West Nile Virus in Birds
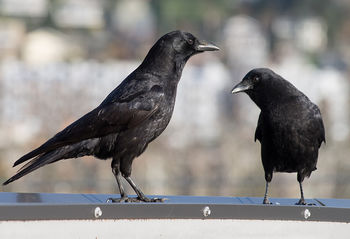
West Nile Virus, of the family Flaviviridae, is a zoonotic disease that infects many species, including humans, throughout the world. Birds are the virus’s primary natural reservoir, where they act as an amplifier host. However, their host competency (their ability to perpetuate the virus' development and spread) and response to infection varies between species; they can have diverging viral loads, viremia, viral shedding, clinical signs, and morbidity. Many factors may affect a particular organism and/or species’ susceptibility and response to infection, including their immune-response capabilities, previous immunities, breeding systems, genetics, geographic spread, and stress levels. Overall, birds vary widely in how they are affected by West Nile Virus, and further surveillance and research into WNV infections in birds is necessary to develop additional knowledge and maintain preparedness for future outbreaks.
Initial Infection
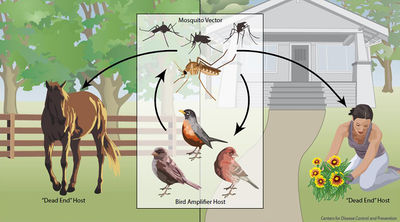
Birds may become infected with West Nile Virus in multiple ways. The most common occurrence is through a mosquito vector [21]. However, studies have shown that they may contract the infection through ingestion of infected organisms or direct contact with infected materials.
Insect Vectors
Over 40 species of mosquitoes have been found to carry West Nile Virus. The most important for spread is the genus Culex, which mainly feed on birds [1], [21]. If a mosquito feeds off an infected host with high enough viral load (approximately one million virus particles per milliliter of blood), it will take up the virus into its gut. The virus will then multiply and spread to the rest of the mosquito’s body, including its salivary glands [1]. The vector may then feed off another animal and transmit the virus to a new host.
Other blood sucking insects, such as ticks, have been found to be able to transmit the virus, though they are not as important for overall spread and outbreaks [1].
Oral Transmission
Birds may become infected by West Nile Virus through consuming infected prey items such as insects, small mammals, and other birds. After ingesting infected organisms, viremia usually occurs identically to that of mosquito-borne transmission [5]. This may influence incidence of infection in carnivorous birds, such as raptors and insectivores.
Contact Transmission
Research has found that it is possible for birds to transmit the virus to one another as a result of emitting particles in oral or cloacal secretions [6]. These secretions, such as feces, may contaminate water and food, or may directly contact another susceptible organism. In captivity this process is rare, and it is unknown how common it occurs in nature. It is hypothesized that this contact transmission route is a factor in spread of the virus in communal roosting populations and during breeding seasons [2], [6].
Species Susceptibility and Competence
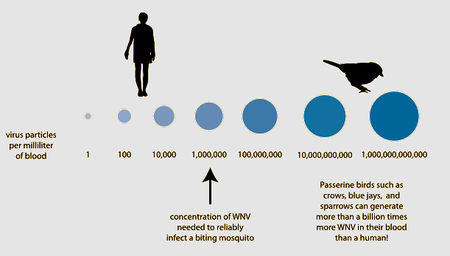
Table of West Nile Virus host competency of 23 species of birds. A larger index number correlates to higher amounts of viral load in concurrence with long durations of viremia. Data adapted from Komar et al. 2003 [2]. | |
Species | Reservoir Competence Index |
---|---|
Blue Jay | 2.55 |
Common Grackle | 2.04 |
House Finch | 1.76 |
American Crow | 1.62 |
House Sparrow | 1.59 |
Ring-billed Gull | 1.26 |
Black-billed Magpie | 1.08 |
American Robin | 1.08 |
Red-winged Blackbird | 0.99 |
American Kestrel | 0.93 |
Great Horned Owl | 0.88 |
Killdeer | 0.87 |
Fish Crow | 0.73 |
Mallard | 0.48 |
European Starling | 0.22 |
Mourning Dove | 0.19 |
Northern Flicker | 0.06 |
Canada Goose | 0.03 |
Rock Dove | 0 |
American Coot | 0 |
Ring-necked Pheasant | 0 |
Monk Parakeet | 0 |
Most mammals, including horses and humans, are “dead-end” hosts for West Nile Virus, as, after infection, the virus does not highly concentrate enough in their blood to then be spread to insect vectors. Many bird species, however, are important “amplification-hosts” for the spread of West Nile Virus, as the virus multiplies to exorbitant numbers within them. Some species can generate the highest levels of virus particles in their blood presently known for any viral infection; they can have millions of times more viral particles in their blood than similarly infected mammals [1].
Over 300 species of birds have been found infected with West Nile Virus [1]. Passerine birds (of the order Passeriformes, such as flycatchers, robins, wrens, mockingbirds, and finches), charadiiform birds (of the order Charadriiformes, such as gulls, plovers, and stilts), and many raptors have been found to be competent hosts for viral infection and spread. In particular, Corvids (species of the family Corviade, including crows, ravens, magpies, and jays), as well as a few other sensitive species, such as American Kestrels and Great Horned Owls, are competent and highly susceptible. Birds of the orders Anseriformes (ducks, geese), Columbiformes (pigeons), Galliformes (chickens, turkeys), Gruiformes (rails, coots), Piciformes (woodpeckers, toucans), and Psittaciformes (parrots) are generally less competent [2]. The competency levels of selected species of experimentally infected birds are shown in the table to the left. These numbers were calculated using an equation that accounted for susceptibility (the proportion of birds that actually became infected after being experimentally exposed to the virus through oral or vector transmission), the bird's average daily infectiousness (the proportion of exposed vector mosquitoes that became infectious per day when feeding off these experimental birds), and duration of infectiousness (the number of days that the bird had high enough viral load to continue spread, determined from analyzing blood samples) [2]. Thus, birds with greater index numbers show higher amounts of viral load as well as long durations of viremia, and are therefore better hosts for spreading infection. Species with low index numbers are relatively unaffected or resistant to the virus.
Occurrence of mortality due to infection increases with species competence. In early studies of WNV in the United States, up to 100% mortality was observed in American Crows (Corvus brachyrhynchos) and Blue Jays (Cyanocitta cristata) experimentally inoculated with the virus. In these susceptible species, large amounts of virus are widely distributed in major organs, causing multi-organ failure and inducing a rapid death that does not allow the development of clinical signs [6]. It is suspected that WNV infection may require underlying illnesses or immune suppression in particular species to result in death [2]. Many symptoms, such as neurological issues that impede flight and coordination, may lead to subsequent issues in survival that increase mortality in the wild [5].
It has been found that some species, particularly Corvids such as American Crows, may contain a high enough viremia level that they remain reservoir competent postmortem; they are able to transmit viral particles to vector hosts that feed on their remains up to 5 days after death [1], [7].
Many birds that contract West Nile Virus do not die. Certain immune responses, such as antiviral mechanisms, may limit viral infection and viremic spread. Birds with low susceptibility may present minor symptoms and recover, or may appear healthy with low-grade, non-progressive infection. These birds may then have successful immune-mediated clearance of infectious particles [5]. Some species may also maintain low levels of viral replication that lead to chronic infections [8].
Infection Symptoms
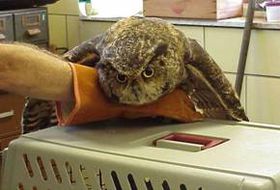
The majority of individual birds infected with West Nile Virus do not get sick or show symptoms. However, if they do become sick, clinical signs may include uncoordinated walking, lethargy, ataxia, weakness, tremors, inability to fly, anorexia followed by rapid weight loss, green waste products, blindness, lack of awareness, head droop, and abnormal body posture [3], [8], [23]. They may die within 24 to 48 hours of showing symptoms [3]. In the final phase of infection preceding death, birds may have severe tremors or seizures. These symptoms reflect virus infection and inflammation of the brain and spinal cord [3], [8].
Through bird necropsies, organs found to be most frequently infected with virus particles are the spleen, kidney, skin, and eye [5]. Usually, the virus first appears in the spleen, then rapidly spreads to other organs, and reaches the central nervous system later. Tissue distribution occurs earlier in more susceptible species. Histologic lesions, such as mild to severe encephalitis, necrosis, inflammation, and hemorrhages, have been found in multiple tissues, including the brain, spinal cord, eye, peripheral nervous system, heart, spleen, liver, kidney, lung, gastrointestinal tract, endocrine system, gonads, skeletal muscle, skin, and bone marrow. The speed of viral distribution and host death may affect the development of lesions, as hosts with higher susceptibility may die before lesions become observable. Macroscopic changes due to infection include emaciation, dehydration, multi-organ hemorrhages, and congestion [5], [8].
Issues with movement and flight in wild birds, as opposed to internal issues, leads more frequently to human notice, interaction, and intervention. Thus, neurological symptoms are very commonly identified symptoms of the virus. And although they are very frequently infected by WNV, Corvids rarely present neurological symptoms, as the virus spreads so quickly that the bird dies before presenting most problems; many die from lesions of their internal organs before the viral particles reach the brain or spinal cord [8]. Less susceptible birds, such as owls and hawks, frequently live long enough to show brain and spinal cord lesions, and thus more commonly present neurological issues [5].
Host Factors
There is little evidence about why bird species are affected by West Nile Virus differently. Some speculation and study points to life-style variations, such as breeding systems and geographic spread, which lead to unique collections of pathogen defense components for each species. Other differences may arise from genetics, such as intrinsic capabilities to combat viral infection.
Geography
Phylogenetically related, geographically divergent species have been shown to be differently affected by West Nile Virus infection [10]. Thus, their geographic spread is thought to influence their susceptibility. It is believed that this is through climate differences influencing the acquisition of cross-reactive immunologic protection to West Nile Virus. Sometimes, when a bird is infected with one kind of virus, it will gain immune protection against other related viruses. Warmer habitats support greater vector populations as well as better conditions for viral propagation, so southern-living species, where the climate is warmer, are more frequently exposed to mosquito born Flaviviridae infections, and therefore may develop better resistance to WNV [10].
There is some speculation that differences in species susceptibility may be related to co-evolution of the virus with particular species. In the Old-World (Europe, Africa, Asia), West Nile virus is mostly a benign infection in birds, possibly due to thousands of years of evolutionary selection for birds that produce antibodies and therefore survive. Generally, Old-World birds (such as Barn Owls, Tyto alba, native to Europe) appear to be less susceptible to WNV than New-world birds (such as Red-tailed Hawks, Buteo jamaicensis and Great Horned Owls, Bubo virginianus, native to the Americas) [5]. It wasn't until 1999 that the virus first appeared in North America. Therefore, it may be that because Old-World birds have been in contact with the virus for longer, they have evolved better immune responses to it [11], [12].
Breeding Systems
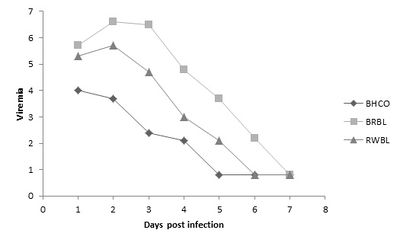
Taxonomically similar species may diverge in viral susceptibility due to their type of breeding system. In particular, it has been found that parasitic breeding may increase resistance to WNV, as they present lower viremia levels [13]. Brood parasite bird species, such as Brown-Headed Cowbirds (Molothrus ater), may develop a robust immune system and innate disease response. As shown in the figure to the right, the Brown-headed Cowbird, as compared to related species, consistently presents the lowest and fastest decreasing viremia when experimentally infected with WNV by mosquito vectors [13]. This is because they are in close contact with varying host bird species, and thus experience infestation with a greater diversity of vector species than do other songbirds [13]. Therefore, this life-style evidently influences their susceptibility to WNV.
Because it is possible for infection to spread through direct contact between birds, species that that have collective breeding patterns, or those that have group roosting, feeding, or migration stopovers sites, as well as other similar behaviors that increase group densities, may be more prone to spreading infection to one another [2], [24]. Spread may also increase through mosquito vectors because of the proximity and availability of hosts in these situations. These factors may lead to an increase in notable effect on these kinds of species' populations.
Genetics
Few studies look at the genetic basis for species' host capabilities for WNV. However, there has recently been research into a potential genetic basis of WNV resistance in particular bird hosts. One gene, 2’-5’ oligoadenylate synthetase 1b (Oas1b), codes for an enzyme that was previously found in mice to play a part in WNV resistance, and has been found in chickens to show similar oligoadenylate synthetase activity [14]. This chicken OAS-L enzyme, when expressed in mammalian cells, has antiviral activity against WNV. Chickens, compared to many other bird species, are relatively resistant to WNV infection. This gene may be responsible for their particular immune abilities to fight the virus [14].
Because Corvids, a related group of birds, are collectively more susceptible to infection, it is clear that they have a similar reason for increased detrimental effect. Their immune systems react slowly to initial infection, which may be caused by genetic factors. This slow immune reaction allows the virus to spread through their organs quickly and cause severe damage. This is in opposition to birds with lower susceptibility, which show lower speed of viremia spread, and thus have a better chance of fighting viral load increase or damage to vital organs.
Effect on Populations
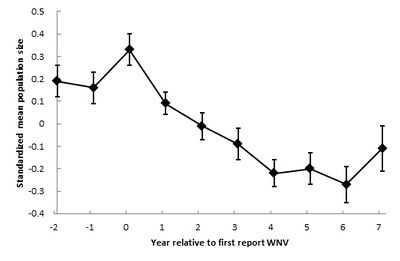
Individual animals vary in their responses to WNV infection; in the wild, birds are frequently found with WNV antibody prevalence, suggesting previous WNV infection and subsequent survival. American Crows, which experimentally show a very high mortality rate (upwards of 100%), have been found with antibodies to the virus [17]. However, the virus generally effects species in trends of greater or lesser affect, as discussed above. Thus, effects due to WNV to species total and regional populations have been studied since the virus was first detected in the United States in 1999.
Crows and "Dilution Effect"
Within four months of West Nile Virus's initial introduction, approximately 5,500 crows died of infection. By 2001, the virus spread to bird populations in many eastern states. By 2002 it spread to most of the mid-west as well as California, and by 2004 had been detected in every state of the continental US, excluding Oregon [15].
Crow populations have been the most looked at and analyzed for how they have been effected by the viral outbreak, and changes to their populations have been studied over time. One analysis of American Crow populations describes that initially populations declined rapidly, then lessened in decline, and are presently normalizing, as displayed in the figure to the left. Thus, West Nile virus seemingly became less virulent as it moved westward across North America [15]. The researchers discuss that the virus potentially evolved as it spread. As an ssRNA virus, West Nile presents a large amount of genetic variation. The viral strains that killed their hosts too quickly could not be effectively spread through mosquito vectors, nor moved regionally through migratory flight patterns, and therefore the only strains that were able to be spread westward were those to be lower in lethality. This characteristic of a lower level of lethality of the virus may explain the lessening of population decline observed today [16].
These researchers also found that crows in diverse habitats were less likely to become infected with the disease than crows in species-poor areas. They explain the decrease in infection and population decline through the idea of a "dilution effect." Areas of higher diversity in bird populations were less affected by the virus, because it was less likely for a vector mosquito to bite a very susceptible bird; they were more likely to infect "dead-end" host species [15], [16].
Some studies which have compared the effects of West Nile Virus on different species have found evidence for possible changes in species' ranges, potentially due to deaths of another species' population caused by WNV infection. Fish Crows, which show lower mortality rates to WNV than do American Crows, are normally found in small regions near coasts. Researchers found that some populations seem to have begun growing their ranges, moving inland [17]. They hypothesize that the increase in Fish Crow range may be a result of the decrease in American Crow populations from WNV mortality. The Fish Crows may be taking the open niches that American Crow populations have left as their numbers have decreased. More research should be pursued into this area, as it may represent ecological effects and disturbances [17].
2012 Eagle Deaths
In late 2012, over two dozen Bald Eagles (Haliaeetus leucocephalus) succumbed to West Nile Virus infection in Utah [18]. Their deaths caused lots of press coverage because of the irregularity of their deaths, as well as their position as a symbol of the country. The birds were being found laying listless on the ground, many suffering from head tremors, seizures, and paralysis in the legs, feet, and wings. Because this occurred in the winter, it is suspected that mosquitoes were not responsible for the virus's spread. Researchers believe that the eagles may have contracted WNV from eating dead grebes. Grebes are prevalent in large numbers in the area in the winter months, and may have been affected by an outbreak of WNV. The deaths of these Bald Eagles will most likely not affect the greater population of Bald Eagles in the United States [18], [25].
Endangered Species
There is concern that endangered species may be at risk of further population damage from a WNV outbreak. Such threatened species include the Florida Scrub-Jay (Aphelocoma coerulescens), California Condor (Gymnogyps californianus), and Whooping Crane (Grus americana) [12]. Since 1999, no dramatic changes to endangered bird species has been shown to be a result of WNV infection. However, because the virus is still prevalent across the country, there is a continuing possibility of future outbreaks [1], [12]. If changes do occur, organizations are in place to detect them and respond accordingly; currently, surveillance on endangered species populations is largely in place, and tracking of WNV spread is in progress as well (see below section "Future Studies and Surveillance").
Surveillance and the Future of Infection
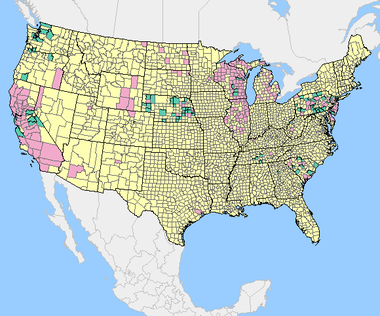
Even though the effects and spread of West Nile virus have recently declined/stagnated [15], it is necessity to continue research and surveillance of this disease. Some support includes the fact that the disease is still frequently detected and that it has been identified at least once in every state [1]. Furthermore, the evidence that West Nile Virus had adaptively evolved to lower lethality to encourage spread [15] illustrates its potential for recurrence and more dangerous outbreaks. Another change in viral strain may occur, as it is clear that the genetic variation of the virus allows it to have adaptable abilities.
To further study the spread and effect of WNV, surveillance systems have been put into place by the CDC and USGS (United States Geological Survey) [19]. Many states, including California, Pennsylvania, Illinois, Ohio, and many others encourage their residents to report dead or sick birds to governmental services; each state has a website easily found online where residents can contact and provide information to officials. The USGS tracks and reports WNV in US counties by obtaining data regarding arboviral cases through an agreement with the Centers for Disease Control and Prevention (CDC), and this information is used to gain further knowledge about disease spread. They generally use sentinel chickens for their research [19]. The map of the United States to the right is a recently produced and frequently modified figure available online to give information to the public about where WNV is currently tested for and being found.
Currently, a commercial West Nile Virus vaccine exists for horses [9], while no vaccine for humans or birds exists for common use [21]. Research has been going into developing bird vaccines, and some advancements have been established. Several zoos provide vaccinations to their birds with intramuscular commercially available inactivated whole virus vaccine, and have found significant differences in antibody titers in particular species, including American flamingos (Phoenicopterus ruber) and black-footed penguins (Spheniscus demersus) [20]. Further studies have been done to test the use of other types of WNV vaccines in varying bird species. One research group injected DNA vaccinations against WNV into Red-Tailed Hawks (Buteo jamaicensis) and found that vaccinated birds presented seroconversion. They had less severe viremia with shorter, lower-intensity shedding periods than did non-vaccinated animals. The vaccine did not completely eliminate illness, but successfully weakened the effects of infection [22]. More research is warranted to find better and stronger vaccines against WNV for birds.
In caring for sick animals found by the public, wildlife rehabilitation centers frequently come across birds with West Nile Virus infections. These organizations keep track of birds they deem as potentially West Nile positive. However, testing to confirm infection is expensive. Normally, determination of disease is concluded based on symptoms; sometimes oral samples are collected, but only after the presentation of symptoms or death [23]. Thus, many non-symptomatic cases may be left unnoticed. Numerous birds that die in the wild may also go undetected. Thus, further academic research and testing of birds is necessary to continue advancing knowledge about the virus and developing preparedness against outbreaks.
Further Reading
West Nile Virus— Centers for Disease Control and Prevention
West Nile Virus— Wikipedia
California Department of Health— California WNV Tracking and Reporting Website
References
1. Greene, S. E., and A. Reid. 2013. FAQ: West Nile Virus. American Society For Microbiology.
2. Komar, N., S. Langevin, S. Hinten, N. Nemeth, E. Edwards, D. Hettler, B. Davis, R. Bowen, and M. Bunning. 2003. Experimental Infection of North American Birds with the New York 1999 Strain of West Nile Virus. Emerging Infectious Diseases 9(3): 311-322.
3. "West Nile Virus." Seattle Audubon Society. Web. 9 Mar. 2014. <http://www.seattleaudubon.org/sas/LearnAboutBirds/SeasonalFacts/WestNileVirus.aspx>.
4. Taylar, I. Crows. 2012. Seattle, WA. flickr. Web. 9 Mar. 2014. <http://www.flickr.com/photos/taylar/8516674909/>.
5. Nemeth, N., D. Gould, R. Bowen, and N. Komar. 2006. Natural And Experimental West Nile Virus Infection In Five Raptor Species. Journal of Wildlife Diseases 42(1): 1-13.
6. Kipp A.M., J.A. Lehman, R.A. Bowen, P.E. Fox, M.R. Stephens, K. Klenk, N. Komar, and M.L. Bunning. 2006. West Nile virus quantification in feces of experimentally infected American and fish crows. American Journal of Tropical Medicine and Hygine 75(4): 688-690.
7. McLean, R.G. 2004. West Nile Virus: Impact on Crow Populations in the United States. USDA National Wildlife Research
Center - Staff Publications 367. 9 Mar. 2014. <http://digitalcommons.unl.edu/icwdm_usdanwrc/367>.
8. Gamino, V., and U. Höfle. 2013. Pathology and tissue tropism of natural West Nile virus infection in birds: a review. Veterinary Research 44(1): 39.
9. Frequently Asked Questions about West Nile Virus and Wildlife. USGS National Wildlife Health Center. Web. 9 Mar. 2014. <http://www.nwhc.usgs.gov/disease_information/west_nile_virus/frequently_asked_questions.jsp>.
10. Lopes, H., P. Redig, A. Glaser, A. Armien, and A. Wünschmann. 2007. Clinical Findings, Lesions, and Viral Antigen Distribution in Great Gray Owls (Strix nebulosa) and Barred Owls (Strix varia) with Spontaneous West Nile Virus Infection. Avian Diseases 51(1): 140-145.
11. Ludwig, G.V., P.P. Calle, J.A. Mangiafico, B.L. Raphael, D.K Danner, J.A. Hile, T.L. Clippinger, J.F. Smith, R.A. Cook, T. McNamara. 2002. An outbreak of West Nile virus in a New York City captive wildlife population. American Journal of Tropical Medicine and Hygine 67(1): 67-75.
12. Chu, M., W. Stone, K.J. McGowan, A.A. Dhondt, W.M. Hochachka, and J.E. Therrien. 2003. West Nile File. Cornell Lab of Ornithology, Birdscope 17(1). Web. 11 Mar. 2014. <http://www.birds.cornell.edu/Publications/Birdscope/Winter2003/West_Nile_File.html>.
13. Reisen, W.K., and D.C. Hahn. 2007. Comparison of Immune Responses of Brown-Headed Cowbird and Related Blackbirds to West Nile and Other Mosquito-Borne Encephalitis Viruses. Journal of Wildlife Diseases 43(3): 439-449.
14. Tag-El-Din-Hassan H.T., N. Sasaki, K. Moritoh, D. Torigoe, A. Maeda, and T. Agui. 2012. The chicken 2’-5’ oligoadenylate synthetase A inhibits replication of West Nile Virus. Japan Journal of Veterinary Research 60(2-3): 95-103.
15. Koenig, W.D., W.M. Hochachka, B. Zuckerberg, and J.L. Dickinson. 2010. Ecological determinants of American crow mortality due to West Nile virus during its North American sweep. Oecologia 163(4): 903-909.
16. Powell, H. 2014. All About Birds - Counting Crows - West Nile virus effect on American Crows. Cornell Lab of Ornithology, Web. 13 Mar. 2014. <http://www.allaboutbirds.org/Page.aspx?pid=1956>.
17. Wilcox, B.R., M.J. Yabsley, A.E. Ellis, D.E. Stallknecht, and S.E.J. Gibbs. 2007. West Nile Virus Antibody Prevalence in American Crows (Corvus brachyrhynchos) and Fish Crows (Corvus ossifragus) in Georgia, U.S.A. Avian Diseases 51(1): 125-128.
18. Glionna, J.M. 2014. West Nile virus blamed for rash of bald eagle deaths in Utah. Los Angeles Times, 3 Jan. sec. Nation Now. Print.
19. West Nile Virus Maps - Bird - USA. United States Geological Survey. U.S. Department of the Interior. Web. 11 Mar. 2014. <http://diseasemaps.usgs.gov/wnv_us_bird.html>.
20. Okeson, D.M., S.Y. Llizo, C.L. Miller, and A.L. Glaser. 2007. Antibody Response Of Five Bird Species After Vaccination With A Killed West Nile Virus Vaccine. Journal of Zoo and Wildlife Medicine 38(2): 240-244.
21. West Nile Virus. 2014. Centers for Disease Control and Prevention. Web. 24 Mar. 2014. <http://www.cdc.gov/westnile/index.html>.
22. Redig P.T., T.N. Tully, B.W. Ritchie, A.F. Roy, M.A. Baudena, and G.J. Chang. 2011. Effect of West Nile virus DNA-plasmid vaccination on response to live virus challenge in red-tailed hawks (Buteo jamaicensis). American Journal of Veterinary Research 72(8): 1065-1070.
23. DNR - West Nile Virus (WNV). Michigan Department of Natural Resources. Web. 10 Mar. 2014. <http://www.michigan.gov/dnr/0,4570,7-153-10370_12150_12220-99070--,00.html>.
24. Pérez-Ramírez, E., M.A. Jiménez-Clavero, and F. Llorente. 2014. Experimental Infections of Wild Birds with West Nile Virus. Viruses 13(2): 752-781.
25. Soaring deaths of bald eagles in Utah attributed to West Nile virus. 2013. NBC News, Dec 31. Web. 1 Apr. 2014. <http://usnews.nbcnews.com/_news/2013/12/31/22125743-soaring-deaths-of-bald-eagles-in-utah-attributed-to-west-nile-virus?lite>.
Edited by Leah Pomerantz, a student of Nora Sullivan in BIOL168L (Microbiology) in The Keck Science Department of the Claremont Colleges Spring 2014.