The Role of Heliobacter pylori in Intestinal Disease
Introduction
By Makarios McCune
Other examples:
Bold
Italic
Heliobacter pylori was first isolated by both Warren and Marshal in 1983 (Houben et al., 1999). The bacteria Heliobacter pylori is a rod shaped, gram negative bacterium equipped with a flagella for chemotaxis (Suzuki et al., 2022). The bacterium is estimated to have infected 4.4 billion individuals worldwide, and is infamous for its association with numerous intestinal diseases and cancer. The bacterium typically infects the stomach and duodenum of the small intestine, and has been known to cause peptic ulcers and gastritis. If left untreated, the inflammation H. pylori can lead to stomach cancer and other serious health complications (Chen et al., 2023). H. pylori is able to entrench itself into the gastric mucus of the stomach by producing urease to alter its morphology. Once situated, the bacterium can avoid detection from the immune system and propagate anti-inflammatory responses. However, Toll-like receptors (TLRs) are able to bind and recognize the bacterium and initiate inflammation. Unfortunately, the chronic inflammation produced by infection can lead to the upregulation of specific proteins and growth factors that increase the ability of cancer associated fibroblasts to metastasize throughout the body (Chen et al., 2023). Infection is typically identified with either a stool antigen test, or a urea breath test. Endoscopies are also used to uncover infection, usually while also scoping out patients with chronic abdominal pain. Treatment for H. pylori infection involves an eradication program, requiring daily intake of various drugs and antibiotics to neutralize the infection. While generally successful, the development of antibiotic resistant strains has reduced the efficacy of some antibiotics - especially clarithromycin and nitroimidazole compounds. The growth of antibiotic resistant strains is due to the excretion of antibiotics into the environment, as well excessive use of antibiotics and negligence regarding following treatment plans. Eradication therapy can also leave lasting effects on the gut microbiome of the patient, as proton pump inhibitors and antibiotics can alter the pH of the environment and eliminate other species native to the stomach. Overtime, this dysbiosis can generate metabolic disorders and other digestive complications. Current solutions to this issue revolve around testing antimicrobial susceptibilities, and redesigning multi-drug eradication therapies.
Sample citations: [1]
[2]
A citation code consists of a hyperlinked reference within "ref" begin and end codes.
To repeat the citation for other statements, the reference needs to have a names: "<ref name=aa>"
The repeated citation works like this, with a forward slash.[1]
Morphology
Every point of information REQUIRES CITATION using the citation tool shown above.
The bacteria Heliobacter pylori is a rod shaped gram negative bacterium. The organism is infamous for its association with development of intestinal cancers. The bacterium typically infects the stomach and duodenum of the small intestine, and has been known to cause peptic ulcers and gastritis. If left untreated, the H. pylori can lead to stomach cancer and other serious health complicate.
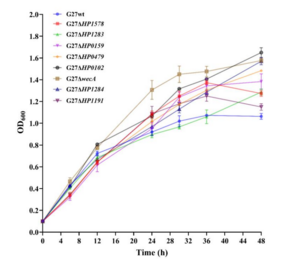
Infection and Immune Response
H. pylori infects around 50% or the worlds total population, with there being an estimated 4.4 billion cases around the globe (Salama et al., 2013 ; Suzuki et al., 2022 ; Mandel et al., 2004). Infection usually occurs orally, from consuming infected food and water sources (Salama et al., 2013). Once inside the body, H. pylori migrates to the digestive system, and is prominently found in the gastric mucus (Salama et al., 2013). Typically, the acidic environment of the stomach is able to neutralize bacteria and pathogens that manage to enter the body. H. pylori circumvents this issue through urease production and chemotaxis to infiltrate the gastric mucus and escape the harsh environment of the stomach lumen (Salama et al., 2013). The urease catalyzes the production of of ammonium ions that interact with the gastric mucus and raises the pH of the system to neutral levels (Salama et al., 2013). This alters the viscoelasticity of the structure and allows H. pylori to pass through rather than become trapped and degraded by antimicrobial agents (Salama et al., 2013).
H. pylori employs a variety of unique strategies to thwart the mechanisms of the body’s immune system. One such strategy is the use of specific protein such as catalse and superoxide dismutase, which are responsible for counteracting the effects of reactive oxygen species deployed by the immune system (Salama et al., 2013). The production of arginase is also used to neutralize nitric oxide production in macrophages and neutrophils that may target the bacterium (Salama et al., 2013). Additionally, H. pylori is able to use to the gastric mucus produced by epithelial cells to hide its pathogen-associated molecular patterns (PAMPs) and from detection by pattern recognition receptors (PRRs) on immune cells (Salama et al., 2013). The lipopolysaccharide (LPS) that lines the outer membrane of H. pylori also assists in defense from the immune system. The LPS on H. pylori is structured to lack phosphate groups on the 1’ and 4’ sections oft the lipid backbone, causing it to be less negatively charged (Salama et al., 2013). This small change is enough to prevent binding and recognition of the LPS by Toll-like receptors (TLRs) (Salama et al., 2013). However, this mechanism is not completely fool-proof, with a study finding that TLR4 could recognize the and bind the to LPS of the bacterium (Mandell et al., 2004). On the other hand, TLR2 could not detect plain LPS, but could recognize the entire intact bacterium, as well as other species of Heliobacter (Mandell et al., 2004). In this study, mice macrophages deficient in TLR4 could still mount an immune response and secrete IL-6, whereas TLR2 knockout cells could not (Mandell et al., 2004). This could be because TLR2 specializes in binding lipoproteins (Salama et al., 2013), making it unable to trigger a cytokine response to solely LPS.
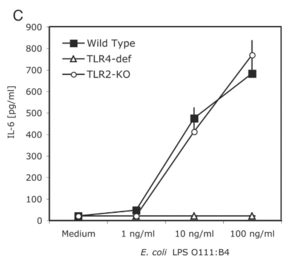
TLR4 T and The flagellin protein belonging to the flagella of H. pylori are also unable to bind to TLR5, owing to a mutation in the N-terminal domain of the protein (Salama et al., 2013). Additionally, H. pylori is able to prevent the induction of pro-inflammatory resposnes in the immune system. The fucosylated ligands of H. pylori are able to foil the C-type lectin receptor DC-SIGN - disrupting the protein complexes and halting downstream signalling for the pro-inflammatory response (Salama et al., 2013). If uptaken by dendritic cells, H. pylori DNA can activate TLR9, which generates an anti-inflammatory response to prolong infection (Salama et al., 2013).
H. pylori can also disrupt action by the adaptive immune system by modulating responses from T cells. The virulence factor VacA, can disorder the interaction within the T cell receptor-IL2 pathway, which hampers T cell proliferation (Salama et al., 2013). VacA has also been found to block nuclear translocation of the the transcription factor NFAT, which is required to activate many T cell related genes (Salama et al., 2013). Moreover, VacA can force dendritic cells to upregulate the production of T regulator cells and IL-10 (Salama et al., 2013). This causes T regulator cells to accumulate in large numbers in the gastric mucus, which lowers overall production of T helper cells and makes it far more difficult for them to eliminate invading H. pylori (Salama et al., 2013).
Despite its innate ability to avoid detection by the immune system, H. pylori is still vulnerable to being bound by NOD1 and NOD2 receptors (Salama et al., 2013). The NOD1 receptor is able to bind and recognize peptidoglycan from H. pylori. Once bound, NOD1 signals for the transcription factor NF-κB, which subsequently causes gastric epithelial cells to secrete β-defensin 2 peptide which terminates H. pylori (Salama et al., 2013).
Cancer Development
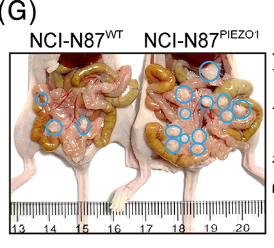
H. pylori is strongly associated with the development of intestinal cancers, and is classified as a group 1 carcinogen by the World Health Organization (WHO) (Ables et al., 2007). It has been estimated that those infected with H. pylori are three times more likely to be diagnosed with gastric cancer (Ables et al., 2007). Gastric cancer itself is ranks fourth for cancer deaths worldwide (Chen et al., 2023). H. pylori causes cancer by stimulating a pro-inflammatory response in the body. The continual persistence of the bacteria leads to chronic inflammation, which then leads to gastric cancer if left untreated. A study found that NFκB activated by infection activates an calcium channel protein called PIEZO1 (Chen et al., 2023). Once activated, PIEZO1 can interact with other proteins like YAP1, which was found to increase metastasis in mouse samples (Chen et al., 2023). Mice with knockout PIEZO1 saw a decrease in YAP1 expression, with YAP1 knockouts having nullified cancer cell migration (Chen et al., 2023). Additionally, upregulation of PIEZO1 and YAP1 proteins was found to correspond to an increase presence of connective tissue growth factor (CTGF), which together with YAP1 and PIEZO1, increased the invasiveness of α-smooth muscle actin cancer associated fibroblasts (Chen et al., 2023). These fibrobalsts can then generate extracellular matrix that can lead to stiffening of cellular tissue by creating tension within tumors (Benn et al., 2023). Stiffening alters the function of affected cells, and encourages them to migrate, also increasing metastasis of the cancer (Chen et al., 2023). Moreover, PIEZO1 and the presence of the cancerous fibroblasts were both positively correlated to infection by H. pylori (Chen et al., 2023). This demonstrates that H. pylori infcetion, the prevalence of calcium channel proteins, hardening of tumor cells, and metastasis of gastric cancer are all intertwined.
Treatment and Complications
The primary and most effective method for handling H. pylori infection is a combination of various identification techniques coupled with eradication treatment. For identification, both the stool antigen test and the urea breath test are typically the most effective and reliable for detecting H. pylori presence, and are recommended by American national standards. (Ables et al., 2007). The stool antigen tests use monoclonal antibodies to detect antigens belonging to H. pylori in the patient’s stool samples (Ables et al., 2007). Meanwhile, the urea breath test requires a patient to consume a pill or liquid containing urea, which is broken down into carbon dioxide by H. pylori. Excess carbon dioxide expelled by the patient is measured to determine the possibility of infection. Endoscopy can also be utilized to confirm H. pylori presence. However, it is suggested that endoscopies should primarily be reserved for determining the pathophysiology of patients with persistent upper abdominal pain, and not solely be used to detect H. pylori due to the invasive nature of the procedure (Ables et al., 2007).
Once identified, H. pylori infection is typically cleared through an H. pylori eradication program. The treatment involves taking a mix of proton pump inhibitors, bismuth salts, and antibiotics for a duration of approximately 10-14 days (Ables et al., 2007). However, treatment can vary greatly, with some therapies lasting for upwards of six weeks (Ables et al., 2007). This combination drug therapy has proven to be potent - with patients reporting an eradication of upwards of 95% (Ables et al., 2007). The number and type of drugs differs with regard to region, with different parts of the world having different standards for H. pylori eradication. North America, Europe, and China prescribe a quadruple drug therapy consisting of two or three antibiotics coupled with a proton pump inhibitor and sometimes bismuth. South Korea and Japan both still rely on the usage of just three drugs, with Japan basing treatment around the gastric suppressant drug vonoprazan (Suzuki et al., 2022). Despite using less drugs, the vonoprazan regimen has proven to be exceedingly effective, with an eradication rate of 89-93%, and a greater than 80% success rate against clarithromycin resistant strains (Suzuki et al., 2022). The antibiotics tetracycline and metronidazole were historically used for treatment, with amoxicillin, tinidazole, and clarithromycin now being utilized (Suzuki et al., 2022).
The largest obstacle for treatment currently is the development of antibiotic resistant strains. Antibiotic resistant H. pylori have been a growing concern, especially in the developed world (Ables et al., 2007). WHO lists clarithromycin resistant H. pylori as a “high-priority species,” which is in the same category as methicillin resistant Staphylococcus aureus (MRSA) (Suzuki et al., 2022). Approximately 10-30% of eradication treatments fail to eliminate H. pylori infection (Houben et al., 1999). Specifically, nitroimidazole resistance is reported to be 10-56% in western nations, and 80-90% in developing nations (Houben et al., 1999). Strains resistant to clarithromycin and nitroimidazole do significantly reduce the eradication percentage of treatment (Houben et al., 1999). An extensive review of treatment literature found that H. pylori resistant to clarithromycin and nitroimidazole compounds have cure rates as low as 0% when treated with certain drug combinations (Houben et al., 1999). The study also concluded that the cure rates for treating patients with nitroimidazole resistant H. pylori have decreased overall by 50% (Houben et al., 1999). This problem of antibiotic resistance is exacerbated by the lack of a simplified method for testing H. pylori resistance, and the fact that multiple resistant strains can coexist within the human body at once (Houben et al., 1999). Antimicrobial susceptibility testing is a common procedure that has proven helpful for determining the most effective treatment option for patients, as well as reducing unnecessary antibiotic usage (Suzuki et al., 2022). Unfortunately, this method does require invasive endoscopy for sample acquisition, and is therefore not a standard medical procedure. Culturing and analyzing H. pylori also requires specialized laboratory equipment, which may be unavailable or costly to implement (Suzuki et al., 2022). While a clear method for combatting resistant strains is still not fully fleshed out, a study did find treatment with rifabutin was 38% in clearing resistant cases (Ables et al., 2007). The use of quadruple drug therapies has also been suggested as a possible solution and has been reported to have some success - although this was tested in a small patient testing pool (Ables et al., 2007 ; Houben et al., 1999). Future research and continued collaboration will be crucial for identifying strains of antibiotic-resistant H. pylori, and employing designing new drugs and therapies for eradication.
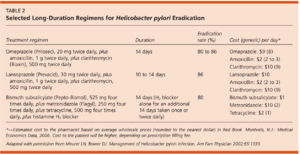
Besides antibiotic-resistance, gut microbiome dysbiosis is another crucial issue tied to H. pylori treatment. Dysbiosis is an imbalance to the local microbiome caused by the introduction or elimination of microorganisms. Because eradication therapy employs numerous antibiotics and gastric acid suppressants, the gut microbiota are directly impacted (Suzuki et al., 2022). Studies recording the effects of eradication treatments found the alpha diversity indexes of patients to be lower 2 weeks following treatment (Suzuki et al., 2022). Furthermore, the microbiome of groups receiving a quadruple drug treatment failed to return to baseline values even after a full year of recovery (Suzuki et al., 2022). Even treatment with vonoprazan saw significant alterations to patients’ microbiomes last for two months before returning to standard levels (Suzuki et al., 2022). It is clear that the attempt to treat an ongoing H. pylori infection comes with the added risk of reshaping the local gut microbiome and possibly causing unexpected metabolic and intestinal issues.
Additionally, H. pylori infection was found to conflict with other popular treatments, such as the usage of nonsteroidal anti-inflammatory drugs (NSAIDS) to treat dyspepsia and inflammation in the gut. Taking NSAIDS with an active H. pylori infection were found to increase the risk for both ulcer bleeding and peptic ulcer disease in infected patients (Ables et al., 2007). Because of this, it is advised that patients diagnosed with dyspepsia and H. pylori undergo eradication therapy before beginning chronic NSAIDS therapy (Ables et al., 2007). Other drug-related complexities require specific planning with medical professionals.
Conclusion
In conclusion, H. pylori is a bacterium with the potential to cause peptic ulcers, gastric cancers, and various intestinal diseases. The most common treatment option for infection is eradication therapy that requires patients to take multiple drugs and antibiotics over a 10-14 day period. Current eradication rates for this treatment range from 80-90%, however the continual emergence of antibiotic resistant strains of H. pylori threaten to neutralize this effectiveness as the prevalence of these strains begins to proliferate. In order to improve treatment options, limit the intestinal dysbiosis, and combat the rise of antibiotic resistance, new drug therapies need to be tested, and affordable and efficient methods of strain resistance need to be researched.
References
Authored for BIOL 238 Microbiology, taught by Joan Slonczewski,at Kenyon College,2024