Adeno-Associated Viruses as Gene Therapy Vectors
By: Chad Kurylo
Adeno-Associated Viruses
Adeno-associated viruses (AAV) are small viruses that affect humans and other members of the primate species (Fig. 1). They belong to the parvovirus family and require a helper virus, such as adenovirus, herpes simplex virus, or vaccina virus, to replicate (1). For this particular reason they are also part of the dependovirus genus. If no helper virus is present, they are able to incorporate themselves into the host cell’s genome and latently replicate as the host cell replicates. When a helper virus infects the cells that the AAV has latently incorporated itself into, it is able to engage in lytic replication and release itself from the cell.
The AAV genome is made up of ssDNA, is very small (4.7kbp), and is flanked by two 145 nucleotide-long inverted terminal repeats (ITR)(1). It codes for two different types of proteins, the first being Rep, which is responsible for replication and rescue of the virus. The second protein, Cap, is a structural protein that is the chief constituent of the icosahedral capsid that houses the viral genome. Furthermore, the AAV genome contains three promoters, p5, p19, and p40, and two open reading frames (Fig. 2). The mRNA transcripts produced in conjunction with each of the promoters is able to code for seven different Rep and Cap proteins due to alternate splicing (1). The AAV genome doesn’t code for a DNA polymerase and is consequently dependent on that provided by the host cell. AAV are very simple viruses, but as we will see, they have an extremely wide variety of medicinal applications.
AAV Binding to Host Receptor and Life Cycle
The first stage in the AAV life cycle is the binding of the particle to a host cell. To bind cell receptors, AAVs use heparan sulphate proteoglycan structures on the cell surface. These structures are composed of have two or three HS groups located near the cell surface or extra-cellular matrix. Once bound to these receptors, they utilize co-receptors on the cell surface, for example, avb5 and FGFR1, which aid in internalization via receptor-mediated endocytosis (2).
Once in the cell, AAVs can partake in either lytic or lysogenic replication. If a helper virus is available, the AAV can engage in a lytic life cycle, which involves rapid replication within the cell, which subsequently bursts, releasing the new particles into the environment. A lysogenic life cycle, however, is what the AAV is initially programmed to do as its gene expression is auto-repressed. It then, when infecting humans, integrates into the host chromosome (19q13.3-qter) of human chromosome 19 using the ITRs and Rep proteins 78 and 68 as guides (2). Once an AAV has latently integrated itself into the host genome, it can be “re-awakened” if the host cell is infected by a helper virus, an adenovirus, a vaccina virus, or a herpes simplex virus (2). At this point, Rep proteins are used to excise the AAV genes out of the host cell’s chromosome where it is replicated and repackaged in the cell. When a large number of these particles have assembled, they burst out of the cell into the environment to restart the cycle.
Using AAV Vectors to Treat Diseases
AAV vectors have been shown to be capable or targeting a wide variety of diseases and malignancies. Common diseases targeted include various types of cancer, neurodegenerative diseases, arthritis, muscular dystrophy, cystic fibrosis, hemophilia B, and many more.
Functionally, AAV are well suited as gene vectors. One important aspect of their function is that they are able to transduce a wide variety of cells regardless of their stage in the cell cycle. This is extremely important because it allows them to target cells that are differentiated, and also cells that are rapidly dividing, such as cancer cells. Furthermore, AAV are desirable as gene vectors because when they infect host cells, they elicit little immune response. If any response is elicited, it is little more than the generation of neutralizing antibodies. No cytotoxic effect is caused, and the host system is relatively unaffected other than the intended, therapeutic, infection. All of these aspects of AAV are important, but probably the greatest advantage of the AAV vector system is that it allows for permanent genetic implantations to occur (2). Unlike the adenovirus, AAV is able to permanently implant its genes into the genome of the host, which creates a relatively-long term effect on the host as opposed to a powerful, transient, effect like that typically produced by adenoviral vectors. AAV are also extremely reliable and predictable when inserting their genes into the host cell’s genome. They always implant their genes into a particular spot on the 19th chromosome of humans, while retroviruses are extremely unpredictable and prone to mutating DNA during implantation (7). If mutations or misplaced insertions occur at this stage of the therapy, malignancies and adverse effects may be observed, compromising the efficacy of the treatment. There are also 11 different serotypes of AAV that are all different in their structure. Certain serotypes, such as AAV2, are specific to particular receptors in the host (6). This aids researchers to mix and match the genomes of these serotypes to find a vector that is specific to a particular receptor in the host. All of these are advantages make using AAV very desirable for a wide variety of gene therapy treatments.
When the capsid proteins are complementary to particular cell surface receptors, they are able to bind those receptors and initiate a process called receptor-mediated endocytosis, which is when the virus is pulled into the cytoplasm within a proteinous vesicle (Fig. 3) (2). At some point the virus breaks out of the vessicle, uncoats, and injects its DNA into the cell's nucleus. At this point, the virus integrates itself into the host cell's genome (2)
While there are a great amount of advantages to using the AAV vector system, there are also a few drawbacks. The main drawback of using AAV as vectors is that the small size of their genome (4kbp) significantly limits the amount of genetic material that it can carry. Oftentimes, almost the entire genome must be replaced in order for the required genes to be placed onto the AAV. By comparing the size of the AAV genome to other types of vectors it becomes evident as to why it is so disadvantageous. Adenoviruses, for example, can carry roughly twice as much material and retroviruses usually hold between 8-10kbp. While the AAV is unable to carry large genes, steps are being taken to discover ways to expand the carrying capacity of AAV vectors by annealing the two inverted terminal repeats (ITR).
AAV Vector Production (2 Transfection Method)
The first step in producing AAV vectors is finding helper viruses that will aid in replicating the AAV. Many times this helper virus is an adenovirus, which needs a minimum of its E2A, E4, and VA1/II gene regions in order to be effective at replicating the AAV. Then, two plasmids are created, one containing the AAV rep and cap genes plus the adenoviral helper genes prescribed above. The other plasmid contains an expression cassette composed of a CMV promoter, multiple cloning site, and SV40 polyA (2). This expression cassette on the second plasmid is also flanked by AAV inverted terminal repeats. This concoction is then transfected into a large quantity of 150mm dishes that contain the 293 line of human embryonic kidney cells, which encode the E1 region of the Ad5 genome (3). After the transfection, the cells are harvested and purified using ultracentrifugation. Another advantage of the AAV system is that large number of AAV can be harvested using these techniques (>10^8vp/ml)(6).
The only portions of the AAV that are absolutely necessary in cis to the vector are the inverted terminal repeats and the therapeutic gene(1). The packaging and structural genes, rep and cap, are able to be provided in trans. This means that the genome of the AAV can essentially be wiped out to make room for recombinant genes.
Gene Therapy of Cystic Fibrosis Using AAV
Cystic fibrosis, a hereditary genetic disorder of the CFTR gene that produces chloride ion channels in the epithelium of airway tissue, which are important for the proper production of mucus, sweat, and digestive juices. In the lungs, defective CFTR genes often result in mucus that is too thick to be efficiently moved out of the system. This means that pathogens in the respiratory system aren’t removed from quickly enough and the person is more susceptible to infection.
Previously, gene therapy using viruses in the respiratory tract has been difficult as respiratory viruses typically have low infection rates. Respiratory viruses have low rates of infection because there is a precarious evolutionary balance that viruses have to tiptoe between virulence and infectivity. If they are too virulent, they will kill all the host cells, if they are too infective and not virulent enough, they will exhaust the number of host cells. In either case, the virus particles will be eliminated from the system. For this reason, if scientists want to utilize respiratory viruses as gene transfer vectors, they must find a way to increases the infectivity. Scientists at the University of Iowa and University of California, Berkeley have done this using natural, evolutionary, techniques of introducing a variety of virus phenotypes into the system and selecting for the virus that is most infective (4). In the first stage of this search they shifted around the capsid genes from two different serotypes of the AAV, AAV2 and AAV5, to see whether they could increase the infectivity of the virus to the host cells. They optimized the viral capsid by subjecting the capisid coding genes to DNA shuffling and error-prone PCR so that extensive variation was observed. They then selected AAV mutants that were effective at gaining entry to the nucleus by having those particles amplified by helper adenoviruses in the cell. They did multiple rounds of this selection and encountered increasing amounts of new serotypes relative to AAV2 (Fig. 4). After five rounds of this selection, they found a variant that was a combination of AAV2 and AAV5, which they named AAV2.5T. When host cells were treated with this “chimera,” transduction and binding both increased when compared to the original AAVs, which indicated that this new variant would be able to efficiently transduce genes into the airway epithelial cells and act as an effective therapeutic agent.
The next stage of this experiment was to determine whether or not this new particle could effectively express the CFTR gene, the defective gene that causes cystic fibrosis, and fix the problematic chloride ion channel. They inserted an effective CFTR gene into the viral genome and saw that the vector was effectively able to bind the target host cells and restore appropriate levels of chloride ion flow in the previously defective cells. Before the introduction of this vector to the system, the CFTR protein product was not visible to the researchers using immunocytochemistry. After the treatment, however, CFTR protein is easily visible and is being produced in the correct location on the epithelial cells (Fig. 5).
AAV serve as an effective vector for this study because the proteins that make up their capsid, the region of the particle that contacts receptors on the host cell, are coded for by one gene. Furthermore, this gene is observed in multiple varieties in the wild, which means that the capsid has evolved and possesses the capacity to be manipulated into an optimized form for the target airway epithelial cells in this study. AAVs are also large enough to carry the CFTR gene, making it the ideal carrier in this case.
This case study is interesting because it discusses the ways in which adeno-associated viruses are developed and used as vectors in real host systems to treat real diseases caused by genetic disorders. It shows how AAVs are mutable and able to be optimized to treat a particular system using natural, evolutionary pressures that restore the infective properties of the virus to optimal levels for therapeutic purposes.
Gene Therapy of Glioblastoma Multiforme Using AAV
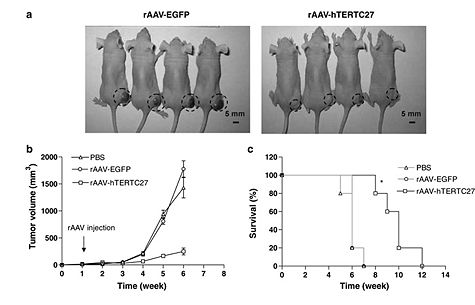
This case study is interesting because it discusses the ways in which adeno-associated viruses are developed and used as vectors in real host systems to treat real diseases caused by genetic disorders. It shows how AAVs are mutable and able to be optimized to treat a particular system using natural, evolutionary pressures that restore the infective properties of the virus to optimal levels for therapeutic purposes.
In most cancers, including glioblastoma, human telomerase reverse transcriptase (hTERT) is reactivated in the cell (5). hTERT is the enzyme that maintains the telomere portions of the genome and is typically only present and functional in cells that are continuously dividing, stem cells, germ cells, and cells for tissue renewal (. Researchers have found that when the 27kDa C-terminal polypeptide, when overexpressed, can cause a dysfunctional hTERT and problems during the anaphase stage of mitosis in HeLa cells, human cells with cervical cancer (5). A dysfunctional hTERT can cause the telomeres of the cancerous cells to rapidly decrease over the course of cell divisions, which can induce senescence. Problems during anaphase can prevent the cell from replicating correctly and reduce the tumorigenesis of the cancerous cells (5).
To test the idea that gene therapy can be effective in targeting and reducing the effects of glioblastoma by causing the overexpression of the C-terminal protein, (C27), the researchers subcutaneously transplanted glioblastoma cells into mice and introduced rAAV-hTERTC27. Mice were injected with glioblastoma cells and after a week, rAAV-hTERTC27 and a control, rAAV-EGFP (recombined AAV containing green fluorescent protein) were injected into mice and tumor size was measured weekly. They found that mice injected with rAAV-hTERTC27 experienced a 80% decrease in glioblastoma cell growth and a twofold increase in median survival time, which proves that the treatment had a positive effect (Fig. 6). Additionally, Cells treated with rAAV-hTERTC27 experienced greater amounts of necrosis, apoptosis, neutrofil infiltration, and microvessel density compared to the control. Cells treated with rAAV-hTERTC27 showed differential gene expression of apoptotic-associated genes, TMSI, MAP3K7IP2, LOXL 2, IGHG1, and Qk1 (5).
The researchers overtly discuss their reasons for using the AAV vector in this study. The first reason is that it has never been known to cause disease. Second, it can infect a large variety of cells regardless of their stage in the cell cycle, something that is very important for therapies targeting cancer. Third, the fact that it can implant its genome into the host cells genome increases the longevity of the treatment, and fourth, because it can deliver high concentrations of transgene expression when coupled to a strong promoter. Ultimately, this experiment has shown that gene therapy is a viable method for treating cancer.
Gene Therapy of Parkinson's Disease Using AAV
Parkinson’s disease is often caused by insufficient formation and action of dopamine that is produced in the dopaminergic (DA) neurons of the brain. It impairs motor skills, speech, and high-level cognitive dysfunction. Two potential causes of the disease are the degeneration of DA neurons and a dysfunctional nigrostriatal (NS) pathway, the pathway that dopamine takes through the brain (9). Both of these complications arise due to genetic disorders in the brain.
Conventional treatment of this disorder revolves around the replacement of dopamine in the striatum, the subcortical part of the cerebrum, but this does nothing to reduce the neurodegenerative nature of the disorder. One therapeutic approach to treating the disorder is by using glial cell line-derived neurotrophic factor (GDNF), the most potent molecule for enhancing the survivability of the DA neurons (9). Simply injecting these molecules into the central nervous system (CNS), however, isn’t all that effective for two main reasons, first it doesn’t have a long active life in that environment, and second, because of its relative inability to cross the blood-brain barrier (BBB) (9). For these reasons, gene therapy has become a viable option of increasing the length and effectiveness of treatment. Treatment of Parkinson’s diseases, however, is complicated because the degradation of DA neurons is already advanced by the time symptoms are perceived in the individual (9). To treat this disease researchers must then show that a particular treatment is able to help correct degradation or slow the degeneration of DA neurons.
To test the idea that delivering GDNF genes into the striatum via AAV vectors can help regenerate DA neurons and the nigrostraiatal pathway, researchers created a recombined AAV with GDNF and the FLAG peptide to form AAV-GDNFflag and tested its effectiveness against striatum tissue 4 weeks after an intrastriatal lesion was created (9).
The first thing they did was recombine AAV with GDNF and FLAG to form AAV-GDNFflag and AAV with B-galactosidase to form AAV-LacZ, which was the control. First, they tested whether or not the AAV-GDNFflag made it to the substantia nigra the region in the brain that contains the dopaminergic neurons that were degraded. After finding out that some AAV-GDNFflag did, indeed, make it to the substantia nigra (SN), they wanted to see whether it had any effect on the intrastriatal lesion. To measure the effects that AAV-GDNFflag had, they measures the density of tyrosine hydroxylase (TH) fibers in both the mouse treated with AAV-GDNFflag and the mouse treated with the control. They found that the TH density in the AAV-GDNFflag treated mouse was 29% greater than in the control, indicating that the introduction of the functional GDNF gene into the striatum cells worked, and that the treatment was effective in rescuing the innervation of the DA neurons in the striatum (Fig. 7). Furthermore, they discovered that the SN was greatly rescued as a result of the treatment (Fig. 8).
These results show that how gene therapy via AAV is effective at treating genetic disorders in the brain. It is able to move to the target and implant its genes into the target cells, eliciting the desired response, which has a long lifespan, and even has the potential to restore damaged tissues that would have otherwise continues to degrade. It is much more effective than superficial treatments of GDNF protein, and provides a relatively long-term solution to a serious problem
Conclusion
Adeno-Associated Gene vectors are both effective within the realm of viral gene vectors and effective within the realm of therapeutic means for treating diseases and disorders. They are highly versatile, as seen in each of the case studies presented above. They can treat rapidly dividing cancer cells, as well as stable lung and brain tissue cells. They can be manipulated to be specific to particular targets and they are able to infect cells without incurring any damage to the cell through immunological responses. AAV vectors are able to impose lasting, thorough, change within the molecular biology of the target cell, which is certainly a lot better than superficially treatments, such as when GDNF molecules are injected into the CNS to treat Parkinson’s. Gene therapy, in that case, is more effective at reaching the target, and also at creating a lasting, regenerative, response within the host. AAV vectors are fantastic instruments of molecular biology and hopefully their use pushes the boundaries of medicine so that more diseases and disorders can be effectively cured and treated in the future.
References
2. “Vector Biolabs - Introduction to AAV.” 2007
6. “AAV Vectors.” Baylor College of Medicine. 2007.
8. UNIBS. "Heparan Sulphate Proteoglycans." UNIBS.
10. "Recombinant Adeno-Associated Virus (rAAV) Vectors"
Edited by student of Joan Slonczewski for BIOL 238 Microbiology, 2009, Kenyon College.