Origins of a Homochiral Microbial World
By: Maggie Taylor
Introduction
How did life begin? Where do we come from? What were the first organisms on earth? Questions like these have long puzzled even the brightest of scientists, and answers are only just beginning to surface. Historically, questions about the origins of life and evolution have evaded conversation. In societies heavily reliant on religion and faith, scientific hypotheses about the origins of life is usually disapproved and waved to the side. In 1925, John Scopes, a biology teacher, was even arrested for teaching evolution in a classroom. Those days are long gone, however, and today, society has started to use a more scientific analysis of the origins of life on Earth.

Microbes are the oldest life forms that have been identified today. Their simplicity, size, and metabolic diversity makes them ideal candidates for life in a world without oxygen, in a world under constant attack from meteorites, and in a world where no other life forms exist. Stromatolites dating back 3.4 billion years depict communities of cyanobacteria in a tidal pool in the Bahamas. Microfossils depict early microbial cells decayed by time. Biosignatures found in sedimentary rock show signs of organic molecules formed only by microbes. The question now sought after asks how these microbes even came to be. Though microbial cells are simpler than multi-cellular, complex, eukaryotic cells, life is by no means “easy.” Enzymes, arguably the most important feature of life, are specific and highly regulated. They have evolved to be exact, even perfect. How can a world of "nothing" evolve to perfection?
In 1848, Louis Pasteur demonstrated a novel way that molecules and crystals can become even more specific without differing in molecular weight and chemical make-up. Pasteur identified two types of crystals of a solution and determined that they were the same in every way except that they were mirror images of each other. He then separated these two crystals and made solutions of each, deeming one “+” and one “-“. By shining polarized light through each solution, Pasteur then discovered that the two solutions had equal but opposite optical activity. In other words, the direction of polarized light passing through these molecules was in opposite directions, one spinning left (L, levo), and one spinning right (D, dextro). When the two solutions were combined, now known as a racemic mixture, the solution demonstrated no optical activity. The two types of crystals, mirror images of each other, are today known as enantiomers.
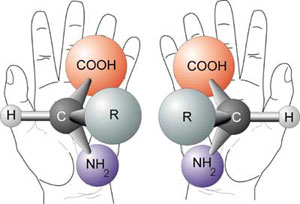
Since Pasteur’s discovery of enantiomers, there has been much research in the specific orientation of atoms around organic carbon. Today it is generally understood that all living organisms contain sugars and amino acids of only one enantiomeric form. Thus, organisms are called “homochiral.” Homochirality is one of the most remarkable features of living organisms. In fact, it is critical to the formation of life. Enzymes act on only one enantiomer of a sugar molecule. Proteins themselves are homochiral; amino acids in living organisms are (L)-enantiomers. The positioning of atoms in non-random, specific orientations, however, is actually quite difficult to do in a laboratory, especially without the aid of chiral enzymes. Kinetically, molecules prefer to isomerize in water, driving a homochiral system toward a racemic system. The prebiotic environment, if not covered by ice, would have been characterized by large waves of water that would have diluted prebiotic chiral molecules, resulting in a racemic composition of amino acids and sugars. How then did the prebiotic world select for a homochiral world? How do (L)-amino acids arise? From where did these homochiral molecules originally come? How did they survive the harsh prebiotic soup that the earth was billions of years ago? How did the world select only one enantiomer biochemically and develop an excess of that enantiomer? Answering these questions will give researchers more insight into the evolution of proteins, enzymes, and microbes on Earth.
The question of homochirality on Earth is an active area of research today. Three specific hypotheses incorporating all branches of the natural sciences—physics, chemistry, and biology—will provide the missing links that will help us find more finite solutions. These hypotheses include: photolysis of one enantiomer of a racemic amino acid with circularly polarized ultraviolet light, transfer of chirality from enantioenriched amino acids to proteinogenic amino acids, and aqueous amplification of one enantiomer of an amino acid residue.
Circularly Polarized Ultraviolet Light
Circularly polarized light (CPL) is an electromagnetic wave whose electric vector spirals clockwise or counterclockwise along its direction of travel. Right and left-handed CPL (R- and LCPL) appear in various places in interstellar space. For example, sunlight is rich in ultraviolet light. This light is scattered and circularly polarized at low levels, but levels are sufficient to provide a slight excess of LCPL on earth. More substantial sources of CPL are supernovas, stars at the end of their lifecycle. Upon blast, supernovas expand and pick up additional materials to form a supernova remnant. Synchrotron radiation from supernova remnants also contains circularly polarized light. In a neutron star, this synchrotron radiation would have one chirality above a circulation plane and the opposite below it. Thus, one form of circularly polarized light (for example, RCPL) can be directed into our part of the universe, while the opposite polarized light (LCPL) would be sent in the opposite direction.
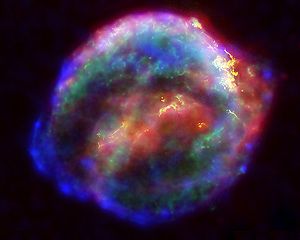
This concept led to the hypothesis today that circular polarized light from deep within space could selectively eliminate one enantiomer of a racemic mixture of amino acids on a carbonaceous chondritic meteorite, thus creating a slight enantiomeric excess that could eventually be transferred to Earth. In the 19th century, right and left-handed circularly polarized light, which, like enantiomers, are mirror images of each other, were thought to be capable of stereoselectively interacting with chiral molecules. In 1897, J. H. van’t Hoff proposed the idea that optically active substances, such as enantiomers of amino acids, might be formed in nature from an interaction with R- and LCPL. It wasn’t until 1929, however, that this hypothesis was supported in a lab by Kuhn and Brown. At this time, the first successful asymmetric photolysis of racemic substance using ultraviolet circular polarized light was accomplished and soon accepted by many as the agent responsible for the natural origin of optical activity.
Today, similar studies are still undergoing to verify the assertion that circular polarized light was involved in the prebiotic foundations of optical activity on earth. Flores, et al (1976) provided a novel approach to photolyzing a racemic amino acid (RS-leucine) with ultraviolet R- and LPCL. Using a LiF Fresnel rhomb, Flores, et al converted linear waves of length 212.8 nm—closely approximating the desired 211 nm—to CPL. This novel approach had never before been achieved in a laboratory and allowed them to study the effects of R-and LCPL on a racemic mixture of leucine using gas chromatography. The results confirmed studies conducted a century earlier.
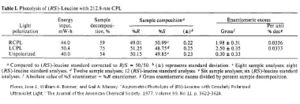
The (R)-leucine component of (RS)-leucine selectively absorbed RCPL and was degraded more extensively than the (S)-leucine component. On the other hand, the (S)-leucine component of the racemate selectively absorbed LCPL and was degraded more extensively than the (R)-leucine component. In fact, the enantiomeric excesses were almost “equal and opposite,” as depicted in Table 1.
Unpolarized light had no selective effect on leucine enantiomer degradation. Before this publication, CPL flux in scientific studies had been insufficient at short (UV) wavelengths to have an effect on asymmetric amino acid degradation. Flores, et al, however, demonstrated the first asymmetric photolysis of amino acids using a new type of laser and even reported the second highest-reported photolysis of their racemate.
The discovery of meteorites with enantiomeric excesses of amino acids in 1997 further revolutionized the idea of extraterrestrial origins of homochirality. Specifically, the Murchison meteorite—a carbonaceous chondrite—dates back 4.5 billion years. Cronin, et al discovered that this meteorite contains a significant enantiomeric excess of two (L)-amino acids: isovaline and α-methylnorvaline (Fig. 1).

Though the excesses discovered are slight, they are enough to indicate an extraterrestrial asymmetric influence on biochemical evolution before the origin of life.
Continued research such as this will support the assertion that circularly polarized light was involved in the prebiotic genesis of optical activity and the formation of homochiral molecules that could be used in the development of microbes on earth.
Transfering Chirality to Proteinogenic Amino Acids

Before circular polarized light can disintegrate one enantiomer of an amino acid, the organic molecule must first be created in interstellar space. The most commonly accepted mechanism for the creation of amino acids in the universe is the Strecker reaction. Devised by Adolph Strecker in 1850, this reaction mechanism makes racemic α-amino acid from a carbonyl compound, ammonia, hydrogen cyanide, and water, all of which have been detected in interstellar space. Once these amino acids are formed, they are picked up by meteorites, and as they pass a supernova remnant, CPL emanated from the star creates an enrichment of only one enantiomer.
The enantiomeric excess of one amino acid in meteorites demonstrates the possibility of an extraterrestrial homochiral origin. For life to arise, however, it is necessary that this homochirality be transferred to the prebiotic soup that was earth billions of years ago. One study proposes a novel approach to the transfer of this homochirality from an α-amino acid to biologically-relevant molecules on earth (Levine, et al, 2008) using compounds that were either present on earth or else arrived with the meteorite.
Levine, et al, demonstrate that (L)-α-methylvaline, discovered on the Murchison meteorite, can transaminate phenylpyruvate to (L)-phenylalanine in a 37 % enantiomeric excess and pyruvate to (L)-alanine in up to 20 % enantiomeric excess using a copper catalyst (Fig. 1). They also demonstrate that this novel copper-catalyzed decarboxylative transamination can be extended to the synthesis of (L)-valine.
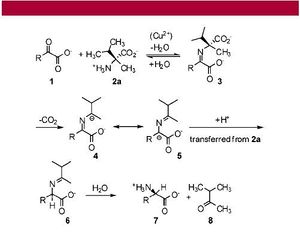
Levine, et al, first provide a never-before seen synthesis of (L)-α-methylvaline with chemical means, synthesizing it in an 8 % yield from (L)-isoleucine (Fig. 2). This starting enantiomer was then added in solution to a pyruvate derivative and cupric sulfate, yielding the enantiomer (L)-phenylalanine (Fig. 3). The authors then obtained mechanistic insight into the enantiomeric selectivity in the copper-catalyzed reaction using highly advanced computer technology. They discovered that one potential reaction intermediate is a square planar copper (II) complex. Analysis of the van der Waals surface of this complex suggested that only side of the α-carbon is accessible to interact with a second molecule—the other side is sterically hindered by bulky benzyl groups. Decarboxylation and selective protonation of the α-carbon from the accessible side will then lead to a single enantiomer of (L)-phenylalanine, passed from the enantiomer (L)-α-methylisoleucine to molecules on earth.
The best temperature for this reaction recorded was 160 °C for 60 minutes. Shorter reaction temperatures and times yielded a lower enantiomeric excess of phenylalanine. These reaction conditions, free of solvent and hot, closely mimic conditions thought to exist in a prebiotic earth. Levine, et al, thus provides a highly plausible transfer of homochirality from amino acids on meteorites to biologically-relevant molecules on a young earth billions of years ago.
Aqueous Amplification of Enantiomers
Include some current research in each topic, with at least one figure showing data.
Conclusion
Overall paper length should be 3,000 words, with at least 3 figures.
References
(1) Bailey, Jeremy, Antonio Chrysostomou, J. H. Hough, T. M. Gledhill, Alan McCall, Stuart Clark, Francois Menard, and Motohide Tamura. “Circular Polarization in Star-Formation Regions: Implications for Biomolecular Homochirality.” Science. 1998. Volume 281, p. 672-674. http://www.jstor.org/stable/2895546?seq=1
(2) Bonner, William A. “The Origin and Amplification of Biomolecular Chirality.” Department of Chemistry. Stanford University. 1991.http://journals.ohiolink.edu/ejc/pdf.cgi/Bonner_William_A.pdf?issn=01696149&issue=v21i0002&article=59_toaaobc
(3) Cronin, John R., and Sandra Pizzarello. “Enantiomeric Excesses in Meteoritic Amino Acids.” Science. 1997. Volume 275, No 91. 951-955. http://www.sciencemag.org/cgi/reprint/275/5302/951.pdf
(4) Flores, Jose J., William A. Bonner, and Gail A. Massey. “Asymmetric Photolysis of (RS)-Leucine with Circularly Polarized Ultraviolet Light.” The Journal of the American Chemical Society. 1977. Volume 99, No 11. p. 3622-3624. http://pubs.acs.org/doi/pdf/10.1021/ja00453a018?cookieSet=1.
(5) Glavin, Daniel P. and Jason P. Dworkin. “Enrichment of the Amino Acid L-Isovaline by Aqueous Alteration of Cl and CM Meteorite Parent Bodies.” PNAS. 2009.
(6) Levine, Mindy, Craig Scott Kenesky, Daniel Mazori, and Ronald Breslow. “Enantioselective Synthesis and Enantiomeric Amplification of Amino Acids under Prebiotic Conditions. Organic Letters. 2008. Volume 10, No 12. p. 2432-2436. http://www.dcb-server.unibe.ch/groups/reymond/education/chembiol/model_chemistry/2.pdf
(7) "NASA: Supernova Remnant Turns 400." 2004. http://www.nasa.gov/multimedia/imagegallery/image_feature_219.html
(8) "Precambrian Fossils." 2008. http://www.fossilmuseum.net/Paleobiology/Precambrian-Fossils.htm.
(9) Spitzer, Lyman. Physical Processes in the Interstellar Medium. Wiley InterScience. 2007.
(10) Toxvaerd, Soren. “Origin of Homochirality in Biosystems.” International Journal of Molecular Sciences. 2009. Volume 10. p. 1290-1299. http://dirac.ruc.dk/~st/articles/ijms-10-01290.pdf
Edited by student of Joan Slonczewski for BIOL 238 Microbiology, 2009, Kenyon College.