Countermeasures of Bacillus anthracis use as a Biological Weapon
Biological Weapon Overview

Biological Weapons with varying degrees of lethality have been used for many centuries and deliver toxic classifications of microorganisms such as bacteria and viruses in such a manner that causes widespread disease among many different living organisms. While intentional infection of animals and agriculture can be fear-inducing and economically detrimental, it is the infliction of disease within the human race itself that can have an extremely dramatic effect on a country’s ability to function. The use of such weapons started off in a rather elementary and blunt fashion. For example, in 1346, citizens of Kaffa (Located on the Crimean Peninsula) were attacked by the invading Tartar army via exposure to plagued corpses leading to widespread of illness.[3] In a sense one could argue that human history has been shaped by microbial infection, as implied by Paul F. Torrence [15]. We have now begun to experience much more subtle, but increasingly efficient uses of biological weapons, as displayed in the anthrax letter attack on multiple media and news offices and two United States senators in 2001, where 17 people were infected and five were killed as a result of exposure to anthrax spores(Image 1). [4]
While some may underestimate the possible impact of biological weapons relative to that of other weapons of mass destruction, such attacks should not by any means be taken lightly [15]. A large factor as to why people do not necessarily see the potential for danger is due to the fact that the impact is more often than not initially undetectable, and even the full potential of exposure can be delayed since most weapons of this nature can live outside of a host organism for long periods of time while dormant. As such, biological weapons can be transmissible and can travel from one infected person to another; perpetuating the spread of the virus. In conjunction, these pathogens require a certain incubation period that varies from one pathogenetic species to another, so it is quite likely that an infected individual can transfer the disease before they are aware that they are affected.
Clearly, it is becoming increasingly necessary to take precautious measures against the spread of these disease-spreading biological weapons. There are a couple international policies currently in place to help in the prevention of their use: The 1925 Geneva Protocol prohibits the use of biological weapons in warfare while the 1972 Biological and Toxin Weapons Convention places restrictions on production, development, and acquisition of biological agents with malicious intentions. [3] While these are legitimate means of prevention, it is equally critical if not more important to undertake means of treatment post-infection since the increasing availability of biotechnology is making the development and dissipation of dangerous microorganisms much easier than ever before.
As foreseeable, there are a seemingly endless number of microorganisms that can be used maliciously. The selection of a biological weapon is dependent on the type of attack desired, the pathogenicity of the organism, and the incubation period (amount of time observed between exposure and symptomatic illness expression) [2]. Due to the nature of biological attacks and terrorism, some toxins are more desirable to the attacker than others. One pathogen that is especially effective is anthrax, whose etiological agent is Bacillus anthracis.
Bacillus anthracis
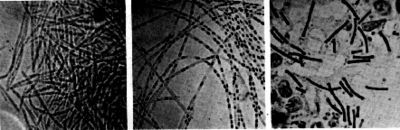
Bacillus anthracis has developed as a biological weapon and bioterror agent as a result of this specific bacterium's toxicity in its vegetative form, and its ability to produce spores that are tolerable of many extreme environments. This bacillus became the first bacterium to be directly linked to causing disease when scientist Robert Koch acquired a pure culture and successfully produced anthrax experimentally [5]. Koch proved the causative link to the disease by implementing his widely accepted postulates, for which he was awarded the Nobel Prize in Physiology or Medicine in 1905 [5].
The anthrax bacillus is a relatively large organism measuring 1-1.2 micrometers in width and 3-5 micrometers in length. This specific bacillus can be distinguished from similar bacterium such as B. thuringiensis and B. cereus by its characteristic square-shaped ends, and centrally located ellipsoidal endospore which is associated with production of an intracellular parasporal crystal (a protein crystal)[7]. B. anthracis is a Gram-positive rod with spore forming capabilities. It is these spores formed by the bacterium that are what enable this species to be effective as a biological threat if incidentally brought into contact.
Endospores
Since the bacterium in classified as a Gram-positive organism, this allows for interestingly durable spore formations. The spores produced are resistant to relatively extreme environmental conditions and because of their thick cell walls with a high amount of peptidoglycan, they have the ability to potentially exist in a dormant state for years and even decades [8]. Even when only a small number of spores are present at the time of exposure, the spores will then germinate into their vegetative state where there exists a period of very rapid growth. This rapid growth stage typically results in host fatality due to the high concentration of toxins.
Pathogenesis
Human infection can occur when the host is exposed to the spores of the bacterium via one of more of three routes: cutaneous, gastrointestinal, or inhalation. Cutaneous contraction of the disease typically occurs through open skin wounds coming into contact with another infected organism/ part of organism. This is generally the least severe form of the disease. Gastrointestinal contraction typically results from consumption of undercooked meat that contains either the vegetative form or dormant spores of the disease. When air bourne spores are inhaled, a respiratory infection can result. Gastrointestinal and respiratory infections are historically the most letal and lead to the highest mortality rates [12].
Inhalation anthrax
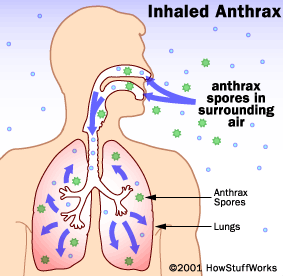
Inhalation anthrax is the most common way in which B. anthracis is used as bioterrorism weapon. It is very dangerous and is the fatality rate if not treated early is much higher than by either of the alternate two types of anthrax. When the spores are inhaled, they quickly adhere to alveolar macrophages and begin their germination process. The bacteria then make their way to the lymph nodes and begin a process of rapid replication [13]. A tripartile toxin composed of a protective agent (PA), Zn2+ metalloproteinase lethal factor (LF), and a adenylate cyclase edema factor (EF)[13] is excreted by the bacterium. (A more detailed description of each factor can be found in the following Anthrax Toxin section.) PA, LF, and EF interactions and activity within the cell kills hosts macrophages, and as a results leads to large impact on the immune system as a whole which gets continually worse as the disease continues until death results.
Anthrax Toxin
It was not until 1954 that the actual toxigenic properties of B. anthracis were observed. Originally, it was observed that in most cases of animal death from the disease, 109 bacteria/ml were present, leading scientists to conclude that strictly the large number of bacteria caused capillary blockage by a theory known as "log-jam". Experimentally, on the other hand, clinical testing showed that only 3x106 bacteria/ml were actually needed to inflict death.[4] This discovery indicated that some sort of diffusible exotoxin was responsible for anthrax's level of pathogenesis; this exotoxin being the durable spore formed by the bacterium.
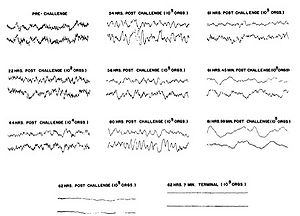
In a study of the neurological and physiological responses of primates to the anthrax toxin (shown in Figure 1), the apparent physical cause of death of an infected organism is due to a sharp decrease in the cellular oxygen level, which then leads to secondary shock. This secondary shock increases the vascular permeability where partial to complete loss of cortical electrical activity (EEG) is observed in the toxin-challenged primate. A couple hours before death, another increase in EEG activity is observed in conjunction with anoxic hypertension and eventually a complete collapse of the cardiovascular system. It was concluded that the extreme effect on the cardiovascular system is a results of direct impact of the toxin in the depth of the brain relating to the respiratory system by observation of intact junction of the phrenic and neuromuscular nerve [9]. It has also been shown that death often occurs unexpectedly and rapidly as a sharp increase in the level of lethal factors is observed during the later stages of the disease [4].
The primary virulence toxin in B. anthracis is contained in a temperature-sensitive plasmid, pX01 which contains three different antigenetic factors [4]. Each of the following are proteins with a molecular weight of about 80kDa:
Factor I: is known as the the edema factor (EF) which is an adenylate cyclase that elevates intracellular cAMP levels and is calmodulin-dependent [10]. It is an inherent adenylate cyclase and is necessary for the edema production activity of the toxin [4]. Edema is swelling as a results of fluid build up in the body's tissues [11].
Factor II: is the protective antigen (PA) and is the binding domain of the toxin. It has two domains; one for the EF and one for the lethal factor [4], which will be mentioned next. This factor is essential for the effectiveness of the toxin, since it serves as the binding site of the cytoplasmic targets for the other two factors. Without the PA factor, the other factors will not be able to recognize their cellular targets or have means of transportation into the cytoplasm of the host from the extracellular space[10].
Factor III: is a zinc-dependent metalloprotease toxin known as the lethal factor (LF) whose figure is shown in Image 4. [8] that targets all but one APK kinases [10]. Its lethality to the host cell is through the means of secretion into the cell where it then causes signaling pathway disruptions, cell destruction, and impinges shock on the circulatory system [8].
Individually, these factor do not have any effect on the host, but different combinations of the factors yield some interesting results. When the PA is present with the LA, the two factors can combine together to produce lethal activity since the PA serves as transportation and recognition of the host's protein receptor molecules which the LA uses to enter the cell and infect the organism. When the edema factor (EF) is present with the PA, edema is results for reasons comparable to those for the PA and LF combination since the PA also serves as the binding domain of the toxin. The pairing of EF and LF does not have any effect on the cell since the two factors do not have any way to gain access to the cytoplasm of the cell from the extracellular location without the assistance of the PA's binding domain and site-recognition assistance. When all three factors are present together, the infection is in full scale and is highly effective in the host organism [4]. As mentioned, Koch's Postulate was used to correlate the bacterium with the disease, but this postulate does not take into consideration smaller aspects of the actual toxins responsible for the disease. It may be that if a B. anthracis cell were to be identified in an organism but not contain all three necessary factors, then the organism would not be infected. This is a possible problem with using Koch's postulate theory, since a person may have the necessary pathogen for a disease, but not actually be infected if every aspect of the causative agent is not present.
The combination of EF and PA has been shown to induce an elevation of cyclic AMP to extreme levels, making the cells very susceptible due to changes that result in the membrane and its permeability leading to edema. When all three factors are present, the transport of the EF and LF factors into the cell result in a depletion of the hosts' ATP. This leads to the possibility that one of the factors may need to be present to hinder the ability of the host to engulf other local particles so that it has enough energy to engulf the toxin during the infectious stage.
Lethal Factor Inhibition
After the onset on the anthrax disease there is clearly not much that can be done in terms of prevention means except for prevention of further spreading. For that matter, other means of dealing with the actual bacterium and endospores themselves is necessary. There are a variety of ways which scientist have found to kill the bacterial infection in the human host and also ways to prevent the infected individual from being able to transfer the disease to healthy individuals. Most of the treatments for weaponized anthrax is via antibiotic treatment. Treatment must begin as soon as detected to prevent really extensive damage and if it is caught quickly enough the symptoms should remain mild and are generally flu-like [8]. Currently, antibiotic options to combat the infection are relatively readily available, unfortunately though there are no realistic options available to reverse any of the bodily destruction during the infection or damage done once it has been eliminated by the antibiotic treatments [8].
In an experiment performed at Merck Research Laboratories or New Jersey, it was found that an LF inhibitor (LFI) antibiotic could be used to halt the protection of the macrophage, which would allow the host cell to be able to eliminate spores of the bacterium in the early stages of the infection. Another method of inhibition of the LF while an infection is active was to use an LFI to prevent the later stage effects of the Lethal factor. Using this method along with addition antibiotic treatments. In their research, use of the crystal structure of a particular LFI, hedroxamate LFI to observe the interactions of the inhibitor with the lethal factor at the cellular level.
Methods
The LFI and recombinant toxins used for all the related studies performed were held constant. The hydroxamate LFI was specifically (2R)-2-[(4-Fluoro-3-methylphenyl)sulfonylamino]-N-hydroxy-2-(tetrahydro-2H-pyran-4-yl)acetamide. And the recombinant toxins (Lethal factors) used were isolated and purified from Escherichia coli and compared with the LF isolates from B. anthracis. The LFI used displayed identical inhibition between the two LF sources. The recombinant PA factor was also purified from Escherichia coli.
X-ray crystallography was used to obtained truncated LF and LFI complexes via vapor diffusion method where orthorhombic crystals were obtained. The graphical rebuilding of the models for comparison was performed on a computer-based refinement and rebuilding model.
A model of toxemia in mice was created with recombinant PA and LF where the mice were split into four groups at random, each with different types of treatment with LFI at 0, 1, 10, or 30 mg three times per day by a saline transport vehicle [8]. For control all the mice were treated intravenously with 100 micrograms of the recombinant PA and 100 micrograms of the recombinant LF. This was done to ensure that there would be at least two of the factors available since each factor by itself does not affect the host.
Experimental Conclusions
Through structure obtained from the X-ray crystallography of the lethal factor in combination with the LFI, the research team was able to design various compounds to inhibit the lethal factors with increased effectiveness. Although the entire LF structure was not represented and lacked its PA-binding domain, other main domains were conserved and the differences between the inhibited and uninhibited molecules were the locations of a couple domains and some conformational changes to the structure. These conformational changes will inhibit the binding function of the LF and therefore decrease the effects of the anthrax toxin through the inhibition of the LF. Figure 2A indicates the locations of the LF:LFI domains, while Figure 2B shows a molecular imaging of the LF around the inhibitor's binding site (Figure 2) [8].
In the mouse toxemia model, testing with LFI as performed to observe its ability to protect the treated mice from the mixture of the recombinant PA and LF, which together are lethal when uninhibited since the PA provides a transfer mechanism and bonding recognition site for the host's receptor proteins to gain access into the cytoplasm for the letal factor so that it may then infect the host. In the group that was given only a saline solution (0mg dosage), none of the mice survived after the allotted time period. On the other hand, the mice to which LFI was administered, some survivorship was observed. In the remaining LFI administration dosage categories, 1mg, 10mg, and 30mg the percent survivorships were 12.5%, 87.5%, and 100%; respectively (Figure 3). Even though there was a high percent of survivorship, the mice in the 10mg treatment showed signs of typical anthrax infection, while the mice with 30mg doses maintained a normal appearance throughout the duration of the treatments [8].
Through their experimentation, the researchers were able to conclude that the interactions at the molecular level between the LF active site and the LFI led to inhibition of the LF protease and resulted in the protection of microphages against the pairing of LF and the PA. They were also able to observe 100% protection against the lethal toxins in mice against the PA and LF (Figure 3)
. In summary, the molecule hydroxamate LF inhibitor has the ability to prevent anthrax infection and could therefore be useful in combating anthrax [8].
Metal-Protease Inhibitors
Bacillus anthracis lethal factors (LF), metalloproteinase, is clearly a very integral aspect of the disease’s three-part toxin mechanism. It was determined that to fully exert its lethal effect, the LF toxin must enter the cell compartment through ubiquitous PA binding to the appropriate extracellular receptors. After being activated by proprotein convertases, a mature PA protein binds to both LF and EF, which are then expelled after endocytosis. After being liberated from the cell they exert their toxicity. Inside the cell, LF cleaves kinases and disrupts host cells' signaling transduction which leads to eventual death. For this reason, the LF factor in particular is being looked to as a key to developing antitoxin pharmaceuticals and treatment regimes. One study on the efficient synthetic inhibitor of anthrax lethal factor explored a fragment-based approach to develop LF inhibitors. The molecular inhibitors that were synthesized were tested and proved to be highly selective against LF in in vitro testing and cell-based assays [13]. These synthetic inhibitors that were developed are very promising with regards to their anti-toxin effecs: although typical human metalloproteinases are very similar to LF in terms of their structure, the synthetic inhibitors do not affect the host's prototypical metalloprotease structures. This makes them very safe for use in humans, especially when used in conjunction with antibiotic ciprofloxin. The effect of these two treatment option led to significant protection against the disease [13].
Experimental Strategy
A starting point for optimizing the synthetic inhibitors was established by identification of weakly binding structure scaffolds. Following this identification, NMR-based enzymatic assays were performed to search for the most potent inhibitors. Conventionally, fluorescence-based assays are used for this type of analysis, but when trying to determine weaker ligands, a lot of interference occurs from the test compounds which are typically used at high concentrations. In opposition, NMR can detect enzyme activity and inhibitions in proteases and in kinases, which is critical when trying to observe the interactions between the two when developing inhibitors for the toxin's metalloproteases. In addition, the wide range of chemical shifts allows for high spectral resolution. This drug-scaffolding analysis was then applied to about 300 compounds that represent most of the scaffolds commonly found in drugs based on their drug-like properties, ease of synthesis, and availability of derivatives. These possibilities for derivatives allow the small library of 300 compounds to represent a much larger chemical space [13].
Results
By analyzing the interactions between different drug-like chemicals and their inhibition properties of B. anthracis, identification of a compound that exerted 50% LF inhibition at a concentration of 140µM was identified and is shown in Figure 4. After identification, exploration of commercially available analogous derivatives that matched that of the isolate identified yielded 22 compounds that also conferred inhibition of the anthrax toxin [13]. When these 22 synthesized compounds were observed via NMR analysis and fluorescence, increased inhibitory activity was observed.
This study allowed for successful identification of an initial LF inhibition scaffold that can be used in drug development. Optimization of the scaffold resulted in a series of phenylfuran-2-ylmethylenerhodanineacetic
acid derivatives with a nanomolar inhibitory activity against LF which can be used in metal-protease inhibitors. This was done predominately by using structure-guided derivations of Zn2+ chelating compounds. In addition to inhibition of B. anthracis, this methodology may also carry implications for other Zn-metal-protease disease, since the overlying concept is protection of macrophages from cytotoxicity [13].
Conclusion
Although the threat for bioterrorism exists, there is really no specific therapy that has been found to be effective for treating inhalation anthrax, which is the most deadly in humans. However, research is being done that gives a much more detailed look at the pathogen’s mechanism of lethality by examining x-ray crystallography images of the pathogen, where it binds to the cell, and possible compounds that may serve as inhibitors at receptor sites. Several different mechanisms to study these inhibitors have been discovered and many more are being explored. Slowly but surely, countermeasures to anthrax and other such biological weapons are surfacing through scientific exploration of interactions between different toxins and host cells, and what types of chemicals exist or can be synthesized to ensure that such diseases can be inhibited, while leaving the host's cells unharmed.
References
[1] ["http://www.textbookofbacteriology.net/Anthrax.html"
[2] Introduction to Biological Weapons. 2010. Federation of American Scientists
[3] “Amerithrax Investigation” Federal Bureau of Investigation, 2010.
[4] [[6] Todar, K. "Bacillus anthracis and Anthrax". Todar's Online Textbook of Bacteriology. 2009.
[5] [Slonczewski, Joan L., J.W. Foster. "Microbiology: An Evolving Science". Koch's Postulates Link a Pathogen with a Disease. W. W. Norton & Company, Inc. New York, N.Y. 2009. p.21-22.]
[6] [http://srs.dl.ac.uk/Annual_Reports/AnRep01_02/anthrax-spores.jpg
[7] [Aronson, Arthur I., D.J. Tyrell, P.C. Fitz-James, and L.A. Bulla Jr. July 1982. "Relationship of the Syntheses of Spore Coat Protein and Parasporal Crystal Protein in Bacillus thuringiensis" Journal of Bacteriology Vol. 151. No.1: 399-410.]
[8] W. L. Shoop, Y. Xiong, J. Wiltsie, A. Woods, J. Guo, J. V. Pivnichny, T. Felcetto, B. F. Michael, A. Bansal, R. T. Cummings, B. R. Cunningham, A. M. Friedlander, C. M. Douglas, S. B. Patel, D. Wisniewski, G. Scapin, S. P. Salowe, D. M. Zaller, K. T. Chapman, E. M. Scolnick, D. M. Schmatz, K. Bartizal, M. MacCoss, J. D. Hermes, William C. Campbell. May 2005. “Anthrax Lethal Factor Inhibition”. Proceedings of the National Academy of Sciences of the United States of America. Vol. 102 No. 22: 7958-7963 .
[9] Klein, F., R. Lincoln, J. Dobbs, B. Mahlandt, N. Remmele, J. Walker. Feb. 1968 "Neurological and physiological Response of the Primate to Anthrax Infection". The Journal of Infection Diseases. Vol. 118 No. 1" 97-103.
[10] Abrami, Laurence, L. Shihui, P. Cosson, S. Leppla, F. Gisou van der Goot. Feb. 2003. “Anthrax toxin triggers endocytosis of its receptor via a lipid raft-mediated clathrin-dependent process”. The Journal of Cell Biology, 2003. Vol. 160: 321-328.
[11] [http://www.nlm.nih.gov/medlineplus/edema.html "Edema". United States National Library of Medicine and the National Institutes of Health. 12 April 2010.
[12] [http://www.jbc.org/content/281/8/4831.full Joyce, Joseph, C. James. Feb 2006. "Immunogenicity and Protective Efficacy of Bacillus anthracis Poly-γ-d-glutamic Acid Capsule Covalently Coupled to a Protein Carrier Using a Novel Triazine-based Conjugation Strategy" Journal of Biological Chemistry. 281: 4831-4843.
[13] Martino Forino†, S. Johnson, Thiang Y. Wong, Dmitri V. Rozanov, Alexei Y. Savinov, Wei Li, Roberto Fattorusso, Barbara Becattini, Andrew J. Orry‡, Dawoon Jung, Ruben A. Abagyan, Jeffrey W. Smith, Ken Alibek§, Robert C. Liddington, Alex Y. Strongin, and Maurizio Pellecchia. "Efficient synthetic inhibitors of anthrax lethal factor." 23 May 2005.
[14] [7]
[15] Torrence, Paul. Antiviral Drug Discovery for Emerging Diseases and Bioterrorism Threats. John Wiley & Sons, Inc. Hoboken, New Jersey. 2005.
Edited by student of Joan Slonczewski for BIOL 238 Microbiology, 2010, Kenyon College.