Clostridium botulinum Neurotoxin: The Duality of a Microbe with Fatal and Therapeutic Applications: Difference between revisions
No edit summary |
No edit summary |
||
Line 43: | Line 43: | ||
Spores are the most resistant cell type, and can survive in extremely adverse conditions, such as extreme heat, desiccation, starvation, chemicals, and radiation.<ref name=Dürre>[https://doi.org/10.1128/microbiolspec.tbs-0010-2012 Dürre P. (2014) “Physiology and Sporulation in Clostridium,” Microbiology Spectrum. ASM Journals. 2(4)]</ref> Under stress conditions, the cell initiates spore formation by either activating or repressing the gene expression of sporulation regulators. As a result, a vegetative cell enters an asymmetrical septation phase, forming a mother cell and a forespore. The mother cell engulfs the forespore, providing protective layers for the maturing spore.<ref name=Munir></ref> Finally, the mature spore is released by lysing the mother cell, and this dormant spore can later undergo germination to begin producing botulinum toxin.<br> | Spores are the most resistant cell type, and can survive in extremely adverse conditions, such as extreme heat, desiccation, starvation, chemicals, and radiation.<ref name=Dürre>[https://doi.org/10.1128/microbiolspec.tbs-0010-2012 Dürre P. (2014) “Physiology and Sporulation in Clostridium,” Microbiology Spectrum. ASM Journals. 2(4)]</ref> Under stress conditions, the cell initiates spore formation by either activating or repressing the gene expression of sporulation regulators. As a result, a vegetative cell enters an asymmetrical septation phase, forming a mother cell and a forespore. The mother cell engulfs the forespore, providing protective layers for the maturing spore.<ref name=Munir></ref> Finally, the mature spore is released by lysing the mother cell, and this dormant spore can later undergo germination to begin producing botulinum toxin.<br> | ||
<br> | <br> | ||
Bacterial endospores contain several integument layers, one of which is the peptidoglycan spore cortex, which is essential for conserving spore dormancy and heat resistance. Spore germination is the first stage of a dormant spore becoming a vegetative cell, which is the normal growing cell type that produces the <i>C. botulinum</i> neurotoxins.<ref name=Broussolle></ref> Spore germination is initiated by a germinant receptor which responds to nutrient germinants. The <i>C. botulinum</i> germinant receptor is typically composed of three proteins, GerA, GerB, and GerC, whose encoding genes make up a multi-gene locus.<ref name=Brunt></ref> The germinant receptor of <i>C. botulinum</i> spores is usually induced by germinants such as amino acids or sugars. In one study, most strains were shown to germinate in the presence of L-lactate and L-alanine, but germination was also found to be strain, temperature, pH, and buffer dependent.<ref name=Brunt></ref> Once germination is initiated, the spore releases dipicolinic acid and various ions, such as Ca2+, which leads to hydrolysis of the cortex peptidoglycan. Then, cortex-lytic enzymes degrade the spore cortex, which allows for core hydration and expansion to occur, taking the spore out of its dormant state.<ref name=Brunt></ref> Cell metabolism and outgrowth recommences once germination is complete and the spore is no longer dormant. The mechanism of spore germination differs slightly between <i>C. botulinum</i> groups, with groups I and III displaying the same mechanism, which is a different mechanism than of groups II and IV. | Bacterial endospores contain several integument layers, one of which is the peptidoglycan spore cortex, which is essential for conserving spore dormancy and heat resistance. Spore germination is the first stage of a dormant spore becoming a vegetative cell, which is the normal growing cell type that produces the <i>C. botulinum</i> neurotoxins.<ref name=Broussolle></ref> Spore germination is initiated by a germinant receptor which responds to nutrient germinants. The <i>C. botulinum</i> germinant receptor is typically composed of three proteins, GerA, GerB, and GerC, whose encoding genes make up a multi-gene locus.<ref name=Brunt></ref> The germinant receptor of <i>C. botulinum</i> spores is usually induced by germinants such as amino acids or sugars. In one study, most strains were shown to germinate in the presence of L-lactate and L-alanine, but germination was also found to be strain, temperature, pH, and buffer dependent.<ref name=Brunt></ref> Once germination is initiated, the spore releases dipicolinic acid and various ions, such as Ca2+, which leads to hydrolysis of the cortex peptidoglycan. Then, cortex-lytic enzymes degrade the spore cortex, which allows for core hydration and expansion to occur, taking the spore out of its dormant state.<ref name=Brunt></ref> Cell metabolism and outgrowth recommences once germination is complete and the spore is no longer dormant. The mechanism of spore germination differs slightly between <i>C. botulinum</i> groups, with groups I and III displaying the same mechanism, which is a different mechanism than of groups II and IV.<br> | ||
<br> | |||
<b>Mechanism of Toxicity</b> | <b>Mechanism of Toxicity</b> | ||
<br> | <br> |
Revision as of 20:39, 15 April 2024
Introduction
By Juliette Leclerc
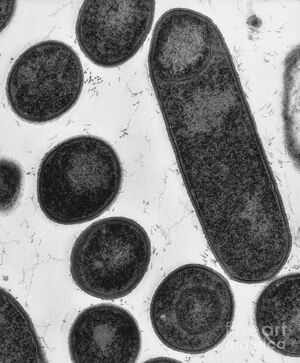
Clostridium botulinum is a gram-positive, rod-shaped, anaerobic, and spore-forming bacterium.[1] C. botulinum is made up of four different groups (I-IV), related by their collective ability to produce the neurotoxin botulinum.[2] Botulinum toxin has seven serotypes (A-G), most of which cause botulism, a neuroparalytic disease that can be fatal. There are several types of botulism, including food-borne, wound, infant, and iatrogenic botulism.[3] Clostridium botulinum is an extremely biologically diverse microorganism, and can exist in a variety of different environments and conditions, especially due its highly heat resistant spores, which create the botulinum toxin. Botulinum neurotoxin is the most potent natural toxin known to science, and despite even very small doses being fatal, it also has several therapeutic applications. Its most common application is in the cosmetics industry, as botulinum toxin is the active ingredient in Botox®.[4] Overall, Clostridium botulinum is a truly unique microbe that has an extraordinary range of applications in our world.
Early History of Botulinum Toxin
The first appearance of botulism in written history begins with the acknowledgment of food poisoning during the Byzantine era, over a millennium ago.[5] Food-borne botulism, and therefore the Clostridium botulinum toxin, is thought to have accompanied humans since we first began storing and preserving food.[6] There are few sources available documenting cases of botulism prior to the 19th century, as the connection between food and death by paralysis had not yet been realized or established. The first to really delve into understanding food poisoning and food-borne botulism was Justinus Kerner (1786-1862), a medical officer in a small town in Germany.[6] During the Napoleonic wars, the sanitation of rural food production experienced a severe decline due to widespread poverty, which led to several documented outbreaks and cases of paralytic and fatal food poisoning, specifically relating to the consumption of blood sausage, a delicacy of the time.[5] Kerner ran numerous experiments on animals and himself to investigate the symptoms and onset of what he termed “sausage poison,” and eventually published the very first case study on botulism. He even suggested possible therapeutic applications of the toxin, which have since been proven to be a reality.
The bacteria that produces the botulinum toxin was first isolated in 1895 by Emile Pierre-Marie van Ermengem following the death of 3 musicians at an event with a meal featuring smoked and pickled ham.[5] Ermengem, a microbiologist, performed autopsies on the bodies, as well as a detailed analysis of the ham itself. He originally named the bacterium Bacillus botulinum, inspired by the latin word for sausage, “botulus.” [6]
Following its initial identification, a Dr. G. Landmann discovered in 1904 another bacterial toxin that caused botulism, this time isolated from a bean salad; the first of its kind to be found in a non-meat product. This bacterium was found to be distinct from the original toxin identified by Ermengem, which had been nonproteolytic, while this new one was in fact proteolytic.[7] This discovery marked the first identification of botulinum toxin serotypes, which we now know to be made up of types A-G, each immunologically distinct neurotoxins.[8] This new finding debuted a key notion: that different toxins may be produced by the same bacterial strains, just as the same toxin can be produced by different strains.[7] The proposal to change the genus name occurred in the 1920s, in order to separate the aerobic Bacillus species from the anaerobic Clostridium species, officially giving Clostridium botulinum its current name.[9]
Cell Biology
Cell Structure and Biology
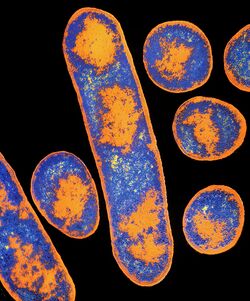
Clostridium botulinum is a gram-positive, rod-shaped, anaerobic, and spore-forming bacterium.[1] Clostridium botulinum is an extremely diverse species. In fact, it is not a homogeneous species, but rather a collection of four distinct phenotypes/bacterial groups, labeled groups I-IV, all of which share the commonality of producing the botulinum neurotoxin.[2] Groups I and II both cause botulism in humans, but group I is proteolytic, while group II is non-proteolytic.[10]
These two groups were the two discovered by Emile Ermengem and Dr. Landmann when C. botulinum was first isolated and identified.[7] Group III causes animal botulism, and group IV does not cause botulism.[11]
In fact, studies have shown that the four groups’ lack of a phylogenetic relationship means that they could rightfully be considered four separate species.[2]
In addition to the four phenotypes of the C. botulinum species, there are seven confirmed serotypes of the botulinum neurotoxin, named types A-G, and each display structural and functional differences. All seven serotypes’ molecular mass is 150 kDa, and all of their genomes have been sequenced; the deviation in their amino acid sequences was found to range between 37.2% and 69.6%.[10] In fact, within each serotype (A-G), several subtypes have been identified (approximately 40 in total), a further display of the extremely vast diversity of the Clostridium botulinum species.[2]
Group I produces types A, B, and F, and group II forms neurotoxins of type B, E, and F, so the serotypes C, D, and G do not typically affect humans. Clostridium botulinum’s genome has been fully sequenced, revealing that it is made up of one chromosome and one small plasmid encoding a bacteriocin. The genomes of Group I and II were highly similar, and both had low G-C content (28.2% and 27.5% respectively).[10]
Peptidoglycan is a major component of the cell wall of gram-positive bacteria. Peptidoglycan is a large polysaccharide macromolecule that helps bacterial cells maintain their rigidity and shape. The major amino acids and amino sugars that make up the C. botulinum cell wall peptidoglycan are glutamic acid, alanine, diaminopimelic acid, glucosamine, and muramic acid.[12]
Some gram-positive bacteria have what is called a surface layer, which surrounds the peptidoglycan cell wall and acts as a protective sheath. It was found that certain C. botulinum cells contain an S-layer, and research suggests that the S-layer serves to hinder phage lysins from lysing the cell, acting as a kind of protection mechanism.[13]
Sporulation and Germination

Sporulation in Clostridium botulinum is a survival and pathogenesis strategy.[14] Proteolytic C. botulinum is responsible for food-borne, wound, and infant botulism, and more specifically, the oval-shaped spores that induce botulism when they infect or are ingested by the human body.[15]
Spores are the most resistant cell type, and can survive in extremely adverse conditions, such as extreme heat, desiccation, starvation, chemicals, and radiation.[16] Under stress conditions, the cell initiates spore formation by either activating or repressing the gene expression of sporulation regulators. As a result, a vegetative cell enters an asymmetrical septation phase, forming a mother cell and a forespore. The mother cell engulfs the forespore, providing protective layers for the maturing spore.[14] Finally, the mature spore is released by lysing the mother cell, and this dormant spore can later undergo germination to begin producing botulinum toxin.
Bacterial endospores contain several integument layers, one of which is the peptidoglycan spore cortex, which is essential for conserving spore dormancy and heat resistance. Spore germination is the first stage of a dormant spore becoming a vegetative cell, which is the normal growing cell type that produces the C. botulinum neurotoxins.[15] Spore germination is initiated by a germinant receptor which responds to nutrient germinants. The C. botulinum germinant receptor is typically composed of three proteins, GerA, GerB, and GerC, whose encoding genes make up a multi-gene locus.[2] The germinant receptor of C. botulinum spores is usually induced by germinants such as amino acids or sugars. In one study, most strains were shown to germinate in the presence of L-lactate and L-alanine, but germination was also found to be strain, temperature, pH, and buffer dependent.[2] Once germination is initiated, the spore releases dipicolinic acid and various ions, such as Ca2+, which leads to hydrolysis of the cortex peptidoglycan. Then, cortex-lytic enzymes degrade the spore cortex, which allows for core hydration and expansion to occur, taking the spore out of its dormant state.[2] Cell metabolism and outgrowth recommences once germination is complete and the spore is no longer dormant. The mechanism of spore germination differs slightly between C. botulinum groups, with groups I and III displaying the same mechanism, which is a different mechanism than of groups II and IV.
Mechanism of Toxicity
Clostridium botulinum produces seven distinct neurotoxins, serotypes A-G. [8] The neurotoxins produced by C. botulinum are the most potent naturally occurring toxic substances known to man, with just 30-100 nanograms being potentially fatal.[17] The neurotoxins act primarily at peripheral synapses, and inhibit the release of the neurotransmitter acetylcholine. One study carried out on rabbits and mice showed that the botulinum toxin binding sites are localized on the presynaptic membranes, which are at the end of axons, where the action potential gets converted to a chemical signal to trigger neurotransmitter release.[18]
At neuromuscular junctions, intracellular calcium ions signal the induction of acetylcholine release. The botulinum toxin is thought to reduce the influx of Ca2+ ions into the nerve terminal. The toxin also reduces the neurotransmitter release system’s affinity for calcium ions, and though the neurotransmitter terminals remain structurally functional, this serves to decrease and inhibit the release of neurotransmitters.[19]Botulinum neurotoxins form complexes with accessory proteins, like hemagglutinin, which help protect it and aid it in becoming absorbed by the body. In their most active forms, the toxins exist as dichain molecules, with a heavy chain and a light chain linked by a disulfide bond.[10] The heavy chains have two functional domains, the C- and N-terminal domains.[10] The C-terminal domain is involved in selectively and irreversibly binding the neurotoxin to receptors at presynaptic surface, or motor end plate, of cholinergic nerves, while the N-terminal moves the light chain into the cytoplasm of the nerve cell, where the disulfide bond is cleaved and the toxin is released into the cytoplasm.[4] The light chains are zinc endopeptidases that become active within the nerve cell and cleave proteins to destabilize the neurotransmitter-vesicle fusion complex.[10] When the complex is destabilized, the calcium-mediated release of the neurotransmitter cannot occur correctly.[20]
The blocking of acetylcholine, which is essential for proper muscle contraction, leads to the muscle weakness and paralysis that are key features of botulism disease.
Botulism disease
Types and Manifestation of Botulism

Clostridium botulinum toxin causes botulism, a rare, but potentially fatal neuroparalytic disease. The majority of the symptoms of botulism are caused by the muscle paralysis induced by the toxin, and include difficulty swallowing, muscle weakness, drooping eyelids, double or blurry vision, slurred speech, difficulty breathing, vomiting and nausea, as well as stomach pain and diarrhea, and when not rapidly treated, can lead to death.[3] The symptoms of botulism often make hospitalization necessary, as the first symptom of blurred vision is followed by acute symmetrical descending bilateral paralysis, and eventual paralysis of the respiratory and cardiac muscles.[2] If caught early, an antitoxin can be administered that stops the circulation of the toxin in the bloodstream, but even so, recovery can take several weeks. In certain cases, even with administration of the antitoxin, patients die from respiratory failure, as the muscles that control breathing become paralyzed. Patients who do survive may have long term shortness of breath and fatigue, potentially requiring years of therapy.[21]
Nowadays, only about 5 to 10% of botulism cases are fatal, but prior to the development of the antitoxin, about 50 in 100 people who contracted botulism would die.[3]
There are several known types of botulism: food-borne, wound, infant, and iatrogenic botulism. Food-borne botulism can be contracted by eating improperly preserved, canned, or fermented foods.[3] Clostridium botulinum thrives in anaerobic conditions, and produces its fatal toxin in low oxygen environments, such as in home-canned foods. The spores that C. botulinum produces are able to survive in adverse conditions that would normally kill other cells, making them a prominent food safety hazard.[22]
Symptoms of food-borne botulism typically begin occurring within 12 to 36 hours after consumption of contaminated food.[21] The second type of botulism is wound botulism, which can occur if the bacterial spores enter a wound, where they begin producing the toxin. Wound botulism is most common in intravenous drug users, such as black tar heroin users, as well as in wounds resulting from surgery or traumatic injuries.[3] The third type is infant botulism, which babies can get when bacterial spores enter their intestinal tract and produce toxins. Infants’ immune systems are not developed enough to stop the germination of the spores, as they lack the gastrointestinal acidity and bacterial flora diversity of more mature children, making infants under 12 months the most susceptible and vulnerable.[23] This is why raw honey should not be fed to children under the age of one, as it can contain C. botulinum spores, which can be very dangerous to their health.[24] In the United States, there are approximately 100 cases of infant botulism a year, and 20% of them can be linked to the consumption of raw honey. In fact, infant botulism is the most common type of botulism, making up 70% of all new cases a year.[23] In certain rare cases, adults can also contract intestinal botulism, known as adult intestinal toxemia, especially adults with preexisting conditions that already negatively impact their gut health and microbiome.[3] Lastly, the fourth type of botulism is iatrogenic botulism, which is a man-made form of botulism caused by injecting too much botulinum toxin for cosmetic, medical, or therapeutic reasons. Following injection, the toxin is taken up into the bloodstream and dispersed throughout the body, resulting in botulism, with about 20% of iatrogenic cases requiring antitoxin. [25]
Disease Prevention
The Clostridium botulinum toxin is dangerous, especially since it can be contracted in a multitude of ways. Another aspect of the microbe that makes it particularly hazardous is its ability to grow in many entirely different environments, and at several extreme environmental conditions. Clostridium botulinum’s optimal growth temperature is around 30°C, and its minimum growth temperature is 3.0°C.[10] Its optimal pH is 6.9, and it cannot grow below a pH of 5.6. Its spores are highly heat resistant, and one study showed that proteolytic, or Group I spores, are able to withstand heat shock of 75°C, but other C. botulinum spores can even resist at much higher temperatures.[26]
In fact, C. botulinum spores are some of the most heat resistant of all pathogenic organisms, making their inactivation the standard and reference point for commercial sterilization.[14] C. botulinum’s extreme resistance to environmental conditions makes it very difficult to get rid of spores within food products, and biological and chemical treatments are not very effective, often just presenting secondary food safety hazards. For example, nitrite is a chemical additive that has been used to cure meats against vegetative C. botulinum, but it was found to be carcinogenic, making the food unsafe in a different way.[14] Overall, thermal treatment remains the primary method of controlling C. botulinum hazards in food. Thermal treatment consists of exposing C. botulinum to temperatures beyond a certain threshold, resulting in denaturation and the loss of enzymatic functions. Thermal treatment, essentially high temperature pasteurization, ensures commercial sterility and helps preserve the nutritional and sensory properties of the food products.[14] Irradiation has also been found to be a successful method of achieving commercial sterility, but availability of the technology and customer concerns present limitations on its widespread use in food treatment.[14]
Therapeutic Applications of Botulinum Toxin
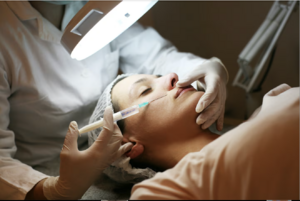
Botulinum toxin blocks the release of acetylcholine at the neuromuscular junction, which sparked interest in its use as treatment for overactive smooth muscle and abnormal gland activity. It was first demonstrated as an effective management for strabismus, and now is used to treat several disorders of spasticity, as well as in almost every subspecialty of medicine.[4] As of now, serotype A is the only toxin type available for commercial clinical use, but types B,C, and F will potentially emerge as future therapeutic agents.[27] Two preparations of toxin serotype A exist: Dysport® and Botox®. It has been shown that Botox® is three times more potent than Dysport®. Botox® was approved by the FDA in 1989 for the treatment of strabismus, blepharospasm, and hemifacial spasms, and it received FDA approval again in 2002 for the cosmetic treatment of the temporary reduction of glabellar forehead frown lines.[4] The peak effect of the toxin occurs after four to seven days after injection, and while it does not damage the nerve terminals, the blocking of acetylcholine is irreversible. After two to three months, new nerve terminals and synaptic contacts form, and the injected area recovers its normal function.[4] Each vial of Botox® contains, in a sterile, vacuum-dried form without a preservative: 100 Units of Clostridium botulinum type A neurotoxin complex, 0.5 milligrams of human Albumin, and 0.9 milligrams of sodium chloride.[4] In a 100-unit vial, only 4.8 nanograms of neurotoxin are needed; as little as 30-100 nanograms is a fatal dose.[22] Botox® is one of the most common non-surgical cosmetic procedures, with an estimated 11 million people having used it.[28]
In addition to its use as a cosmetic, botulinum neurotoxin can also be used as a treatment for pain. Most pain mediations are either inadequate or have deleterious side effects, such as addiction. Some natural pain remedies have been investigated, such as venom from cone snails or spiders.[29]
Botulinum toxin is also a kind of natural pain reliever. The neurotoxin acts in two ways: either by directly blocking the contractile activity of the muscle or by stopping the release of neurotransmitters, other than acetylcholine, that are involved in the stimulation of muscle nociceptors, otherwise known as pain receptors. Botulinum toxin has also been shown to be effective against neuropathic pain, or pain affecting the nerves that carry sensory information to the brain.[29] Despite being one of the most potent and fatal toxins known to science, botulinum neurotoxin also has cosmetic, medical, and therapeutic applications that are beneficial to humankind.
Conclusion
Clostridium botulinum is a diverse organism; in terms of both its biology and its functions and applications in the world. The species is made up of four distinct groups, related by their collective ability to produce the botulinum neurotoxin, the most potent natural substance known to science, of which there are seven different serotypes. C. botulinum is also a spore producing microorganism, whose spores are extremely heat resistant, allowing them to survive in a multitude of environments and conditions. When spores are absorbed by the human body, they cause a neuroparalytic disease called botulism, which if not treated rapidly, can be fatal. The four types of botulism are food-borne, wound, infant, and iatrogenic botulism. Iatrogenic botulism is caused by the excessive injection of botox, which is a cosmetic application of C. botulinum. Apart from being used as a cosmetic, C. botulinum has several other clinical applications, such as for pain treatment. Overall, Clostridium botulinum is a unique and complex microorganism that has both harmful and beneficial functions for the human world, but continues to be studied, as there remains a lot more to learn and discover.
References
- ↑ 1.0 1.1 Johnson, E.A., Bradshaw, M., (2001) “Clostridium botulinum and its neurotoxins: a metabolic and cellular perspective,” Toxicon, 39(11), 1703-1722.
- ↑ 2.0 2.1 2.2 2.3 2.4 2.5 2.6 2.7 [10.3389/fmicb.2016.01702 Brunt J., van Vliet A.H.M., van den Bos F., Carter A.T., Peck M.W. (2016) “Diversity of the Germination Apparatus in Clostridium botulinum Groups I, II, III, and IV,” Frontiers in Microbiology, 7.]
- ↑ 3.0 3.1 3.2 3.3 3.4 3.5 Centers for Disease Control and Prevention. June 8, 2022. Botulism. U.S. Department of Health and Human Services.
- ↑ 4.0 4.1 4.2 4.3 4.4 4.5 Nigam, P. K., & Nigam, A. (2010) “Botulinum toxin,” Indian journal of dermatology, 55(1), 8–14.
- ↑ 5.0 5.1 5.2 Whitcup, S.M. (2019) “The History of Botulinum Toxins in Medicine: A Thousand Year Journey,” Botulinum Toxin Therapy, Handbook of Experimental Pharmacology, 263.
- ↑ 6.0 6.1 6.2 Erbguth, F.J. (2004) “Historical notes on botulism, Clostridium botulinum, botulinum toxin, and the idea of the therapeutic use of the toxin.” Mov. Disord., 19, S2-S6.
- ↑ 7.0 7.1 7.2 Smith, T.J., Hill, K.K., Raphael, B.H. (2015) “Historical and current perspectives on Clostridium botulinum diversity,” Research in Microbiology, 166(4), 290-302.
- ↑ 8.0 8.1 Oguma, K., Fujinaga, Y., Inoue, K., (1994) “Structure and Function of Clostridium botulinum Toxins,” Department of Bacteriology, Okayama University Medical School, 39(3), 161-168.
- ↑ Winslow, C. E., Broadhurst, J., Buchanan, R. E., Krumwiede, C., Rogers, L. A., & Smith, G. H. (1917). The Families and Genera of the Bacteria: Preliminary Report of the Committee of the Society of American Bacteriologists on Characterization and Classification of Bacterial Types. Journal of bacteriology, 2(5), 505–566.
- ↑ 10.0 10.1 10.2 10.3 10.4 10.5 10.6 Carter, A.T., Peck, M.W. (2015) “Genomes, neurotoxins and biology of Clostridium botulinum Group I and Group II,” Research in Microbiology, 166(4), 303-317.
- ↑ Gregory, K. S., Acharya, K. R. (2023) “A Comprehensive Structural Analysis of Clostridium botulinum Neurotoxin A Cell-Binding Domain from Different Subtypes,” Toxins, 15(2), 92.
- ↑ Takumi, K. and Kawata, T. (1970), Chemical Composition of the Cell Walls of Clostridium botulinum Type A. Japanese Journal of Microbiology, 14: 57-63.
- ↑ Zhang, Z., Douillard, F. P., Korkeala, H., & Lindström, M. (2022). Specific Isolation of Clostridium botulinum Group I Cells by Phage Lysin Cell Wall Binding Domain with the Aid of S-Layer Disruption. International journal of molecular sciences, 23(15), 8391.
- ↑ 14.0 14.1 14.2 14.3 14.4 14.5 Munir, M. T., Mtimet, N., Guillier, L., Meurens, F., Fravalo, P., Federighi, M., & Kooh, P. (2023). Physical Treatments to Control Clostridium botulinum Hazards in Food. Foods (Basel, Switzerland), 12(8), 1580.
- ↑ 15.0 15.1 Broussolle, V., Alberto, F., Shearman, C.A., Mason, D.R., Botella, L., Nguyen-The, C., Peck, M.W., Carlin, F. (2002) “Molecular and Physiological Characterisation of Spore Germination in Clostridium botulinum and C. sporogenes,” Anaerobe, 8(3), 89-100.
- ↑ Dürre P. (2014) “Physiology and Sporulation in Clostridium,” Microbiology Spectrum. ASM Journals. 2(4)
- ↑ Peck, M.W., Stringer, S.C., Carter, A.T. (2011) “Clostridium botulinum in the post-genomic era,” Food Microbiology, 28(2), 183-191.
- ↑ Hirokawa, N. & Kitamura, M. (1979) “Binding of Clostridium Botulinum Neurotoxin to the Presynaptic Membrane in the Central Nervous System,” The Journal of Cell Biology 81(1), 43–49.
- ↑ [10.1113/jphysiol.1976.sp011510 Cull-Candy, S.G., Lundh, H., Thesleff, S. (1976) “Effects of botulinum toxin on neuromuscular transmission in the rat,” The Journal of Physiology, 260.]
- ↑ Hambleton, P. (1992) “Clostridium botulinum toxins: a general review of involvement in disease, structure, mode of action and preparation for clinical use,” J Neurol 239, 16–20.
- ↑ 21.0 21.1 Mayo Clinic Staff, (2022) “Botulism,” MayoClinic.
- ↑ 22.0 22.1 Peck, M.W. (2009) “Biology and Genomic Analysis of Clostridium botulinum,” Advances in Microbial Physiology. Academic Press.Vol. 55, 183-320.
- ↑ 23.0 23.1 Van Horn N.L., Street M. (2024) “Infantile Botulism,” StatPearls.
- ↑ Cagan, E., Peker, E., Dogan, M., & Caksen, H. (2010) “Infant botulism,” The Eurasian journal of medicine, 42(2), 92–94.
- ↑ [10.1097/MS9.0000000000000711 Islam, M., et al. (2023) “The recent outbreak of Iatrogenic botulism: point of view from the present world,” Annals of Medicine & Surgery 85(6), 2289-2290.]
- ↑ Briozzo, J., de Lagarde, E. A., Chirife, J., & Parada, J. L. (1986) “Effect of water activity and pH on growth and toxin production by Clostridium botulinum type G,” Applied and environmental microbiology, 51(4), 844–848.
- ↑ Münchau, A., Bhatia, K.P. (2000) “Regular Review: Uses of Botulinum Toxin Injection in Medicine Today.” BMJ: British Medical Journal, 320(7228), 161–65.
- ↑ University of San Francisco, “Cosmetic Botox,” University of San Francisco Health.
- ↑ 29.0 29.1 Kumar, R. (2018) “Therapeutic use of botulinum toxin in pain treatment,” Neuronal Signal 2(3).
Authored for BIOL 238 Microbiology, taught by Joan Slonczewski,at Kenyon College,2024