Bacillus thuringiensis toxin in C. elegans
By Sarah Adrianowycz
Bacillus thuringiensis as an organism
Bacillus thuringiensis is prevalent in the soil, and is a Gram–positive, spore-forming member of the Bacillus ceres group. The Bacillus ceres group is a collection of seven genetically similar species, three of which are so alike that they are occasionally considered the same organism, and would be if not for their vastly different pathogenic outcomes (Kho et al. 2011). It is these different pathogens, or external expression of the organism’s phenotype, which are often used by researchers to determine which species are distinct. In the case of B. thuringiensis the organism is typified by the production of specific invertebrate related toxins (Ceuppens et al., 2013). However, the issue with using phenotypic differences to differentiate among these organisms is that the differences frequently stem from virulence factors contained on plasmids, which are extremely variable and transient. These genetic elements separate from the primary genome can be exchanged through horizontal gene transmission, lost in the environment, or selected against during culturing in the laboratory. Despite the issues with distinguishing strains based upon their ability to cause disease, B. thuringiensis is often distinguished by the ability to form crystal proteins. These proteins are insecticidal in nature and referred to as cry genes for the genes that code their expression (Ceuppens et al.). In the wild, most strains of B. thuringiensis are capable of producing anywhere from 1-4 types of Cry proteins (Kho et al., 2011).The ability to produce multiple cry genes may be explained because of the synergistic effect that some of these proteins have in taking over a host when working together, or because different potential hosts have different levels of susceptibility to specific Cry proteins (E. Schnephf, 1998).
It is the ability to produce these Cry proteins that gives B. thuringiensis value as an insecticide and has made it especially interesting to humans who are looking into the proteins' biological applications. These toxins have been utilized both in direct application on to crops and as a source of transgenic elements that provide resistance against the orders Lepidoptera, Diptera, and Coleoptera (E. Schnepf, 1998) when incorporated into the genomes of genetically modified organisms. The method of action within this subset of proteins makes them especially effective against insects but has yet to produce conclusive evidence of negative effects against either humans or other higher trophic level organisms, as is typically the case for insecticides. Because of these perceived benefits and the lack of obvious repercussions, the utilization of plants with Cry proteins has become common place since 1996, when genetically modified potatoes, corn, and potatoes with versions of cry genes were made commercially available (Schnepf).
Cry Genes in Bacillus thuringiensis
The genome of B. thuringiensis has between 2.4 – 5.7 million base pairs and is supplemented extensively by plasmids, both linear and circular in nature. The largest of these additional genetic elements are responsible for the encoding of the crystals released during sporulation (Schnepf).
Cry genes are expressed during the stationary phase of B. thuringiensis’s life cycle, and to such an extensive degree that 20-30% of the dry weight of the organism’s spore is made up of the resulting crystals. The regulation of crystal formation and its relationship with the stationary phase are monitored at three levels of regulation: transcriptional, posttranscriptional, and posttranslational, but not all cry genes are dependent upon the process of spore formation (Schnepf). The geometric structure of the resulting crystals differs based upon the type of Cry protein being expressed, ranging from bipyramidal to spherical, although certain Cry proteins require accessory proteins to assemble into their correct crystal structure (Schnepf).
The crystals produced by cry genes are made up of potential toxins that become active only when consumed by a susceptible organism. Susceptible organisms are those that have alkaline conditions in a portion of their digestive system, as is the case for the midgut of insects and the intestines of nematodes. Once the crystal has entered the alkali conditions, the toxin begins to work after being made soluble, cleaving by enzymes involved in protein catabolism, and then being inserted into the membrane of the epithelium where it is able to form pores (Schnepf). The success of various Cry proteins can sometimes be explained by differences in solubility, and decreasing solubility has been suggested as a reason for increasing insect resistance to the toxin. Once processing is completed, Cry proteins are capable of two main functions, the ability to bind to specific receptors on the target organism and to function as an ion channel. Two methods of pore function have been suggested, one where the pore allows non-specific ion transport and another where the controlled transport of specific ions results in undermining of the membrane potential that is necessary for the cell’s normal functioning (Schnepf). The reason that there is debate over the selectivity of the pore is because of the wide range of experimental conditions in testing Cry proteins has yielded conflicting results. But this inconsistency may be evidence of the pore responding to differing environmental conditions instead of improper scientific experimentation (Schnepf).
There have been recent attempts to systematically alter the structure of the Cry proteins in order to improve their functioning from the point of view of those trying to increase the toxin’s insecticidal nature. Although decreasing solubility is the evolutionary means suggested by which insects decrease their susceptibility to the toxins, human efforts have been focused on increasing the amount of irreversible binding, a process typically associated with insertion of the toxin into the epithelial cells of the organism, in order to create more lethal varieties of Cry proteins (Schnepf).
The Relationship between B. thuringiensis Cry Proteins and Nematodes
B. thuringiensis is widespread in the soil and allocates a large amount of energy and resources to the production of Cry proteins that need to be consumed to be activated, despite the fact that most of the insects its Cry proteins have been researched to be effective against spend minimal time feeding directly from the soil (Wei et al., 2003).
In contrast, nematodes, specifically Caenorhabditis elegans, inhabit the soil under typical conditions and are a compelling model organism because not only are they likely to encounter Cry proteins and other pathogens in their natural habitat, but they represent one of the over 100,000 species of nematodes that directly consume soil and B. thuringiensis (Wei et al., 2003). This similarity of habitat and the frequent interaction among these organisms means C. elegans may be one of the intended targets for Cry proteins. Nematodes have a number of responses to deal with environmental threats, among them immune responses, although these are costly for the organism. Another more rapid and less energetically and metabolically expensive option is supplementation by behavioral changes to adapt in a rapidly changing environment to newly encountered threats (Luo et al.,2013).
Although the impact of Cry proteins has been widely studied in insects that harm agricultural products and directly influence humans, less research has been done on the nematode model. It is important to determine the impact of these toxins on C. elegans to answer the question of by what method these proteins are toxic, how the two organisms might have coevolved, and the potential for larger environmental impacts if this stable soil inhabitant is negatively impacted by something that has found widespread application in industrial agriculture (Wei et al., 2003). Because of the inclusion of cry genes within staple food crops, nematodes, including C. elegans, have had increasing contact with this pathogen in what were previously stable environments that contained the Cry proteins as a result of B. thuringiensis but at levels lower than currently.
Structure of Cry5B
There are over 200 types of cry proteins that have so far been identified, and they range in genetic similarity from less than 20% to more than 90% (Hui et al., 2012). However, Cry5B is the most intensively researched cry protein with negative impacts on nematodes, and this toxin functions through binding to specific glycolipid receptors in the worm’s intestines (Hui et al. 2012). Although the sequence similarity between Cry5B and the cry proteins that are most effective against insects is limited to approximately 20%, the proteins share a similar three component structure. The toxins that are effective in insects that have so far been sequenced have a high degree of structural similarity, but the average difference between Cry5B and this group is even greater than for the most dissimilar insecticidal toxin, Cry2Aa (Hui et al., 2012).
The first domain of Cry5B is believed to be the active mechanism for pore formation, and because of this vital functional role it has the largest degree of similarity to other cry proteins which are all believed to function through pore formation (Hui et al., 2012). Although there is only 22% sequence similarity, once the complex is activated by the proteases in the nematode intestine the activated toxin becomes similar to that of Cry4B (Hui et al., 2012). The first domain is made up of five helixes grouped together with one focal helix that serves as initiator for pore formation. The central helix has been identified in all cry proteins that have so far been structurally investigated (Hui et al., 2012).
Domain two is the most structurally independent of the three components of Cry5B, and has greater structural similarity to a banana lectin than to other cry proteins because of the homodimer structure. Because of this structural difference from other cry proteins but the similarity to a manose binding structure, Cry5B is able to accomplish glycan receptor binding that the other cry proteins are not (Hui et al., 2012).
Domain three is conserved among cry proteins and all of the cry proteins structurally analyzed show marked similarity to molecules capable of binding carbohydrates in this domain, among which some of the mechanisms are known and well studied (Hui et al., 2012).
Impact of Cry5B on Caenorhabditis elegans
Cry5B, when produced by E. coli and fed directly to C. elegans results in the death of the nematodes after approximately six days at a lethal dose of approximately 8µg/ml because of cry protein intoxication, and no infection by the bacteria. In contrast, when Cry5B is provided for nematodes at a similar concentration but in the presence of B. thuringiensis death results in 24-48 hours as the bacteria take control, resulting in what the authors refer to as a “Bob” phenotype, or Bag of bacteria, where the internal organs of the nematode are digested and replaced by a combination of bacterial cells and spores surrounded by the cuticle of C. elegans (Kho et al., 2011). Bacterial infection requires both the presence of this pore forming protein (PFP) and the bacterial source, although it does not matter if the cry protein is produced by the bacteria or just available for uptake in the environment. C. elegans cultured in the presence of B. thuringiensis without Cry5B does not result in infection. The “Bob” phenotype can be seen in the accompanying image, Figure 1, as represented in panel B where the infected nematodes are completely linear, devoid of any internal structures, and lacking their typical color. (Kho et al., 2011).

A subsequent experiment determined that the Cry5B protein is only able to cause death in C. elegans when binding occurs between the toxin and a subset of receptors in the organism’s intestinal track. In mutant nematodes that were devoid of glycolipid receptors, the toxin was incapable of forming a pore, and as a result B. thuringiensis was unable to cause an infection, supporting the hypothesis that the establishment of the pore is a vital virulence factor that permits infection by B. thuringiensis (Kho et al., 2011).
Although C. elegans death is almost certain within 48 hours of exposure due to takeover by B. thuringiensis, it can take as few as fifteen minutes of exposure to the cry protein and the pathogenic bacteria for a high level of death to be observed, and there is no statistical difference in the percentage of C. elegans death for any time points tested between 15 minutes and 8 hours of exposure, even when organisms were subsequently washed of the excess cry protein and the bacteria (Kho et al., 2011).
Means of resistance to Cry5B in Caenorhabditis elegans
In order to understand the means through which some C. elegans individuals are able to remain resistant to cry proteins , Huffman et al. selected for mutants that were not killed in the presence of Cry5B and determined that surviving nematodes were defective in bre-1 through bre-5 functioning (2004). Bre (an abbreviation for Br-toxin resistant) mutants are unable to integrate the pore forming protein Cry5B into the epithelium of the intestine, possibly because the bre genes encode a receptor that permits the uptake of Cry5B, or possibly because the genes influence the level of binding that occurs in the nematode’s intestine. Either way, the result is limited binding of the protein and limited pore formation (Huffman et al., 2004).
Impact of Cry6A on Caenorhabditis elegans
Although there has been extensive study of the method of Cry5B’s toxicity in nematodes, until the work of Luo et al., there had been little research into the impact of the structurally distinct cry protein Cry6A. Cry6A is a significant departure from other related toxins because it is genetically different, lacking the five conserved sequences that are present in other identified B. thuringiensis cry proteins. The dramatic genetic difference suggests that Cry6A might act though a mechanism distinct from that previously described for Cry5B, as genetic differences are hypothesized to cause structural differences although the structure of this protein has not yet been crystallized (Luo et al., 2013).
Cry6A severely negatively impacts the typical functioning of C. elegans, impinging growth, stunting brood size, altering movement, and resulting in behavioral changes in an attempt to limit exposure and ingestion of the toxin. Luo et al. found the media lethal dose of Cry6A to be 18.499 μg/mL, a value on par with the previously published value for Cry5B, which speaks to the novel cry protein’s toxicity in C. elegans. Upon determining this value, the authors sought the sub-lethal impacts of Cry6A and discovered that larvae raised in serially diluted Cry6Aa2 were significantly smaller than those raised in control buffer, with the growth reduced by fifty percent at 6.345 μg/mL, as depicted in figure 2 (Luo et al.)
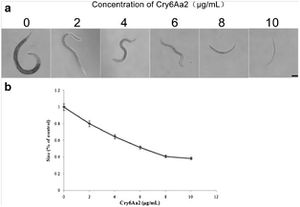
The authors also discovered that C. elegans exhibits a number of behavioral adaptations in an attempt to minimize its exposure to this toxin when able to so. Among these is the decreasing of ingestion rate with increasing toxin concentration, as measured by contraction of the pharynx grinder over the course of 30 seconds. Measured decreases were substantial, from
41.6 to 27.2 times at a concentration of 21.25 μg/mL, and a reduction to 9.9 times at 340 μg/mL of toxin addition (Luo et al.). An interesting component of this experiment was that results were measured for both 1 and 6 total hours of exposure, and the overall rate of consumption was higher at every concentration for the longer time interval (Luo et al.). A possible reason for this increase could be that the organism is only capable of evading a toxin using this method alone for a limited amount of time and eventually is forced to return to the baseline level of consumption to sustain itself, even when toxins are present.
Another study sought to determine the susceptibility of numerous nematode species to a range of cry proteins, including those from both the Cry5 and Cry6 subfamilies. The Cry5 subfamily is made up of six toxins, all of which have four of the five gene sequences present in other studied B. thuringiensis proteins. This genetic similarity indicates not only their linked ancestry, but their relatedness to Cry1A, Cry3A, and Cry4A which are significant insecticides utilized by industrial agriculture (Wie et al.). In contrast, the Cry6 subfamily is made of two proteins which have marked similarity to one another but lack the five conserved sequences the other proteins share (Wie et al.). This group’s findings support the hypothesis that cry toxins work through the intestines of nematodes, showing marked similarity to how the toxins function in insects. The researchers found correlation between the results of the plate assay, where organisms were fed cry toxins produced by plated E. coli, and the altered appearance of the impacted organism’s intestine. When organisms were found dead or intoxicated as a result of the specific cry protein, they were also discovered to have severely impacted digestive systems. Some of the intestinal changes as a result of the toxin were smaller intestinal cells, pulling in of the intestine away from the rest of the body, and general disturbed appearance as can be seen in figure 3 (Wie et al.).The gut morphology of C. elegans was negatively impacted by all of the toxins tested, among which was Cry6A.

Genetic response of Caenorhabditis elegans to Bacillus thuringiensis
When C. elegans is exposed to a culture of entirely B. thuringiensis DB27 the nematode dies within a day, in contrast to nematode survival on three other pathogens on which the organism can subsist anywhere from 2-5 days (Sinha et al., 2012). C. elegans exhibits pathogen and species specific levels of gene regulation in response to B. thuringiensis DB27, and measurement at the four hour time point is long enough to observe a substantial transcriptional response (Sinha et al.). At the simplest level, the amount of gene expression is greatest for the bacteria that pose the greatest threat to the host organism, with C. elegans having a genetic response in 5688 genes, 97% of which mark an up regulation of gene expression, in contrast to the 249 total genes impacted by exposure to S. aureus (Sinha et al.). Sinha et al. also found that the genomic response of C. elegans to B. thuringiensis DB27 was distinct from the genomic response to another gram-positive bacterium, S. aureus, with altered expression appearing only for B. thuringiensis for 97 genes.
Some of the gene varieties with increased expression after exposure to B. thuringiensis are those that increase the number of proteins with lipid processing abilities and those with similar structures, as these lipases frequently are part of C. elegans’s response to pathogens (Sinha et al., 2012). There is also increased gene expression that is non-specific to B. thuringiensis and that is conserved among bacterial threats and includes genes involved with both protein break down and ATPase functioning (Sinha et al.)
Conclusion
The importance of studying the impact of Bacillus thuringiensis and its toxins on native soil communities should not be underestimated. C. elegans is one of the many organisms necessary for aeration and proper functioning of the soil, and changes in toxin concentration, intentional or unintentional, deserve proper research.
References
Ceuppens, Siele, Nico Boon, and Mieke Uyttendaele. "Diversity of Bacillus cereus group strains is reflected in their broad range of pathogenicity and diverse ecological lifestyles." FEMS microbiology ecology 84.3 (2013): 433-450.
Huffman, Danielle L., et al. "Pore worms: Using< i> Caenorhabditis elegans to study how bacterial toxins interact with their target host." International Journal of Medical Microbiology 293.7 (2004): 599-607.
Hui, Fan, et al. "Structure and glycolipid binding properties of the nematicidal protein Cry5B." Biochemistry 51.49 (2012): 9911-9921.
Kho, Melanie F., et al. "The pore-forming protein Cry5B elicits the pathogenicity of Bacillus sp. against Caenorhabditis elegans." PloS one 6.12 (2011): e29122.
Luo, Hui, et al. "The effects of Bacillus thuringiensis Cry6A on the survival, growth, reproduction, locomotion, and behavioral response of Caenorhabditis elegans." Applied microbiology and biotechnology 97.23 (2013): 10135-10142.
Schnepf, E., et al. "Bacillus thuringiensis and its pesticidal crystal proteins." Microbiology and molecular biology reviews 62.3 (1998): 775-806.
Sinha, Amit, et al. "System wide analysis of the evolution of innate immunity in the nematode model species Caenorhabditis elegans and Pristionchus pacificus." PloS one 7.9 (2012): e44255.
Wei, Jun-Zhi, et al. "Bacillus thuringiensis crystal proteins that target nematodes." Proceedings of the National Academy of Sciences 100.5 (2003): 2760-2765.