Pneumococcal disease: Difference between revisions
No edit summary |
|||
Line 1: | Line 1: | ||
{{Conway}} | |||
{{ | |||
[[File:Strep pneumo micrograph.jpeg|400px|thumb|right|Scanning electron micrograph of <i>Streptococcus pneumoniae</i>. From: http://www.news-medical.net/image.axd?picture=9996_lores.jpg]] | [[File:Strep pneumo micrograph.jpeg|400px|thumb|right|Scanning electron micrograph of <i>Streptococcus pneumoniae</i>. From: http://www.news-medical.net/image.axd?picture=9996_lores.jpg]] | ||
Latest revision as of 16:34, 11 February 2016
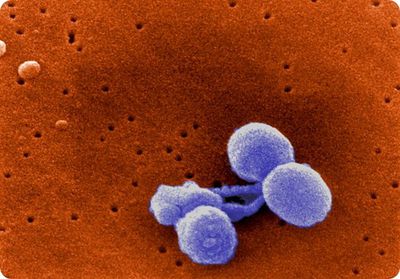
Etiology/Bacteriology
Taxonomy
| Domain = Bacteria | Phylum = Firmicutes | Class = Bacilli | Order = Lactobacillales | Family = Streptococcaceae | Genus = Streptococcus | Species = S. pneumoniae [1]
Description
Morphology
Streptococcus pneumoniae is a Gram-positive, non-motile, non-spore-forming bacterium [2]. S. pneumoniae cells appear as lance-shaped cocci and typically form in pairs (diplococci) but can also appear as single cocci or cocci chains [2,3]. Single S. pneumoniae cells range from 0.5-1.25 µm in diameter [2]. Pneumococcal cells lack the M protein on its surface as virulence factor, distinguishing it from other streptococci [2]. The cell wall of pneumococci contains peptidoglycan and techoic acid, with the techoic acid attached to every third N-acetylmuramic acid [2]. The cell membrane of S. pneumoniae has lipotechoic acid attached to it through a lipid moiety [2]. S. pneumoniae contains an estimated 500 proteins on its surface, and many strains exhibit pili [2]. A polysaccharide capsule also covers the exterior of S. pneumoniae [2]. Serotypes of S. pneumoniae are determined by the structure of the capsule, 93 of which have been presently identified [15].
Cultivation

S. pneumoniae is a fastidious bacterium that grows optimally at 35-37°C with approximately 5% carbon dioxide [3]. Pneumococcal cultures require a source of catalase in order to neutralize the hydrogen peroxide produced by the bacterium as a means of competing with other commensal bacteria [2]. Because of this, it is typically grown on blood agar (as a source for catalase) but can also be cultured on a chocolate agar plate [2,3]. S. pneumoniae is an alpha-hemolytic bacterium and will produce a green-colored zone on blood agar [2,3]. On this medium, S. pneumoniae colonies appear as small, grey, and moist colonies [3]. Serotypes 3 and 37 appear mucoid on blood agar [2]. S. pneumoniae is also a fermentative aerotolerant anaerobe [2]. The generation time of S. pneumoniae on blood agar is 20-30 minutes [2]. Pneumococcal colonies appear raised during the first 24 hours of growth, but between 24-48 hours, the colonies flatten, and the central portion of the colonies depress [3].
Pathogenesis
Transmission
Transmission of S. pneumoniae occurs through coughing, sneezing, or person-person oral contact via microaerosol droplets from the nasopharynx of human carriers [4,5]. In addition, transmission can occur through autoinoculation in those who carry S. pneumoniae in their upper respiratory tract [5]. Crowding, season, and upper respiratory infection or pneumococcal disease in the host are factors that influence the transmission of the bacterium [5]. Though transmission is common, infection is infrequent due to the ability of hosts to asymptomatically carry S. pneumoniae in the nasopharyngeal region [5].
Infectious dose, incubation, colonization
The infectious dose of S. pneumoniae is unknown for humans [7]. However, research on animal models has shown that S. pneumoniae is able to cross the blood-brain barrier at 104 bacteria and can cause sepsis or pneumonia at 107 and 108 bacteria, respectively [7]. The incubation period S. pneumoniae is one to three days [5]. S. pneumoniae colonizes the nasopharyngeal and upper respiratory airway mucosae [8]. In the nasopharynx, S. pneumoniae enables Staphylococcus aureus and Haemophilus influenzae to colonize, while competing for nutrients as well [10]. If S. pneumoniae is allowed to uncontrollably colonize the lung, meninges, or middle ear, the bacterium will lyse and trigger inflammation [9].
Epidemiology
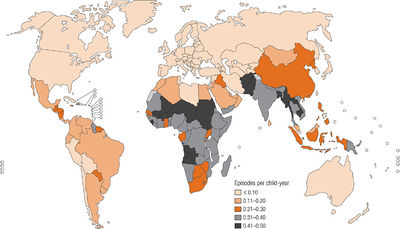
S. pneumoniae is an endemic pathogen worldwide and causes serious disease in children living within developing countries [11]. Diseases caused by S. pneumoniae include: acute otitis media, sinusitis, pneumonia, and bacterial meningitis [12,13]. In developing countries, it is estimated that pneumococcal pneumonia kills more than one million children under the age of five per year [11]. The most susceptible age groups to S. pneumoniae are children less than two years of age and adults over 65 [14]. Though the incidence of pneumococcal pneumonia did not significantly change during the 20th century, the discovery of antibiotics decreased the fatality rate significantly [12]. In the Western world, yearly pneumonia incidence is 1% [14]. Serotypes of S. pneumoniae are capable of exchanging genetic material with each other to recombine their genomes [16]. Because of this, antibiotic resistance has developed worldwide, particularly in the serotypes that most commonly affect children [14].
Virulence factors
Pili
The capsule and pili contribute to the virulence of S. pneumoniae. The first step in pneumococcal invasion of the host is attachment via pili to epithelial cells of the upper respiratory tract [2]. Pili are encoded by the rlrA islet, and experiments on nonpilated vs. pilated pneumococci have demonstrated that the pilated bacteria adhere to the host cells in greater numbers than nonpilated bacteria [2].
Capsule
The polysaccharide capsule of S. pneumoniae serves as a protective mechanism from the host immune response [2]. When the complement pathway is activated, the capsule resists phagocytosis by interfering with the binding of the complement protein C3b to the pneumococcal surface [2].
Pneumococcal surface protein A
Pneumococcal Surface Protein A (PspA) is surface protein located on the cell wall of S. pneumoniae [17,18]. The function of PspA is to inhibit opsonization and phagocytosis of pneumococci by the host’s complement system [2]. PspA carries a highly polar electrostatic charge [19]. The negatively charged end of the protein inhibits complement activation [19]. Mice that received antibodies against PspA acquired passive protection to S. pneumoniae, demonstrating the effect of PspA on the virulence of S. pneumoniae [2].
Hyaluronate lyase
Hyaluronate Lyase (Hyl) is another surface protein expressed by most strains of S. pneumoniae [20]. Hyl is a member of the hyaluronidase enzyme group, which enables tissue invasion of pathogens mainly through the hydrolysis of extracellular matrix components [21,22]. In S. pneumoniae, Hyl degrades hyaluronan in connective tissues, which increases tissue permeability and ease of pneumococcal entry to the host [23]. Increased tissue permeability through the action of Hyl has been linked to wound infections, pneumonia, bacteremia, and meningitis [24].
Pneumolysin
Pneumolysin (Ply) is a cytoplasmic enzyme that contributes to the evasion of host physical barriers by S. pneumoniae. Ply kills ciliated bronchial epithelial cells through cytotoxicity, slows ciliary beating, and disrupts tight junctions of the bronchial epithelial monolayer [25,26]. Because of this, it is more difficult for the host to clear mucus from the lower respiratory tract, which enables the spread and infection by S. pneumoniae [26]. Ply also has a cytotoxic effect on alveolar epithelial cells and pulmonary endothelial cells [27]. The disruption of the alveolar-capillary boundary causes edema and hemorrhage and subsequent alveolar flooding, which provides nutrients for the pneumococci [27]. In addition, the alveolar flooding allows S. pneumoniae to enter the pulmonary interstitium and then the bloodstream [27]. Ply also interferes with the immune response by inhibiting neutrophil and monocyte activity as well as antibody and cytokine production [27].
Autolysins
Autolysins, which are enzymes that degrade bacterial cell walls and lead to cell lysis, contribute to the pathogenicity of S. pneumoniae [28]. Pneumococci utilize three main autolysins: LytA, LytB, and LytC [24]. By lysing cell walls, LytA causes the cell wall components to trigger inflammation in the host [29]. In addition, LytA releases cytoplasmic bacterial proteins to the host environment, including additional virulence factors such as Ply, whose activity is dependent on autolysin activity [24,30]. The functions of LytB and LytC have been less studied than that of LytA, but it is known that LytB is a glucosaminidase that causes cell separation, and that LytC functions similarly to a lysozyme [2].
Pneumococcal surface antigen A
Pneumococcal surface antigen A (PsaA) is a protein attached to the cell membrane of S. pneumoniae with a covalently attached lipid component [31]. The function of PsaA is to transport manganese and zinc ions into the cytoplasm [32].
Choline binding protein A (CbpA)
Choline binding protein A (CbpA) is an adhesin on the surface of S. pneumoniae [24]. CbpA belongs to the choline binding protein (CBP) family along with PspA and LytA due to the common choline binding motif [24]. CbpA enables pneumococci to adhere to host tissues and colonize by binding the platelet-activating factor (PAF) receptor of host cells [33].
Neuraminidase
Neuraminidase is an enzyme that is theorized to enhance the colonization of S. pneumoniae to host cell surfaces [34]. Host cells express glycans on their surface, which includes molecules such as mucin, glycolipids, and glycoproteins [24]. Neuraminidase cleaves terminal sialic acid from the glycans, which most likely changes the glycosylation patterns of the host cells, reveals surface receptors, and enables S. pneumoniae to adhere to the host cells [35]. Neuraminidase exists in two forms: NanA and NanB, though the reason behind this redundancy is unclear [24].
Immunoglobulin A (IgA) protease
Immunoglobulin A (IgA) protease is an exoenzyme of S. pneumoniae that cleaves IgA into Fab and Fc fragments [2,38]. Though the action of IgA protease has been observed in humans, its significance in the pathology of S. pneumoniae has not been well-studied due to the inability of an animal model to demonstrate IgA cleavage [38].
Clinical features
Symptoms
S. pneumoniae causes three major clinical diseases: pneumonia, meningitis, and bacteremia [3]. Fever and chills or rigors characterize the onset of pneumococcal pneumonia [3]. Common symptoms of pneumococcal pneumonia include pleuritic chest pain, a cough that produces rust-colored and mucopurulent sputum, dyspnea, tachypnea, hypoxia, tachycardia, malaise, and weakness [3]. Less common symptoms include nausea, vomiting, and headaches [3]. Common symptoms of pneumococcal meningitis include headache, lethargy, vomiting, irritability, fever, nuchal rigidity, cranial nerve signs, seizures, and coma [3]. One-fourth of patients suffering from pneumococcal meningitis suffer from comorbid pneumonia [3]. Symptoms of pneumococcal bacteremia are similar to pneumococcal pneumonia and meningitis with the additional symptom of joint pain [36].
Morbidity and Mortality
Worldwide morbidity statistics for pneumococcal disease are difficult to estimate, but the World Health Organization (WHO) estimated that disease due to S. pneumoniae occurs in 10-100 cases per 100,000 in Europe and the United States [37]. WHO also estimated in 2005 that pneumococcal disease resulted in 1.6 million deaths worldwide per year [37]. 0.7-1 million children under the age of five die due to pneumococcal infection annually, and most of these deaths occur in developing countries [37]. Pneumococcal deaths in developing countries include a disproportionate number of children under two years of age [37].
Diagnosis
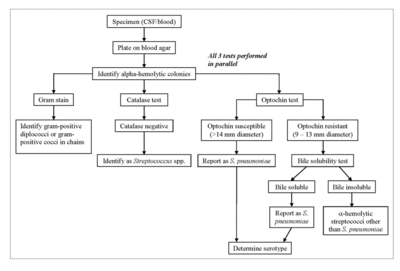
S. pneumoniae can be positively identified through a battery of tests performed on an isolate from a cerebrospinal or blood sample [3]. The sample is first cultured on a blood agar plate [3]. Alpha-hemolytic colonies are isolated, and then a Gram stain, catalase test, and optochin test are then performed in parallel [3]. If S. pneumoniae is the isolate, the Gram stain will reveal Gram-positive diplococci or cocci in short chains [3]. The catalase test determines whether or not the isolate contains catalase to degrade hydrogen peroxide into water and oxygen gas, which differentiates between Gram-positive cocci [3]. Staphylococcus species are catalase-positive, while Streptococcus and Enterococcus species are catalase-negative [3]. A Gram-positive, catalase-negative isolate will be identified as a probable Streptococcus species [3]. The optochin test determines whether or not the isolate is sensitive to optochin [3]. The majority of S. pneumoniae strains are sensitive to optochin, and a Gram-positive, catalase-negative, optochin-sensitive isolate will lead to a positive identification of S. pneumoniae [3]. However, some strains of S. pneumoniae and members of the viridans streptococci are optochin-resistant [3]. A bile solubility test is performed in order to differentiate S. pneumoniae from all other alpha-hemolytic streptococci [3]. After the Gram stain, catalase test, and optochin test, a bile-insoluble result identifies the microbe as an alpha-hemolytic streptococcus other than S. pneumoniae. A bile-soluble result identifies the microbe as S. pneumoniae [3].
Treatment
Penicillin is the antibiotic most commonly used to treat pneumococcal infections [14]. Dosage is typically 3-4 grams per day of oral penicillin V or 1-3 grams of penicillin G intravenously every 6-8 hours. However, since the 1980s, penicillin-resistant strains have complicated the treatment of pneumococcal infection [14]. In addition, resistance to tetracyclines, cotrimoxazole, chloramphenicol, macrolides, and clindamycin has arisen in β-lactam-resistant strains [14]. In the case of penicillin-resistant strains of S. pneumoniae, fluoroquinolones such as levofloxacin and moxifloxacin are typically used [40]. However, overuse of fluoroquinolones has led to resistance as well [41]. Critically ill patients without type 1 allergies to penicillin are primarily treated with cefotaxime or ceftriaxone [40]. Alternative treatments to pneumococcal infections include carbapenems, newer quinolones, clindamycin, telithromycin, linezolid, and vancomycin [42].
Prevention
Vaccination
Children worldwide, especially those under two years of age, are disproportionately affected by pneumococcal infection [37]. Vaccination of children under two for pneumococcal disease is above 90 percent effective [43]. A heptavalent protein-polysaccharide vaccine has been developed specifically for children [14]. In young healthy adults, vaccination is 70-80 percent effective [14]. The vaccine available for adults is a 23-valent capsule polysaccharide vaccine that contains 90 percent of the pneumococcal serotypes that cause disease [14]. In the elderly, vaccination is 50- 60 percent effective [44]. Influenza vaccination also reduces pneumococcal pneumonia in the elderly [14]. The polysaccharide vaccine is mostly ineffective with immunocompromised patients [45]. However, pneumococcal disease has fallen in the United States after the introduction of general immunization of children with the heptavalent vaccine, and pneumococcal disease has also fallen in unvaccinated adults [46].
Hygiene and cleanliness
Frequent handwashing with soap and water, or with hand sanitizer containing 60 percent alcohol if soap and water are unavailable decreases the transmission and infection rates of S. pneumoniae [47]. Avoidance of touching the eyes, nose, and mouth will prevent infection, and covering the mouth with a tissue or sleeve when coughing or sneezing will prevent transmission [47]. Avoiding close contact with those suffering with pneumococcal infections will prevent transmission of the disease as well [47].
Host Immune Response
S. pneumoniae utilizes numerous mechanisms to avoid the host immune response, both innate and adaptive. Hyl degrades connective tissues, which increases tissue permeability and enables S. pneumoniae to invade the host with greater ease [23]. Upon infection, S. pneumoniae resists opsonization by the complement protein C3b and phagocytosis by macrophages in the tissues through the action of PspA and the polysaccharide capsule surrounding the bacterium [2]. When neutrophils are recruited to the site of infection by macrophages, Ply interferes with neutrophil activity and cytokine production [27]. During the adaptive immune response, Ply also interferes with antibody production [27]. In addition, IgA protease cleaves IgA into Fab and Fc fragments, which interferes with the host’s neutralization of the bacterium in mucosal tissues [2,38]. S. pneumoniae also evades the host immune response through its numerous (93) serotypes [25]. Because of this, S. pneumoniae is able to reinfect the host without triggering immunological memory through the presentation of a previously unrecognized antigen of a new serotype [15]. Because of all of the mechanisms that S. pneumoniae has to evade an immune response, mortality due to pneumococcal infection occurs primarily in patients with an underdeveloped immune response, including children under two years of age, patients with HIV, and the elderly [14].
References
1. UniProt. Taxonomy: Species Streptococcus pneumoniae. Available at http://www.uniprot.org/taxonomy/1313.
2. Todar K. Streptococcus pneumoniae. In Todar’s Online Textbook of Bacteriology. Available at http://textbookofbacteriology.net/S.pneumoniae.html.
3. Centers for Disease Control and Prevention. Identification and characterization of Streptococcus pneumoniae. Available at http://www.cdc.gov/meningitis/lab-manual/chpt08-id-characterization-streppneumo.pdf.
4. Ryan KJ, Ray CG (ed). 2004. Medical Microbiology, 4th ed. The McGraw-Hill Companies, United States of America.
5. Centers for Disease Control and Prevention. Pneumococcal Disease. Available at http://www.cdc.gov/vaccines/pubs/pinkbook/downloads/pneumo.pdf.
6. Chen CJ, Huang YC, Su LH, Lin TY. 2007. Nasal carriage of Streptococcus pneumoniae in healthy children and adults in northern Taiwan. Diagn. Microbiol. Infect. Dis. 59(3):265-269.
7. Orihuela CJ, Gao G, McGee M, Yu J, Francis KP, Tuomanen E. 2003. Organ-specific models of Streptococcus pneumoniae disease. Scand. J. Infect. Dis. 35(9):647-652.
8. Kadioglu A, Weiser JN, Paton JC, Andrew PW. 2008. The role of Streptococcus pneumoniae virulence factors in host respiratory colonization and disease. Nat. Rev. Microbiol. 6(4): 288-301.
9. Public Health Agency of Canada. Streptococcus pneumoniae: pathogen safety data sheet- infectious substances. Available at http://www.phac-aspc.gc.ca/lab-bio/res/psds-ftss/streptococcus-pneumoniae-eng.php.
10. Margolis E, Yates A, Levin BR. 2010. The ecology of nasal colonization of Streptococcus pneumoniae, Haemophilus influenzae, and Staphylococcus aureus: the role of competition and interactions with the host’s immune response. BMC Microbiol. 10:59. Available at http://www.biomedcentral.com/1471-2180/10/59.
11. Monto AS. 1989. Acute respiratory infection in children of developing countries: challenge of the 1990s. Rev. Infect. Dis. 11(3):498-505.
12. Austrian R. 1981. Some observations on the pneumococcus and on the current status of pneumococcal disease and its prevention. Rev. Infect. Dis. (Suppl 3):S1-17.
13. Austrian R. 1999. The pneumococcus at the millennium: not down, not out. J. Infect. Dis. 179(Suppl 2):S338-S341.
14. Ortqvist A, Hedlund J, Kalin M. 2005. Streptococcus pneumoniae: epidemiology, risk factors, and clinical features. Semin. Respir. Crit. Care Med. 26(6):563-574.
15. Brueggemann A, Peto T. 2004. Temporal and geographic stability of the serogroup-specific invasive disease potential of Streptococcus pneumoniae in children. Infect. Dis. 190:1203–1211.
16. Dillard JP, Vandersea MW, Yother J. 1995. Characterization of the cassette containing genes for type 3 capsular polysaccharide biosynthesis in Streptococcus pneumoniae. J. Exp. Med. 181:973-983.
17. McDaniel LS, Scott G, Kearney JF, Briles DE. 1984. Monoclonal antibodies against protease-sensitive pneumococcal antigens can protect mice from fatal infection with Streptococcus pneumoniae. J. Exp. Med. 160:386–397.
18. Waltman WD, McDaniel LS, Gray BM, Briles DE. 1990. Variation in the molecular weight of PspA (pneumococcal surface protein A) among Streptococcus pneumoniae. Microb. Pathog. 8:61–69.
19. Jedrzejas MJ, Hollingshead SK, Lebowitz J, Chantalat L, Briles DE, Lamani E. 2000. Production and characterization of functional fragment of pneumococcal surface protein A. Arch. Biochem. Biophys. 373:116–125.
20. Boulnois GJ, Mitchel TJ, Saunders K, Owen R, Canvin J, Shepherd A, Camara M, Wilson R, Feldman C, Steinford C, Bashford L, Pasternak C, Andrew PW. 1991. Analysis of some putative protein virulence factors of Streptococcus pneumoniae, p 83-87. In Dunny GM, Cleary PP, McKay LL (eds), Genetics and molecular biology of streptococci, lactococci, and enterococci. American Society of Microbiology, Washington, DC.
21. Duran-Reynals F. 1933. Studies on a certain spreading factor existing in bacteria and its significance for bacterial invasiveness. J. Exp. Med. 58:161–181.
22. Kreil G. 1995. Hyaluronidases—a group of neglected enzymes. Protein Sci. 4:1666–1669.
23. Boulnois GJ. 1992. Pneumococcal proteins and the pathogenesis of disease caused by Streptococcus pneumoniae. J. Gen. Microbiol. 138:249–259.
24. Jedrzejas MJ. 2001. Pneumococcal virulence factors: structure and function. Microbiol. Mol. Biol. Rev. 65:187-207.
25. Rayner CFJ, Jackson AD, Rutman A. 1995). Interaction of pneumolysin-sufficient and -deficient isogenic variants of Streptococcus pneumoniae with human respiratory mucosa. Infect. Immun. 63:422–427.
26. Steinfort C, Wilson R, Mitchell T. 1989. Effects of Streptococcus pneumoniae on human respiratory epithelium in vitro. Infect. Immun. 57:2006–2013.
27. Rubins JB, Janoff EN. 1998. Pneumolysin: a multifunctional pneumococcal virulence factor. J. Lab. Clin. Med. 131:21–27.
28. Rogers HJ, Perkins HR, Ward JB. 1980. Formation of cell wall polymers, p 437-460. In Nombela C (ed), Microbial cell wall and membranes. Chapman & Hall, London, United Kingdom.
29. Tuomanen E. 1999. Molecular and cellular biology of pneumococcal infection. Curr. Opin. Microbiol. 2:35–39.
30. Mitchell TJ, Alexander JE, Morgan PJ, Andrew PW. 1997. Molecular analysis of virulence factors of Streptococcus pneumoniae. Soc. Appl. Bacteriol. Symp. Ser. 26:62S–71S.
31. Tam R, Saier MH. 1993. Structural, functional, and evolutionary relationships among extracellular solute-binding receptors of bacteria. Microbiol. Rev. 57:320–346.
32. Dintilhac A, Alloing G, Granadel C, Claverys JP. 1997. Competence and virulence of Streptococcus pneumoniae: Adc and PsaA mutants exhibit a requirement for Zn and Mn resulting from inactivation of putative ABC metal permeases. Mol. Microbiol. 25:727–739.
33. Cundell DR, Gerard NP, Gerard C, Idanpaan-Heikkila I, Toumanen E. 1995. Streptococcus pneumoniae anchor to human cells by the receptor for platelet-activating factor. Nature. 377:435–438.
34. Rosenfeld RM, Doyle WJ, Swarts JD, Seroky J, Pinero BP. 1992. Third-generation cephalosporins in the treatment of acute pneumococcal otitis media. An animal study. Arch. Otolaryngol. Head Neck Surg. 118:49–52.
35. Krivan HC, Roberts DD, Ginsburg V. 1988. Many pulmonary pathogenic bacteria bind specifically to the carbohydrate sequence GalNAc beta 1-4Gal found in some glycolipids. Proc. Natl. Acad. Sci. USA 85:6157–6161.
36. National Foundation for Infectious Diseases. Facts about invasive pneumococcal disease for adults. Available at https://health.state.tn.us/ceds/PDFs/pneuadult.pdf.
37. World Health Organization. International travel and health: pneumococcal disease. Available at http://www.who.int/ith/diseases/pneumococcal/en/.
38. Proctor M, Manning PJ. 1990. Production of immunoglobulin A protease by Streptococcus pneumoniae from animals. Infect. Immun. 58:2733-2737.
39. Musher DM. 1992. Infections caused by Streptococcus pneumoniae: clinical spectrum, pathogenesis, immunity, and treatment. Clin. Infect. Dis.14:801-807.
40. Bartlett JG, Dowell SF, Mandell LA, File TM Jr, Musher DM, Fine MJ. 2000. Practice guidelines for the management of community-acquired pneumonia in adults. Clin. Infect. Dis. 31:347-382.
41. Davidson R, Cavalcanti R, Brunton JL, Bast DJ, de Azavedo JCS, Kibsey P, Fleming C, Low DE. 2002. Resistance to levofloxacin and failure of treatment of pneumococcal pneumonia. N. Engl. J. Med. 346:747-750.
42. Musher DM, Groover JE, Rowland JM, Watson DA, Struewing JB, Baughn RE, Mufson MA. 1993. Antibody to capsular polysaccharides of Streptococcus pneumoniae: prevalence, persistence, and response to revaccination. Clin. Infect. Dis.17:66-73.
43. Lucero MG, Dulalia VE, Parreno RN, Lim-Quianzon DM, Nohynek H, Makela H, Williams G. 2004. Pneumococcal conjugate vaccines for preventing vaccine-type invasive pneumococcal disease and pneumonia with consolidation on x-ray in children under two years of age. Cochrane Database Syst. Rev. (4):CD004977.
44. Melegaro A, Edmunds WJ. 2004. The 23-valent pneumococcal polysaccharide vaccine, I: Efficacy of PPV in the elderly: a comparison of meta-analyses. Eur. J. Epidemiol. 19:353-363.
45. Butler JC, Breiman RF, Campbell JF, Lipman HB, Broome CV, Facklam RR. 1993. Pneumococcal polysaccharide vaccine efficacy: an evaluation of current recommendations. JAMA. 270:1826-1831.
46. Whitney CG, Farley MM, Hadler J, Harrison LH, Bennett NM, Lynfield R, Reingold A, Cieslak PR, Pilishvili T, Jackson D, Facklam RR, Jorgensen JH, Schuchat A. 2003. Decline in invasive pneumococcal disease after the introduction of protein- polysaccharide conjugate vaccine. N. Engl. J. Med. 348:1737-1746.
47. Centers for Disease Control and Prevention. Pneumococcal disease (Streptococcus pneumoniae). Available at http://wwwnc.cdc.gov/travel/diseases/pneumococcal-disease-streptococcus-pneumoniae.
Created by Michael Grassi, a student of Tyrrell Conway at the University of Oklahoma.