The Role of Bacteria in the Health Potential of Yogurt
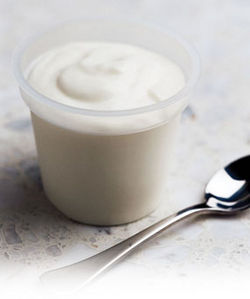
Introduction
At right is a sample image insertion. It works for any image uploaded anywhere to MicrobeWiki. The insertion code consists of:
Double brackets: [[
Filename: PHIL_1181_lores.jpg
Thumbnail status: |thumb|
Pixel size: |300px|
Placement on page: |right|
Legend/credit: Electron micrograph of the Ebola Zaire virus. This was the first photo ever taken of the virus, on 10/13/1976. By Dr. F.A. Murphy, now at U.C. Davis, then at the CDC.
Closed double brackets: ]]
Other examples:
Bold
Italic
Subscript: H2O
Superscript: Fe3+
The Biochemistry Behind Yogurt
Introduce the topic of your paper. What microorganisms are of interest? Habitat? Applications for medicine and/or environment?
Yogurt Production
Benefits of Yogurt
Include some current research, with at least one figure showing data.
Probiotics
Include some current research, with at least one figure showing data.
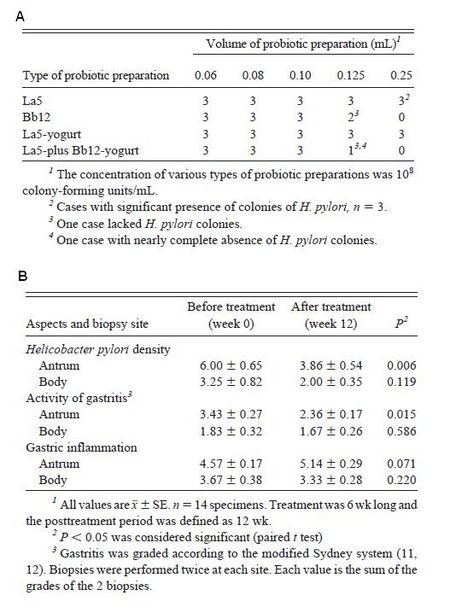
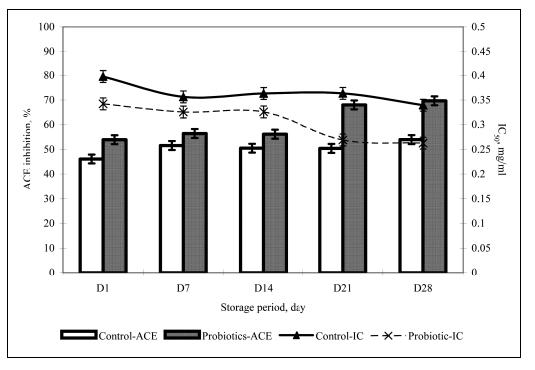
Improving Yogurt
Current Problems
Though the benefits of yogurt are recognized, what is not really understood is if the functionality of yogurt is at its maximum. The most obvious and heavily researched area is increasing the viability of the probiotics that provide the health benefits. However, increasing viability extends to the starter cultures as well, as they have also been documented to contribute some benefits and more importantly, need to be alive in order to be able to produce yogurt even in the presence of other chemicals. Viable cell count/density is the reason not all yogurt brands are equal. Those with live active cultures are indeed healthier than the pasteurized version in which the yogurt is heated, thus killing any live bacteria. However, some brands of yogurt have a higher concentration of live cultures compared to others, though for marketability reasons and overall difficulty in assessing live culture concentration, these numbers are often undisclosed. Improving yogurt by increasing or enhancing bacterial viability is a large field on its own, and is thus discussed below.
Another problem that is not as noticed but certainly plausible is the breakdown of beneficial molecules by the bacteria in yogurt. Paul and Somkuti (2009, 2010) have looked at the degradation of milk-derived peptides that are beneficial to humans. One such peptide is lactoferricin, a 25-amino acid antimicrobial peptide, also reported to have immunostimulatory, antioxidative, and anticarinogenic properties (Paul & Smokuti, 2010). Lactoferricin is released by the pepsin digestion of lactoferrin found in milk. However, the researchers found that when incubated with various strains of S. thermophilus or L. bulgaricus, the amount of lactoferricin decreased over time, suggesting that these species are breaking it down (Fig. 14). Though these results raise the question of how one can get around the hydrolytic breakdown of this peptide with such high potential, it is also worth noting that this particular experiment was carried out only at pH 7. The pH of yogurt is certainly lower, around 4.6, and thus a pH 7 is not representative of the conditions that lactoferricin would be found along with S. thermophilus and L. bulgaricus.
An earlier paper by Paul and Smokuti (2009) looked at the effect of pH on hydrolytic breakdown of certain peptides. Though this experiment focused on different peptides, specifically, an 11-mer antimicrobial and 12-mer hypotensive peptides, they found that the peptides are degraded a lot more quickly at pH 7 (Fig. 15). In fact, at pH 4.5 for some of the strains, over 90% of the original peptide is intact even after incubating for 4 hours. The researchers suggest that while peptides found in the milk at the beginning of yogurt production may be degraded, as the pH of milk is around 6.7-6.8 (www.milkfacts.info), if the peptides are added after yogurt is produced, the peptides may avoid degradation by the bacteria since the pH would have dropped. Thus, the benefits of these antimicrobial and hypotensive peptides may be reaped if they are added at the right time during yogurt production so that they are not broken down before they can be consumed.
Improving functionality of Yogurt
As mentioned above, research today focuses on methods of increasing viability of the live cultures, particularly the probiotics, as they are responsible for conferring the health benefits of yogurt. Perhaps one of the most basic ways to manipulate probiotic viability is by altering environmental conditions and specific starter cultures to find an optimal combination. Abe et al. (2009) manipulated temperature, both for fermentation and storage, and the type of starter culture used (frozen vs. lyophilized, only Bifidobacteria vs. Bifidobacteria along with the presence of Lactococcus lactis ). Combining all of these factors, the researchers concluded that Bifidobacteria are able to survive in yogurt, but are mostly affected by the fermentation temperature and bacterial composition of the starter when they are refrigerated while in storage (Abe et al., 2009). In another study, the type of milk was the variable, where the researchers studied probiotic growth in soy milk compared to cows' milk, the ingredient of standard yogurt (Farnworth et al., 2007). The study reports that while probiotics can grow in soy yogurt, they grow slower and the bacteria utilize different sugars to support their growth compared to cows' milk yogurt.
In addition to manipulating common variables like temperature and starter culture composition, Shah (2000) suggests various methods by which probiotic viability can be increased. First, in order to have an accurate assessment of viability count, he recommends the development of selective enumeration techniques, such as using a media that enhances a specific bacterial species to grow and incubating at an optimal temperature and pH. As for establishing viability, one must pay attention to the combination of organisms present in the yogurt, since there could be antagonistic relationships, as well as to growth requirements and oxygen levels, as some species like Bifidobacteria are anaerobic and are susceptible to oxygen toxicity. Moreover, in order to improve viability, Shah (2000) suggests some techniques like selecting acid and bile resistant strains, using glass containers over plastic containers to let the milk ferment, using a sufficient concentration of inoculum, and adding micronutrients to enhance growth. Other factors suggested by Lourens-Hattings and Viljoen (2001) include the interaction between the species present, chemical composition of the fermentation medium, such as carbohydrate source, final acidity, milk solids content, and availability of nutrients and growth promoters/inhibitors.
In a sense, any factor manipulated in yogurt production (besides altering the genes of the bacteria) is essentially changing the environment, since even the addition of chemicals or other species alters the environment by introducing the presence of a new nutrient or competitor. Many studies have looked at the effect of adding a chemical or molecule on probiotic viability. A group of molecules that has received special attention is the prebiotics. Defined as “a nondigestible food ingredient that beneficially affects the host by selectively stimulating the growth and/or activity of one or a limited number of bacteria in the colon” (Roberfroid, 2007), prebiotics are particularly popular because they are derived from food, and thus one does not need to worry about potential harmful effects since they are regularly consumed anyway. A summary of some of the common prebiotics and its effects on probiotics are shown in Figure 16.
When both prebiotics and probiotics are found in yogurt, it is considered to be a synbiotic, since there is a synergistic effect where the prebiotic enhances the benefits of the probiotic organisms (Schrezenmeir & Vrese, 2001). In addition to prebiotics like inulin, which increases the activity of Lactobacillus acidophilus, increases calcium absorption, and is a good source of dietary fiber (Aryana et al., 2007), and fructoologosaccharides (Akalin et al., 2007), which can be used to replace fat, other compounds have been added to yogurt. These include chitosan, the main derivative of chitin and thought to have hypoglycemic effects (Seo et al., 2009), Versagel©, a whey-protein based fat replacer (Ramchandran & Shah, 2008) and β-glucan, another prebiotic that is just beginning to receive more attention (Vasiljevic, Kealy, & Mishra, 2007). Besides compounds and chemicals, other bacterial species, such as Spirulina platensis, have been added to yogurt to see how it would interact with probiotic bacteria and affect their viability (Akalin, Unal, & Dalay, 2009).
Towards a "Superior" Yogurt
In addition to improving the health potential of yogurt, from an industrial point of view, there is interest in manufacturing a more palatable type of yogurt that appeals to the masses and can be produced efficiently. Such yogurt is generally smooth, mild, and pleasantly sour, though these characteristics may be subject to individual taste. The outcome of yogurt is affected by the quantity and rate of lactic acid addition. L. bulgaricus and S. thermophilus are known to be facultative anaerobic bacteria that can grow in oxygenated environments. It has been found that these species remove the dissolved oxygen in the yogurt mix during fermentation, and only actively begin to produce lactic acid after the dissolved oxygen (DO) concentration in the yogurt mix is lowered to 0 mg/kg (Horiuchi et al., 2009). This suggests that lactic acid production is suppressed by dissolved oxygen in the yogurt mix. By altering the DO concentration to begin with, one could essentially control when lactic acid production begins. In addition to jumpstarting, lactic acid production was also prolonged in a culture that started with 0 mg/kg DO, or reduced dissolved oxygen fermentation (ROF), compared to the control of around 6 mg/kg DO. This means that it takes less time to reach a desired acidity level if yogurt is produced by ROF (Fig. 17A). Moreover, despite initiating lactic acid production much earlier and maintaining it for a longer time, the viable cell counts of the bacterial species and characteristics of the ROF yogurt such as acidity and curd tension were no different from the control yogurt (Horiuchi et al., 2009). One advantage of using ROF is the reduction of production time, as the cultures enter the exponential growth phase sooner. It has been shown that yogurt made at a low temperature produces smooth yogurt and the physical properties of yogurt are improved as the starter culture are given more time to produce aroma substances and other accessory molecules that affect the taste, and are able to block fast acid production (Guzel-Seydim, Sezgin, & Seydim, 2005). Given this fact, Horiuchi et al. (2009) set out to make a yogurt that was smooth but could be counteracted with ROF to reduce the wait time compared to traditional low temperature production, which requires more time in exchange for a smooth product. Using low temperature reduced dissolved oxygen fermentation (LT-ROF), the researchers achieved a "superior" set yogurt with a smooth texture and a strong curd structure. Moreover, the marketability of this type of yogurt production is increased, as Horiuchi et al. (2009) have shown that yogurt produced by LT-ROF takes less time than traditional yogurt produced at 37°C (Fig. 17B), and is comparable to the faster common yogurt production that takes place at 43°C (compare control of Fig. 17A and LT-ROF of Fig. 17B).
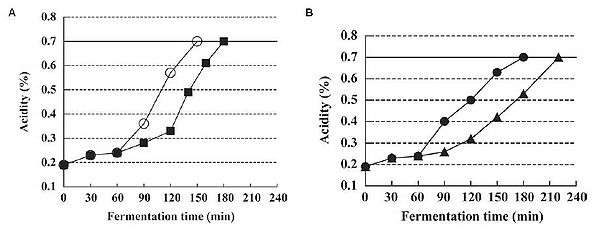
Regardless of the actual percentage of acidity, what is important is how the yogurt appeals to consumers. The LT-ROF yogurt was evaluated to be smoother and milder on average by 200 consumers compared to control yogurt produced at the standard temperature of 43°C (Fig. 18). However, the LT-ROF yogurt turned out to have a smooth texture comparable to the control yogurt made at a lower temperature (37°C). The major difference between these two types of yogurt was the firmness of the curd (Fig. 19). Horiuchi et al. (2009) suggested that having a firm curd was beneficial since manufactured yogurt needs to be transported in trucks, thereby requiring a firmer texture that can withstand the shaking. The researchers thus concluded that the LT-ROW method of producing yogurt was the superior method as it takes about the same time as producing yogurt at 43°C and results in a smooth yogurt that can hold its shape while in transit.
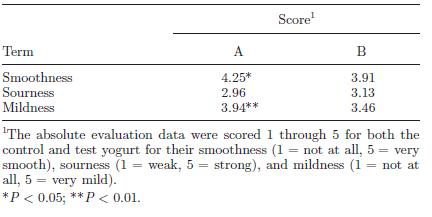
Another study focused on the effect of temperature and starter culture type on the quality of yogurt. Guzel-Seydim, Sezgin, and Seydim (2005) compared how the quality of yogurt changes based on whether it is produced at high (45°C) or low temperatures (35°C) and whether exopolysaccharide-producing or non-producing strains are used. These exopolysaccharides are of interest because it is a ropy, mucoid substance that increases the viscosity of yogurt and decreases whey separation. Guzel-Seydim et al. (2005) quantified the quality of yogurt by looking at the pH, lactic acid percentage, total volatile fatty acids content, acetaldehyde content, tyrosine content, consistency, viscosity, and extent of whey separation of the yogurt samples. They found that the ropy exopolysaccharide-producing strains had a better texture overall when incubated at the lower temperature, but the non-exopolysaccharide strains actually had a better taste, as evaluated by the higher acetaldehyde content. Nonetheless, the researchers suggest that these exopolysaccharide-producing strains may be useful in replacing additives like fat that are used to improve the texture of yogurt. Thus, as is the case with most foods, one would hope to improve not just the taste and texture of yogurt, but also the nutritional value, which also ties back to the health benefits that were described earlier.
Conclusion
Yogurt has a long history and its benefits have been valued by many people, particularly those with gastrointestinal problems. The production behind yogurt is well understood, allowing for improvements and advancements in both the quality and efficient manufacturing of the product. Improving the health potential of yogurt has become a popular field, and for industrial reasons, enhancing the taste and texture, as well as storage life of yogurt is an appealing advancement for yogurt consumers. Yogurt in its basic form is a very eco-friendly product, as humans are essentially consuming the waste products of acidic fermentation. Additionally, the unique taste, texture, and potential for even better health benefits make yogurt an attractive food for people of many cultures.
References
Adolfsson, O., S. N. Meydani, & R. M. Russell. 2004. Yogurt and gut function. Am J Clin Nutr. 80:245–256.
Akalin, A. S., G. Unal., & M. C. Dalay. 2009. Influence of Spirulina platensis biomass on microbiological viability in traditional and probiotic yogurts during refrigerated storage. Ital. J. Food Sci. 21: 356-364.
Akalin, A. S., S. Gonc, G. Unal, & S. Fenderya. 2007. Effects of frutooligosaccharide and whey protein concentrate on the viability of starter culture in reduced-fat probiotic yogurt during storage. Journal of Food Science. 72: M222-M227.
Alvaro, E., C. Andrieux, V. Rochet, L. Rigottier-Gois, P. Lepercq, M. Sutren, P. Galan, Y. Duval, C. Juste, & J. Dore. British Journal of Nutrition. 97: 126–133.
Cornell University Milk Quality Improvement Program. “Yogurt Production.” 29Dec.2006. <http:// www.milkfacts.info/>
Farnworth, E. R., I. Mainville, M.-P. Desjardins, N. Gardner, I. Fliss, & C. Champagne. 2007. Growth of probiotic bacteria and bifidobacteria in a soy yogurt formulation. Journal of Food Microbiology. 116: 174-181.
Gaetke, L.. M., C. J. McClain, C. J. Toleman, and M. A. Stuart. 2010. Yogurt protects against growth retardation in weanling rats fed diets high in phytic acid. Journal of Nutritional Biochemistry. 21: 147-152.
Guarner, F., G. Perdigon, G. Corthier, S. Salminen, B. Koletzkos, & L. Morelli. 2005. Should yoghurt cultures be considered probiotic? Brit. J. or Nutr. 93: 783-786.
Guzel-Seydim, Z. B., E. Sezgin, and A. C. Seydim. 2005. Influences of exopolysaccharide producing cultures on the quality of plain set type yogurt. Food Control. 16: 205-209.
Horiuchi, H., N. Inoue, E. Liu, M. Fukui, Y. Sasaki, and T. Sasaki. 2009. A method or manufacturing superior set yogurt under reduced oxygen conditions. Journal of Dairy Science. 92: 4112-4121.
Kitawaki, R., Y. Nishimura, N. Takagi, M. Iwasaki, K. Tsuzuki, and M. Fukuda. 2009. Effects of Lactobacillus fermented soymilk and soy yogurt on hepatic lipid accumulation in rats fed a cholesterol-free diet. Biosci. Biotchnol. Biochem. 73: 1484-1488.
Paul, M. and G. A. Somkuti. 2009. Degradation of milk-based bioactive peptides by yogurt fermentation bacteria. Lett Appl Microbiol. 49:345–350
Paul, M. and G. A. Somkuti. 2010. Hydrolytic breakdown of lactoferricin by lactic acid bacteria. J Ind Microbiol Biotechnol. 37:173–178.
Ramchandran, L. & N. P. Shah. 2008. Effect of Versagel® on the growth and metabolic activities of selected lactic acid bacteria. Journal of Food Science. 73: M21-M26.
Roberfroid, M. B. 2000. Prebiotics and probiotics: are they functional foods? Am J Clin Nutr. 71:1682S–7S.
Schrezenmeir, J. & M. Vrese. 2001. Probiotics, prebiotics, and synbiotics—approaching a definition. Am J Clin Nutr. 73:361S–4S.
Seo, M. H.,S. Y. Lee , Y. H. Chang, & H. S. Kwak. 2009. Physicochemical, microbial, and sensory properties of yogurt supplemented with nanopowdered chitosan during storage. J. Dairy Sci. 92: 5907-5916.
Shah, N. P. 2000. Probiotic bacteria: Selective enumeration and survival in dairy foods. J. Dairy Sci. 83:894-907.
Slonczewski, J.L. and J.W. Foster. Microbiology: An Evolving Science. New York. W.W. Norton & Company, Inc., 2009. Pp. 595.
Vasiljevic, T., T. Kealy, & V. K. Mishra. 2007. Effects of β-glucan addition to a probiotic containing yogurt. J. Food Sci. 72: C405-411.
Wang, K.-Y., S.-N. Li, C.-S. Liu, D.-S. Perng, Y.-C. Su, D.-C. Wu, C.-M. Jan, C.-H Lai, T.-N. Wang, & W.-M.
Wang. 2004. Effects of ingesting Lactobacillus- and Bifidobacterium-containing yogurt in subjects with colonized Helicobacter pylori. Am J Clin Nutr. 80: 737-741.
Wollowski, I. S.-T. Ji, A. T. Bakalinsky, C. Neudecker, & B. L. Pool-Zobel. 1999. Bacteria used for the production of yogurt inactivate carcinogens and prevent DNA damage in the colon of rats. J. Nutr. 129: 77–82.
Edited by student of Joan Slonczewski for BIOL 238 Microbiology, 2010, Kenyon College.