Borrelia burgdorferi- The Cause of Lyme Disease
By Maggie Williard
Introduction
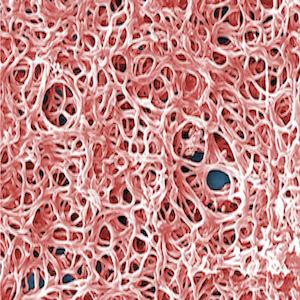
Borrelia burgdorferi is an ancient spirochete-shaped bacteria that is responsible for Lyme disease [1]. These bacteria symbiotically live within the bodies of ticks, benefitting from this relationship, whereas the ticks do not. Borrelia burgdorferi is a part of the Spirochaete phylum, which is distinctive for bacteria that have a spirally shaped body and flagella that are found in the periplasmic space between the inner and outer membranes. Borrelia burgdorferi are excellent swimmers, and are able to navigate their way from ticks to new, vertebrate hosts such as small mammals, birds, and lizards. Oftentimes, ticks in their larval feeding phase pick up Borrelia burgdorferi from rodents infected with the bacteria. As the ticks grow into their adult phase, they eventually target only large mammals, infecting them with Borrelia burgdorferi as they feed. Interestingly, Borrelia burgdorferi are almost never transferred transovarially [1]. Humans typically obtain Borrelia burgdorferi and Lyme disease as a result from nymphal ticks, because they are smaller and more difficult to spot than adult ticks, allowing the nymphs to feed for longer periods of time. Lyme disease can cause an array of symptoms in patients, the most infamous being the distinctive bullseye pattern rash that appears on the skin, called erythra migrans. Because Borrelia burgdorferi is transmitted via ticks, Lyme disease is most prevalent in rural areas, where ticks can be more commonly found [1]. In the United States, Lyme disease can most commonly be found in New England, mid-Atlantic states, upper north-central states, and in some areas of California in the northwest [2]. Furthermore, this infection is seasonal, when ticks are the most active and most likely to come into contact with humans. Research on Lyme disease has been heavily prioritized, with more than $3.5 million per year granted by the CDC to fund new research. In order to better understand Lyme disease and its impacts, it is important to recognize the unique characteristics of Borrelia burgdorferi. Analyzing the remarkable genome of this species of bacteria reveals a lot about how these bacteria function, and which processes they undergo to ensure their survival. In addition to this, it is relevant to look at the type of metabolism Borrelia burgdorferi carry out, and the relationship between the bacteria and their host. Moreover, the morphology of these bacteria can provide insight into how motility is achieved. Finally, the relationship between chemotaxis and motility can show how and why Borrelia burgdorferi seek out certain stimuli in their environments.
Lyme Disease
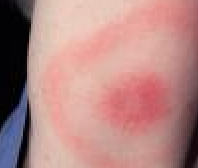
Lyme disease is the prevailing arthropod-borne illness in the United States, and was first characterized as an infectious illness in 1977 [1] [3]. It is estimated that 476,000 people living in the United States are diagnosed and treated for Lyme disease each year [4]. Lyme disease is transferred from blacklegged ticks (Ixodes scapularis and Ixodes pacificus) to humans. For Borrelia burgdorferi to transmit from tick to host, 36 hours of feeding by the tick are required [2]. The process by which Borrelia burgdorferi is transmitted from tick to host is widely speculated. Some researchers believe that transmission occurs through infectious saliva while the tick feeds on the blood of the host organism. On the other hand, some scientists are convinced that transmission may only occur during the regurgitation of fluids from the gut that occurs during the feeding of these ticks [5]. No matter which way the bacteria is transferred, the consequences of this pathogen remain quite uncomfortable. Symptoms of this infection include: rash, fever, headache, and fatigue. The rash, erythema migrans leaves bullseye patterns on the skin of infected individuals, and often serves as a clear indicator of Lyme disease [6]. This disease can progress through three stages: early localized infection, disseminated infection, and late disseminated infection. In the first stage symptoms include erythema migrans, fever, fatigue, headache, malaise, artralgias, and myalgias. In the second stage, which takes place anywhere from days to weeks after the first stage, is the presence of musculoskeletal, neurologic, and cardiovascular symptoms, with legions of erythema migrans. The final stage includes symptoms of pain and swelling of joints and neurologic manifestations such as encephalopathy and neuropathy. Standard treatment for this infection involves a course of antibiotics, typically doxycycline, amoxicillin, cefuroxime axetil, or erythromycin. Additionally, more severe cases utilize ceftriaxone or penicillin G, intravenously [2]. Diagnosis for Lyme Disease is fairly straight forward. Within a month of the onset of symptoms, blood is drawn from patients suspected for the illness, usually patients displaying erythema migrans along with other symptoms. Blood samples are then tested for immunoglobulin G antibodies and immunoglobulin M antibodies via the Western blot technique. However, patients who have had the illness for longer than one month have their blood drawn and tested for only immunoglobulin G antibodies, via Western blot [7].

A hot topic in the world of medicine today is whether or not chronic Lyme disease exists. In fact, leading infectious diseases and neurological academic societies do not acknowledge chronic Lyme disease as a true clinical being. This is disputed by other professional organizations that have been fighting for the acceptance of chronic Lyme disease, by focusing on diagnosis via symtomology rather than by diagnosis that is purely serological [8]. It has been documented that many patients diagnosed and treated for Lyme disease have ongoing symptoms despite treatment. The list of symptoms for chronic Lyme disease is extensive, including: fatigue, night sweats, arthalgia, myalgia, arrhythmias, nausea, diarrhea, abdominal pain, trouble sleeping, cognitive dysfunction, headache, backpain, and depression. Because many groups do not recognize chronic Lyme disease, patients who seek help do not receive much attention or care. For physicians who do recognize chronic Lyme disease based on symtomology or unvalid testing methods, the treatment plan is incredibly variable. Some patients may be treated with several of rounds of different antibiotics for up to several years. This treatment can be quite harmful to both the patient, and towards its potential contribution to generating bacteria that are resistant to antibiotics. A study on primary care physicians in Connecticut found ~2% recognize and diagnose chronic Lyme disease, whereas the rest reported that they did not believe or were hesitant of the existence of the chronic illness [8]. There is a large need for more research to be conducted on this topic so that patients may receive the care and attention that they need, in addition to the avoidance of unnecessary treatment options through the use of antibiotics that are harmful in many different aspects.
Genome and Metabolism
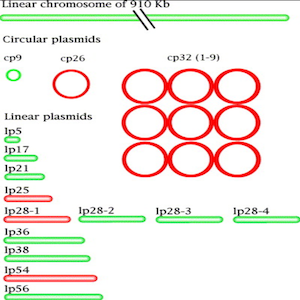
Borrelia burgdorferi has a unique genome from other different types of bacteria. First, these bacteria have linear chromosomes, containing 911 kb. Furthermore, Borrelia burgdorferi have 21 circular plasmids that span 9 to 58 kb. Overall, these bacteria have a genome of a little less than 1,250 kb [10]. Borrelia burgdorferi protects its linear chromosomes from DNA-degrading enzymes through the use of covalently closed hairpin ends. In order to protect the genome, these hairpin ends lock in both the 5’ and 3’ ends of the chromosome. Interestingly, out of the 853 genes that the chromosomes of Borrelia burgdorferi hold, not a single one codes for biosynethtic reactions for these bacteria [10]. It is commonly believed that this seemingly harmful absence of metabolic capacities could be owed to the loss of genes via convergent evolution [11]. Because of this these bacteria have minimal metabolic capabilities, forcing them to rely on the metabolic pathways and nutrients of their host in order to survive [12]. Interestingly, over 6% of the chromosonal genes within Borrelia burgdorferi play a role in chemotaxis and motility. Furthermore, gene expression is altered depending on the environment that the bacteria is residing in, allowing them to adapt to different conditions. This is useful to Borrelia burgdorferi as these bacteria constantly transfer from tick to new host, which oftentimes vary in both temperature and pH [1].
As with all bacteria, Borrelia burgdorferi must adapt to its everchanging environment. Because these bacteria inhabit the midgut of ticks, they must be able to withstand blood flowing in from the prey of the ticks into the gut. In response to this, these bacteria will synthesize outer surface protein C, which directs Borrelia burgdorferi to the salivary glands of the ticks. This is a great example of alteration of protein expression. Furthermore, Borrelia burgdorferi downregulates the synthesis of the protein that anchors the bacterium to the midgut, outer surface protein A [13] [14]. These changes in protein synthesis allow Borrelia burgdorferi to disconnect from the midgut and enter the salivary glands, allowing the bacteria to navigate towards the tick host, so that it may transfer from the tick to a new home. Once Borrelia burgdorferi enters its new host, certain parts of its genome may ensure greater survival. For example, it has been found that Borrelia burgdorferi that lacks its 24.7-27.5 plasmids and 9kb have a lesser degree of infectivity than their counterparts that do have these parts of its genome intact [15]. It has been speculated that this part of the genome of Borrelia burgdorferi may encode for genes that are resistant to the antibodies of the host. Furthermore, Borrelia burgdorferi must protect itself from spikes in oxygen levels while ticks feed on blood [14]. This exposure to high levels of oxygen can be toxic to Borrelia burgdorferi. In order to deal with this, these bacteria upregulate the BB0728 gene, which codes an NADH oxidase enzyme. NADH oxidase may help these bacteria adapt to the large amounts of oxygen entering the environment, protecting the cells from toxicity as a result of oxygen [14].
Although Borrelia burgdorferi has incredibly limited metabolic capabilities, it still undergoes rudimentary forms of metabolism to propel itself within the gut or bloodstream of its host. Homologs of the metabolic pathway of glycolysis have been identified through genomic analysis of Borrelia burgdorferi [16]. It is thought that carbohydrates are solely metabolized via glycolysis in these bacteria. These carbohydrate sources include glucose, glycerol, chitobiose, maltose, mannose, and N-acetylglucosamine. Glycolysis is commonly thought to be the only pathway in Borrelia burgdorferi that generates ATP, resulting in the slow generation time of 8.26 to 12.36 hours, depending on temperature [16] [17]. Borrelia burgdorferi has been found to increase yields of ATP through glycolysis from 2 to 3. In these bacteria, pyrophosphate is used rather than ATP to fuel the function of phosphofructo-1-kinsane, which is the enzyme that limits the rate of glycolysis [17]. Because of this, Borrelia burgdorferi saves the ATP, increasing its net of ATP by 1.
Morphology and Motility
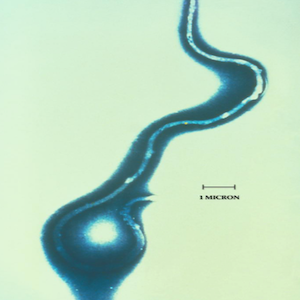
Borrelia burgdorferi is a part of the spirochete phylum, lending to its high motile abilities. Compared to other bacteria, Borrelia burgdorferi can be considered quite big in size, as their length ranges from 10 to 20μm with a diameter of 0.33μm [18]. Physically these bacteria are quite flat with ripples down the body at a measured wavelength of 2.83μm and an amplitude of 0.78μm. Borrelia burgdorferi has a membrane sheath that constitutes as the outermost layer of these bacteria, and houses the periplasmic flagella and the cell cylinder. The periplasmic flagella can be found within the gap of the periplasma and are attached at the ends of the cell cylinder. Major filament protein FlaB and minor filament protein FlaA constitute a range of 7 to 11 periplasmic flagella that are helically shaped and meet up in the middle of the bacterium. Each periplasmic flagella is comprised of a motor, filament, and hook. FliF along with other proteins comprises the motor, while FlgE makes up the hook, and proteins FlaB and FlaA make up the filament [19]. FlaB constitutes 10 to 14% of the total protein in the cell, while FlaA constitutes less than 0.5% of the total protein in the cell. FlaA of Borrelia burgdorferi is located next to the hook, forming a sheath that surrounds the center of FlaB. The gene for FlaB is expressed constitutively and is located on the large linear chromosome. The flagella generate waves that move backwards, allowing the bacteria to swim in many different types of habitats, including both viscous and low viscosity environments [18]. It has been found that in addition to enabling the motility of these bacteria, the flagella also play a role in conserving the morphology of Borrelia burgdorferi with its flat waves. These bacteria are able to move in three different ways: swim, reverse, and flex [20]. It is widely speculated that the rotation direction of the flagella at each end of these bacteria enables Borrelia burgdorferi to swim in many different fashions. Smooth swimming occurs when the flagella at different ends of the cell rotate in the opposite directions of each other. Borrelia burgdorferi can change its direction by switching the direction of motion of the flagella at the opposite ends from one another.
The morphology of Borrelia burgdorferi is incredibly relevant because it helps determine how well the bacteria can move. For a bacteria that must cross through tissues and pass from one host to another this is quite crucial. Furthermore, Borrelia burgdorferi must be able to outmaneuver the immune cells of the host to avoid its destruction [19]. Borrelia burgdorferi with FlaB mutants are nonmotile cells that are shaped like a rod. These mutant bacteria are unable to infect hosts, and have low viability within healthy ticks. The spirochete shape of Borrelia burgdorferi allows them to move, and ultimately enables success in these bacteria. Healthy Borrelia burgdorferi have been reported traveling at velocities anywhere from 10 to 100 times faster than immune cells within the host, lending to their infectivity and survival.
Chemotaxis plays an important role in governing the motility of Borrelia burgdorferi, directing the bacteria to and from different locations within hosts. Borrelia burgdorferi has been observed to swim towards rabbit serum, an example of positive chemotaxis [20]. This serves as a mechanism that allows these bacteria to identify new hosts and begin their journey to them from the midgut of a tick. However, Borrelia burgdorferi swims away from substances such as ethanol and butanol, serving as repellents to these bacteria. Chemotaxis is not identified in Borrelia burgdorferi in the presence of amino acids and sugars. Conditions that mirror that of interstitial fluids such as pH 7.6 and a high concentration of NaCl are required for the mobility of these bacteria. Therefore, it is relevant that Borrelia burgdorferi use chemotaxis to guide itself to the right conditions to allow for motility, enabling their survival. Interestingly, in an experiment, motile Borrelia burgdorferi form cysts when incubated under conditions lacking rabbit serum [21]. Moreover, these bacteria were left fully non-motile, with cyst formations after 48 hours of starvation. Borrelia burgdorferi also responded to these conditions by inducing the synthesis of more than 20 proteins, and many of these proteins were labeled as antigenic. In this case, chemotaxis not only affects motility, but can even alter morphology, under certain conditions of starvation. Unsurprisingly, genetics play a large role in regulating the chemotaxis of Borrelia burgdorferi. More specifically, the response regulator protein, CheY, plays a large role controlling chemotaxis [22]. Here, CheY must be dephosphorylated in order for chemotaxis to function properly, and in Borrelia burgdorferi, a homolog of phosphatase CheX plays the role of dephosporylation. Mutants lacking CheX were observed flexing, but not swimming forwards or backwards. Here, chemotaxis is necessary for the motility of Borrelia burgdorferi, where significant movement cannot occur without properly functioning mechanisms of chemotaxis.
Conclusion
Recently Borrelia burgdorferi has been a hot topic of interest in the world of research. The unique genome comprised of both linear chromosomes and circular plasmids alone provides enough incentive to take a closer look at these spirochetes. Furthermore, the diversity of the different environments that these bacteria must endure as they switch from host to host make up an interesting story that scientists can examine. More specifically, the intricate genomic regulation in response to various stimuli from the act of switching and settling into new environments, or new hosts, is fascinating. Borrelia burgdorferi must be able to adapt to new temperatures, new pH, and new challenges such as the presence of threatening immune cells as they progress through their life cycle, and through new hosts. Moreover, these bacteria serve as a great model to explain the structure function relationship. The spirochete shape of Borrelia burgdorferi provides these bacteria with the incredible ability to reach astounding speeds, through the presence of several well organized flagella. Motility is prioritized in these bacteria over many other basic functions, such as metabolism. These bacteria are able to get away with such a simple form of metabolism because their environment within their hosts provides them with any necessary nutrients that they might need. Examining this relationship between Borrelia burgdorferi and host further can reveal much more about not only these bacteria themselves, but can also provide answers as to why these bacteria evolved the way they did. This can then be extended for exciting new research about Lyme disease. There is much more work left to do in examining any longer impacts that Lyme disease may have on patients who report persisting symptoms that follow treatment. From this research, individuals suffering from chronic Lyme disease may be able to obtain answers for their symptoms. This great debate over the existence of chronic Lyme disease may only be solved through future research, resolving any inconsistencies in patient care and in the practices of physicians. It is incredibly important that a general consensus be found regarding treatment for these symptoms, as to avoid additional contributions to a very large problem today, antibiotic resistance. After acquiring more knowledge about symptoms following Lyme disease, patients may be able to get more help, through safe and effective treatment. Through this research, scientists may even gain additional knowledge about the inner workings of Borrelia burgdorferi itself, and its resulting impact on many individuals, Lyme disease.
References
- ↑ 1.0 1.1 1.2 1.3 1.4 Tilly K, Rosa PA, Stewart PE. 2008 Biology of Infection with Borrelia burgdorferi. Infectious Disease Clinics of North America 22, 217–234.
- ↑ 2.0 2.1 2.2 Bratton RL, Whiteside JW, Hovan MJ, Engle RL, Edwards FD. 2008 Diagnosis and Treatment of Lyme Disease. Mayo Clinic Proceedings 83, 566–571.
- ↑ In press. Electrotransformation of the Spirochete Borrelia burgdorferi | SpringerLink.
- ↑ Kugeler KJ, Schwartz AM, Delorey MJ, Mead PS, Hinckley AF. 2021 Estimating the Frequency of Lyme Disease Diagnoses, United States, 2010–2018. Emerg. Infect. Dis. 27, 616–619
- ↑ Burgdorfer W, Hayes SF, Corwin D. 1989 Pathophysiology of the Lyme Disease Spirochete, Borrelia burgdorferi, in Ixodid Ticks. Clinical Infectious Diseases 11, S1442–S1450.
- ↑ Dandache P, Nadelman RB. 2008 Erythema Migrans. Infectious Disease Clinics of North America 22, 235–260.
- ↑ In press. Diagnosis of Lyme Disease - American Family Physician.
- ↑ 8.0 8.1 In press. Experiences of patients identifying with chronic Lyme disease in the healthcare system: a qualitative study | BMC Primary Care | Full Text.
- ↑ Stewart PE, Byram R, Grimm D, Tilly K, Rosa PA. 2005 The plasmids of Borrelia burgdorferi: essential genetic elements of a pathogen. Plasmid 53, 1–13.
- ↑ 10.0 10.1 [Slonczewski, J.L., Foster, J.W., Zinser, E. (2020). Microbiology An Evolving Science. New York : W.W. Norton & Company.]
- ↑ Fraser CM et al. 1997 Genomic sequence of a Lyme disease spirochaete, Borrelia burgdorferi. Nature 390, 580–586.
- ↑ Kerstholt M, Netea MG, Joosten LAB. 2020 Borrelia burgdorferi hijacks cellular metabolism of immune cells: Consequences for host defense. Ticks and Tick-borne Diseases 11, 101386.
- ↑ Anguita J, Samanta S, Revilla B, Suk K, Das S, Barthold SW, Fikrig E. 2000 Borrelia burgdorferi Gene Expression In Vivo and Spirochete Pathogenicity. Infect Immun 68, 1222–1230.
- ↑ 14.0 14.1 14.2 Tokarz R, Anderton JM, Katona LI, Benach JL. 2004 Combined Effects of Blood and Temperature Shift on Borrelia burgdorferi Gene Expression as Determined by Whole Genome DNA Array. Infect Immun 72, 5419–5432.
- ↑ Xu Y, Kodner C, Coleman L, Johnson RC. 1996 Correlation of plasmids with infectivityof Borrelia burgdorferi sensu stricto type strain B31. Infect Immun 64, 3870–3876.
- ↑ 16.0 16.1 In press. Borrelia burgdorferi hijacks cellular metabolism of immune cells: Consequences for host defense - ScienceDirect.
- ↑ 17.0 17.1 Heroldová M, Němec M, Hubálek Z. 1998 Growth parameters of Borrelia burgdorferi sensu stricto at various temperatures. Zentralblatt für Bakteriologie 288, 451–455.
- ↑ 18.0 18.1 Motaleb Mohammed Abdul, Corum Linda, Bono James L., Elias Abdallah F., Rosa Patricia, Samuels D. Scott, Charon Nyles W. 2000 Borrelia burgdorferi periplasmic flagella have both skeletal and motility functions. Proceedings of the National Academy of Sciences 97, 10899–10904.
- ↑ 19.0 19.1 Sultan SZ, Manne A, Stewart PE, Bestor A, Rosa PA, Charon NW, Motaleb MA. 2013 Motility Is Crucial for the Infectious Life Cycle of Borrelia burgdorferi. Infection and Immunity 81, 2012–2021.
- ↑ 20.0 20.1 Shi W, Yang Z, Geng Y, Wolinsky LE, Lovett MA. 1998 Chemotaxis in Borrelia burgdorferi. J Bacteriol 180, 231–235.
- ↑ Alban PS, Johnson PW, Nelson DR. 2000 Serum-starvation-induced changes in protein synthesis and morphology of Borrelia burgdorferi. Microbiology 146, 119–127.
- ↑ Motaleb MA, Miller MR, Li C, Bakker RG, Goldstein SF, Silversmith RE, Bourret RB, Charon NW. 2005 CheX Is a Phosphorylated CheY Phosphatase Essential for Borrelia burgdorferi Chemotaxis. J Bacteriol 187, 7963–7969.