facilitates in attachment to host cell receptors.
Inhaled droplets cause respiratory infection, while fecal-oral transmission leads to gastrointestinal infection.[4]. There are 3 different types of interactions that can occur upon Ad infection; lytic infection occurs when the Ad enters human epithelial cells and continues through an entire replication cycle, with a host inflammatory response[4]. Chronic or latent infection can also occur, usually infecting the lymphoid tissue, and involves no external symptoms[4]; the exact mechanisms of infection are unknown. The last interaction that can occur is oncogenic within rodents, as an Ad integrates its own DNA into host DNA and produces E1A proteins, altering transcription, deregulating apoptosis and forming malignant tumors[4]. E1A facilitates oncogeny by activation of ras or c-src signaling, polyoma middle T protein[4].
Paradoxically, this role of E1A is not seen in humans, as Ad sequences have not been found in human tumor cells, despite high infection rates in the human population[4].
Ad genome is composed of linear, non-segmented dsDNA, size ranging between 26 and 45 Kbp[5]; the virus can contain up to 22-40 distinct genes[5]. Its genome is significantly larger than that of other viruses within its group, group I, yet it is still considered a relatively "simple virus" and is extremely dependent on host cell for life cycle and replication process[5]. The DNA has a terminal 55 kDa protein within each of its 5' ends of its linear dsDNA which serve as primers in viral replication and ensure that all of the viral genome is replicated[5]. Replication and assembly occurs in the nucleus of infected cells while mature virions leave cell via disintegration[2].
Adenovirus is Key Vector: Benefits and Risk Factors
Ads have been isolated from a large number of different species, 100 serotypes, 57 in humans[4]. Ad is highly effective in gene therapy for its ability to efficiently transduce cells that both dividing and non-dividing[6]. Ad vectors also have capacity to hold large segments of DNA (7.5 kbps); they are easily manipulated with recombinant DNA techniques and have the ability to produce high titers[6]. For most serotypes in Ad, infection is mediated by the binding of the fiber-knob region to a receptor of target cell, known as the coxsackie-Ad receptor (CAR). CAR binding is most commonly observed in serotype 5 in humans, Ad5[7].
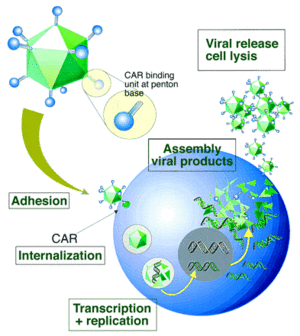
Entry and internalization of the virus is mediated by an interaction between the penton-base Arg-Gly-Asp (RGD) and cellular αv integrins, leading to the endocytosis of the virus through clathrin-coated pits[8]. The αv integrins stimulate actin polymerization which results in the entry of the virus into the endosome. The endosome then acidifies, causing capsid proteins to disassociate. The virus disassembles in the endosome and viral DNA is transported to the nucleus, resulting in expression of viral genes or transgenes[7]. Ad viral DNA is not integrated in the host genome so the risk of mutation is extremely low; however, it remains in an episomal state[8].
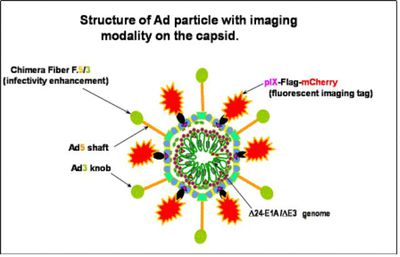
Secondary receptor interactions involving heparan sulfate proteoglycans (HSPGs), have also been reported[7]. Expression of CAR is a rate-limiting factor in Ad infectivity, as expression is highly variable in primary human tumor cells[8]. Reduction of CAR expression correlates with progression of malignant tumors and their state or aggressiveness[7]. CAR is localized within tight junctions, also playing a role in cell adhesion[8].
Life cycle of Ad is separated by DNA replication process in two distinct phases: the early and late[2]. Early genes are responsible for regulatory proteins or proteins which alter host genes for DNA synthesis, activate other viral proteins and avoid premature death of infected cells by host-immune defenses[2]. Early genes can immortalize other cells, for instance, E1A, which binds to Retinoblastoma tumor suppressor, immortalizes primary cells in vitro allowing E1B, which binds to p53 tumor suppressor, to assist in forming tumor cells[2].
Ad vector infection enlists a wide variety of immune responses both humoral and cellular. Responses are due to both Ad vector and already disease-infected cells[8]. Ad presentation activates the immune system and enlists a natural response to combat tumor antigens[8]. However, using Ad as vectors is risky in that too high a dose will result in acute toxicity and autoimmunity[8]. To date, side effects have been mild in Ad therapy, yet non-target side effects are consistently be monitored, both long and short term effects in patients, particularly in the liver[8].
Researchers are currently trying to increase the Ad gene transfer vectors to target tumor cells and decrease any targeting to the liver. There are a number of methods currently being solicited including: Ad fiber pseudotyping, or “tropism modification” through substitution of receptor binding-proteins with those of other serotypes[9]; for example, the substitution of Ad serotype 5 (Ad5) with a knob from Ad serotype 3 (Ad3)[9]. Experimental data with the Ad5/3 chimeras have showed enhanced infectivity in isolated cancer cells without increasing gene delivery and toxicity to murine liver[9].
Gene Therapy
Three regions of the viral genome can accept insertions or substitutions of DNA to generate an independent therapeutic virus vector: a region in E1, E3, and a short region between E4 and end of the genome[10]. In first-generation vectors, the E1 region was removed and replaced with a therapeutic transgene; although vectors were directed to tumor cells, they displayed inhibited viral replication within those cells[10]. Viral transcription of remaining viral genes still occurred, resulting in early innate host cytokine transcription and antigen immune responses[10]. This immune response limited time allotted for gene expression, due to cell-mediated destruction of the transduced cells[10]. Strong immune responses have deemed many studies unsuccessful[10].
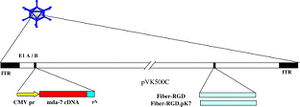
Another serious limitation in the use of these recombinants is difficulty in obtaining efficient gene transfer upon second administration of viral vectors because of neutralizing antibodies[10]. B-cells and T helpers solicited by the major histocompatibility complex (MHC)class II upon presentation of virus vector cannot be fixed by a simple re-design of the vector[10]. Therefore, treatment should only be designated to cases where temporary protein expression is critical in order to stimulate the immune system against cancer or induce apoptotic stimuli, as viral DNA does not integrate within host and eventually disappears[11].
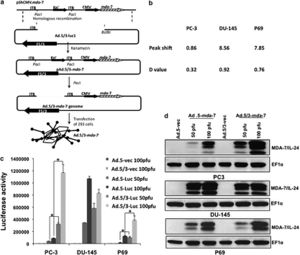
Early trials studied effect of suppression of immune response through naturally co-administration of low-dose etoposide, a cytotoxic agent and anticancer drug, but results were met with limited success[10]. Second and third generation adenoviral vectors have deletions in E1, E2 and E4 genes, since proteins encoded within these genes induce most host immune responses[10]. These new vectors exhibit decreased toxicity and result in prolonged gene expression in in vivo studies.
Interestingly, there are advantages to no integration in host-cell genes within the chromosome which include low disturbances in genes or cellular processes within the body[10]. In pre-clinical trials, vectors have been found to be expressed in the liver, skeletal muscle, heart, brain, lung, pancreas and humor tissues[10]. Administration of Ad intravenously, resulted in virus accumulation in the liver[10]. Treatment close to reproductive organs was designated as safe, as no offspring exhibited germline transmission[10].
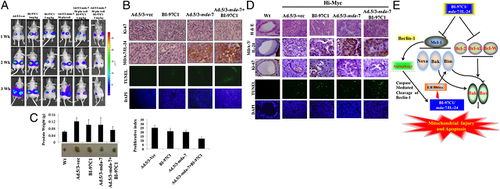
Cancer gene therapy has been most successful in clinical trial involving Melanoma differentiation associated gene-7/interleukin-24 (mda-7/IL-24)[11]. This novel tumor suppressor cytokine is a member of the interleukin-10 (IL-10) gene family[11]. Expression of mda-7/IL-24 using Ad5 vectors has been shown to inhibit growth and induce cell death in many types of cancer cell lines in vitro without harming comparable normal human cells: epithelial, fibrolasts, melanocytes or astrocytes. In addition to apoptosis, it also shows anti-angiogenic, immuno-stimulatory and anti-tumoral activities. Mechanisms of mda-7/IL-24 include ER stress and humoral cell apoptosis by suppression of anti-apoptotic Bcl-2 family members. Additionally, Ad5/3-mda-7/IL-24 has been found to be a better vector compared to Ad5-mda-7/IL-24, in that it is able to deliver gene therapy to a wider range of receptors, not just CAR, which are more specific to tumors. Studies in particular on colorectal cancer cell lines have been conducted on the synergistic effect of the drug Sabutoclax, an Apogossypol derivative Bl-97C1 and Ad5/3-mda-7/IL-24 (Azab et al., 2010)[11]. Bl-97C1 has been found to sensitize cancer cells to mda-7/IL-24, through Mcl-1 and Bcl-2 suppression (Fig 5). Suboptimal doses of Sabutoclax in combination with 50 pfu/cell of Ad5/3-mda-7 were administered to nude mice RKO (expresing 3% surface CARs) and HC116 (expressing 83% surface CARS) carcinoma cell lines. This co-administration resulted in downregulation of pro-survival proteins Bcl-2 and Mcl-1 and induction of apoptosis examined through PARP cleavage[11].
In another study which looks at metastatic potential in prostate cancer cells, in particular two cell lines, DU145, which exhibits moderate metastatic potential and PC3, which exhibits high metastatic potential, researchers found Ad5/3-mda-7/IL-24 to have more of an effect on PC3, opening promising avenues for severe cancer treatment (Eulitt et al., 2010) (Fig 6)[12]. PC3 cells also exhibit low CARs compared to DU145, confirming results found in colorectal cancer cell lines, HC116 and RKO.
Alternative Approaches
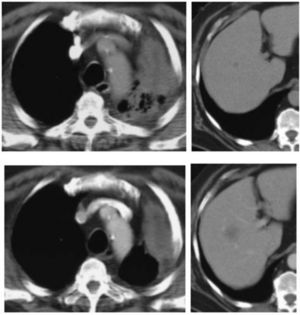
Two strategies have been used to restrict viral replication to target tumor cells and spare normal tissue. The first method involves genetic complementation, with "replication-competent oncolytic agents" or conditionally replicative Ad, CRAds[10]. These CRAds have a mutation in immediate early E1A or E1B Ad genome region, which is complemented in tumor cells and not in normal cells[11]; therefore, location of growth is localized to tumor/tissue specific promoters. There are two known types of CRAds.
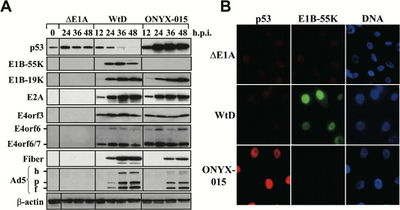
A prototype of type 1 CRAds is Adl1520 or ONYX-015. ONYX-015 contains two mutations in the gene encoding for E1B55k protein and inactivates protein formation[10]. Under normal conditions, E1B55k protein binds and inactivates the tumor-suppressor protein p53 in humans[14]. Tumor cells are most commonly deficient in p53, which would allow Ads with deletion of E1B55k to replicate. This vector works in targeting cancer cells, as it only replicates in cells which do not express p53, more specifically, there is a mutation in the alternate reading frame p14ARF[14]. Results show that nuclear p53 accumulates in ONYX-015 infected cells. Other known effects of p53 are in cell cycle arrest[14]. Primary experiments have shown that in p53- cell lines, C33A and U372, ONYX-015 showed to lyse cells at similar levels to wt Ad virus but was 100-fold less effective at lysing p53+ cell line U87[14]. Another type 1 CRAd, Ad5Δ24 or CB016, involves a mutation of E1A[10]. This use of CRAds removes S phase induction of virus which is not required in proliferating tumor cells or cervical cells transformed by human papillomavirus (HPV) viruses[11].
Type 2 CRAds include a tissue-specific promoter (TSP), for specific target cells[14]. Promoter fragments with specific activity in type 2 CRAds are rare, as structure-function relationship of promoter regions have not been well characterized[14]. TSPs have only been observed empirically, yet hold strong promise for functional therapies[14]. One of the most promising TSPs is for ovarian cancer, secretory leukoprotease inhibitor (SLPI), a 11.7kDa serine protease inhibitor, which is expressed highly in different human carcinomas including breast, lung, endometrium and ovarian cancer[11]. Gene expression analysis has been used to demonstrate that SLPI is 60-fold higher in upregulation in ovarian carcinomas compared to normal ovarian epithelium, and is minimally expressed in normal liver[14].
Chimeric 5/3 fiber modified SLPI CRAd demonstrated a strong anti-tumor effect compared to wild-type Ad in murine ovarian cancer[14].
The promoter, vascular endothelial growth factor (VEGF), has also been found to be effective at targeting molecule for the treatment of non-small cell lung cancer (NSCLC). VEGF is also one of the most important inducers of angiogenesis[11].
The midkine gene serves as a heparin-binding growth factor, and responds to retinoic acid[13]; it has been reported to be over-expressed in a large number of malignant tumors[11]. Midkine promoter has been used successfully for vector gene delivery and CRAd targeting approaches[10].Promoters of CXC chemokine CXCR4 and Survivin, also exhibit an optimal activity and specificity in different tumor types[11]. Genes expressing CXCR4 and Survivin play a major role in progression of metastasis in various tumors[10]. CXCR4 expression is upregulated in breast cancer cells but is not detectable in normal mammal epithelial cells. Survivin is an inhibitor of apoptosis (IAP) and expression levels of Survivin have been shown to be clinical helpful in detection of human gliomas[13]. Survivin is overexpressed in cervical intra-epithelial neoplasias (CINs) and squamous cell carcinomas (SCC) of uterine cervix[13].
Most recently, research has been conducted on highly immunogenic forms of mda-7/IL-24, GST-mda-7, which is delivered to tumors through encapsulation in micro-bubbles[15]. With ultra-sound, these forms of gene therapy can be targeted to cancers[11]. Stem cells also hold high potential to be used as delivery mechanisms of mda-7/IL-24, in brain cancer[14]. There are a wide-variety of approaches and mechanisms to which gene therapy for cancer can occur, all of which are being developed[14]. Phase I trials of mda-7/IL-24 have already proven to be successful in mild cancer therapies[12]. This form of therapy would revolutionize medicine and vastly help in reducing detrimental side-effects of treatments used today in cancer. By using gene therapy as a component in medicine, researchers can not only treat but prevent many forms of cancer from developing and spreading.
References
1) Howe JA, Demers GW, Johnson DE, Neugebauer SE, Perry ST, Vaillancourt MT, Faha B.Evaluation of E1-mutant adenoviruses as conditionally replicating agents for cancer therapy. Mol Ther. 2000 Nov;2(5):485-95.
2) Martin, Malcolm A.; Knipe, David M.; Fields, Bernard N.; Howley, Peter M.; Griffin, Diane; Lamb, Robert (2007). Fields' virology. Philadelphia: Wolters Kluwer Health/Lippincott Williams & Wilkins. pp. 2395.
3) Adenoviral Vectors. http://cmbi.bjmu.edu.cn/cmbidata/therapy/research/re02/002.htm
4) Virology: Adenovirus. MicrobiologyBytes 2010. 14 Nov, 2012 http://www.microbiologybytes.com/virology/Adenoviruses.html
5) Sandra G Gompf, MD, FACP, FIDSA; Chief Editor: Burke A Cunha, MD. Adenoviruses. WebMD LLC. 2012. 14 Nov, 2012. http://emedicine.medscape.com/article/211738-overview
6) Walsh, MP, et al. Computational analysis of two species C human adenoviruses provides evidence of a novel virus. J. Clin. Micobiol. 2011 49:3482-3490.
7) Tuuli Ranki1,2,3,4 and Akseli Hemminki1.Serotype Chimeric Human Adenoviruses for Cancer Gene Therapy Viruses. 2010 October; 2(10): 2196–2212.
8) Gao GP, Yang Y, Wilson JM. Biology of adenovirus vectors with E1 and E4 deletions for liver-directed gene therapy. J Virol. 1996 Dec;70(12):8934-43.
9) Michael Nevels1;Birgitt Täuber1;Thilo Spruss1;Hans Wolf1,Thomas Dobner1. “Hit-and-Run” Transformation by Adenovirus Oncogenes. JVI.75.7.3089-3094.2001 J. Virol. April 2001 vol. 75 no. 7 3089-3094
10) Assessment of Adenoviral Vector Safety and Toxicity: Report of the National Institutes of Health Recombinant DNA Advisory Committee. HUMAN GENE THERAPY 13:3–13 (January 1, 2002),NIH Report. Mary Ann Liebert, Inc.
11) Azab, Belal; Dash, Rupesh; Das, Swadesh . Enhanced Delivery of mda-7IL-24 using a serotype chimeric Adenovirus in Combination with the Apogossypol Derivative Bl-97C1 Sabutoclax Improves Therapeutic Efficacy in Low CAR colorectal Cancer Cells. Journal of Cell Physiology. 2012; 227:2145-2153.
12) Eulitt, Patrick J.;Park, Margaret A.; Hamed, Hossein A.; Cruikshanks, Nichola. Enhancing mda-7/IL-24 therapy in renal carcinoma cells by inhibiting multiple protective signaling pathways using sorafenib and by Ad.5/3 gene delivery. Cancer Biol Ther. 2010; 10(12):1290-1305.
13) D.T. Rein, M. Breidenbach, D.T. Curiel Current developments in adenovirus-based cancer gene therapy. Future Oncol., 2 (2006), pp. 137–143
14) Dent, Paul; Yacoub, Adly; Hamed, Hossein A.; Park, Margaret A. The development of MDA-7/IL-24 as a cancer therapeutic. Pharmacology&Therapeutics. 2010; 128:2:375-84.
15) Frisch, Steven, S. Madhusudan et al. Molecular Targets Article Collection Affymetrix E1A as a Tumor Suppressor Gene
A Multicenter Phase I Gene Therapy Clinical Trial Involving Intraperitoneal Administration of E1A-Lipid Complex in Patients with Recurrent Epithelial Ovarian Cancer Overexpressing HER-2/neu Oncogene. Clin Cancer Res 2004;10:2905–2907.
16) Koski, A.; Pesonen, S.; Cerullo, V.; Escutenaire, S.; Nokisalmi, P.; Raki, M.; Rajecki, M.; Haavisto, E.; Guse, K.; Diaconu, I.; Oksanen, M.; Holm, S.-L.; Laasonen, L.; Partanen, K.; Ugolini, M.; Kangasniemi, L.; Helminen, A.; Karli, E.; Karioja-Kallio, A.; Joensuu, T.; Hemminki, A. Treatment of cancer patients with a serotype 5/3 chimeric oncolytic adenovirus expressing GM- CSF. Hum. Gene Ther. 2009; 11, 1384.
17)Vorburger SA, Hunt KK. Adenovirall gene therapy. Oncologist. 2002;7:46–59.
18) O’Shea Clodagh C.; Johnson, Leisa; Bagus, Bridget; Choi, Serah. Late viral RNA export rather than p53 inactivation, determines ONYX-015 tumor selectivity. Cell. 2004; 6(6):611-23.
Late viral RNA export, rather than p53 inactivation, determines ONYX-015 tumor selectivity.