Hendra Virus
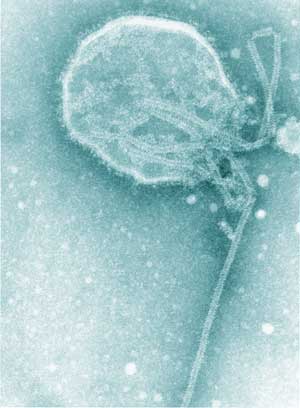
Etiology/Bacteriology
Taxonomy
| Order = Mononegavirales
| Family = Paramyxoviridae
| Genus = Henipavirus
| Species = Hendra Virus
|NCBI: Taxonomy Genome: Genome|}
Description
Hendra Virus is a member of the Paramyxoviridae virus family, which also includes the Measles virus, Canine Distemper virus, and Mumps virus. [1] It shares the genus Henipavirus with Nipah virus, another zoonotic pathogen. [1] Structurally, Hendra virus has a ribonucleoprotein, consisting of a negative sense RNA genome bound to nucleocapsid proteins, contained in a lipid envelope.[2] The surface of the lipid envelope is studded with attachment glycoproteins and fusion glycoproteins that help the virus bind and integrate with the host cells.[2] File:Hendra Virus structure.pdf
Hendra virus first broke out in 1994 at a stable in Hendra, a suburb of Brisbane in Queensland, Australia. In this initial outbreak, the virus killed 13 horses and infected two humans, killing one. [3] There have been several outbreaks of Hendra since then, all concentrated in along coastal areas of Queensland and northern parts of New South Wales in Australia.[4] Flying foxes are considered the reservoir host of Hendra virus, based on the detection of Hendra virus antibodies in the blood of several flying fox species and the discovery of a Hendra-like virus in a healthy flying fox. [5] Hendra Virus has been documented to naturally infect horses, humans, and dogs, but it has infected a wide range of species in experimental research.[6] In horses, an infection is generally characterized by depression, fever, respiratory distress, nervous abnormalities, and a frothy discharge from the nose. [3,6] In humans, a wide range of symptoms have been seen, ranging from flu-like symptoms to pneumonia, encephalitis, and organ failure. [4,7,8] Only two Hendra virus infections have been seen in dogs. In both cases, the animals were asymptomatic but had produced antibodies against the virus.[6] Although the drug ribavirin has been administered to human cases as a treatment and prophylaxis, its effects on Hendra virus are still not known.[4] More encouragingly, an equine vaccine currently available in Australia has proven to be an effective form of prevention.[6]
Pathogenesis
Transmission
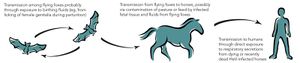
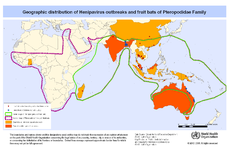
Flying foxes, bats from the genus Pteropus, are considered to be the reservoir hosts of Hendra virus. This is due to to the detection of Hendra virus antibodies in Black flying foxes (Pteropus alecto), grey-headed flying foxes (Pteropus poliocephalus), little red flying foxes (Pteropus scapulatus), and spectacled flying foxes (Pteropus conspicillatus) in 1995.[5] The isolation of a Hendra-like virus from a healthy grey-headed flying fox provided further evidence that flying foxes served as Hendra virus's reservoir. [5] Typically, transmission between infected flying foxes and horses is the first step of an outbreak. The mechanism of transmission is still not completely clear, but several hypotheses have been proposed. In the most plausible, horses become infected by pasture contaminated with flying fox foetal tissues or birthing fluids.[5] This hypothesis was proposed because many Hendra infections fall within the birthing period of flying foxes (August to October), and the virus has been isolated from uterine fluid, foetal liver, and foetal lung of flying foxes in 2000.[7,9] In another proposed theory, horses become infected with the virus when they eat pellets of masticated fibrous fruit discarded by flying foxes.[5] Both of these hypothesis have some plausibility because Hendra virus has been isolated from many other fluids besides uterine fluid, including urine, saliva, and feces.[6] Essentially, it is thought that the horse is infected by feeding on plants contaminated by body fluids or excretions from an infected flying fox or by breathing in the virus while feeding.[6] There is still a great deal to learn about Hendra virus transmission from flying foxes to horses because an infection is technically possible in any region of the flying foxes' geographic range. The fact that outbreaks have been confined to a small region suggests that additional, unknown factors are affecting transmission.
Once a horse become infected, transmission from horse to horse, and from horses to humans, likely occurs through infected body fluids and secretions, although this is still not entirely clear. [4,7] A human or horse could contact the infected fluids directly, such as through invasive procedures like necropsies, or through droplets produced by the respiratory symptoms of the infection.[4]
Currently, the infectious dose of Hendra virus is not known for either horses or humans.[10] However, it is likely that there must be high viral exposure, given the small number of human cases that have been documented. Also, those that have occurred all had close contact with infected or dead horses.
Incubation Period and Disease Progression
Human inoculation with Hendra virus is believed to occur via inhalation of aerosolized infectious fluid.[11] It is also possible that the virus can enter the body through abrasions in the skin, because two fatal cases from the 1994 outbreak had abrasions on their arms and hands while working with infected or dead horses.[8] Incubation period has varied in different outbreaks, ranging from 7-8 days up to 9-16 days in the 2008 outbreak.[4]
Hendra virus first invades the epithelial cells of the lower respiratory tract and progresses to the endothelial cells of the lungs, which allows it to reach the bloodstream. Accessing the blood means Hendra virus can spread throughout the body by circulating independently or binding to leukocytes.[11] Circulating through the bloodstream allows Hendra virus to reach other target organs including the spleen, kidneys, and brain. To infect the brain, the virus must pass through the blood brain barrier.[11] Hendra virus has been shown in experiments to be able to infect the central nervous system directly via the olfactory nerve, which would correspond to a droplet form of transmission. However, since those experiments were conducted on animals with a different physiology than humans, it is not clear whether the virus is capable of using this route in human infections.11]
The disease progression of Hendra virus has varied widely among cases. In the initial 1994 outbreak, one human case had mild symptoms and was ill for 6 weeks, while the fatal case deteriorated quickly and died after 6 days.[3] In a 1994 case diagnosed postmortem, the initial symptoms lasted for 12 days. However, approximately a year later, the patient developed encephalitis and died after 25 days in the hospital.[8] In another case, a veterinarian developed mild, flu-like symptoms that lasted for 8 days.[7] It has not been determined what causes the differences between Hendra virus cases, but it is likely that many factors, such as the amount of virus exposure, the immune strength of the host, and pre-existing health conditions likely play a role. For instance, the fatal case in the 1994 outbreak smoked heavily and showed signs of a Legionella infection at the time of his death.[3] Both of these factors could have weakened his immune system, making it easier for Hendra virus to reach a fatal concentration.
The systematic spread of the virus is clearly indicated by the range of tissues and organs the virus can be detected in. In experimentally infected horses, Hendra virus has been recovered from lymph nodes, lung, kidney, brain and cerebrospinal fluid, as well as nasal swabs, blood, urine, and feces. [10]. In the original 1994 epidemic, the virus was detected in the lung, liver, kidney, spleen, and brain of human fatalities.[8] Additionally, viral antibodies have been detected in the serum of several of those human cases [4, 8]
Clinical Presentation
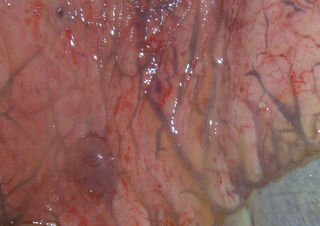
From: Experimental Infection of Horses with Hendra Virus/Australia/Horse/2008/Redlands[10][http://wwwnc.cdc.gov/eid/article/17/12/11-1162-f5
Equine Symptoms
An equine infection of Hendra virus is primarily characterized by respiratory symptoms and neurological symptoms. Infected horses become depressed, develop a fever and have an elevated respiratory rate and heart rate.[3] As the disease progresses, horses can show more pronounced neurologic symptoms such as restlessness, irregular stance, head tilt, muscle spasms, seizures and irritability.[6,10] A characteristic symptom seen in many cases is the excretion of a frothy, occasionally blood-tinged nasal discharge.[10] Histologically, the lungs become edematous, with dilated lymphatic vessels at their margins. Additionally, lung capillaries become degraded, and the foamy fluid discharged from the nostrils is also found in the airways.[10] The spreading of the virus to other areas of the body causes additional histological abnormalities. A horse that died in the 2004 outbreak was found upon necropsy to have cardiomegaly, an enlarged heart, as well as thickened ventricular walls and an enlarged liver.[7]
Human Symptoms
Flu-like respiratory symptoms are typically the first sign of a Hendra virus infection. Much like infected horses, human infections begin with fever, muscle pain, headache, and lethargy, which can last for 3 to 4 days.[3,4,8,11] Depending on unknown factors, the disease course can take several different paths. Some people only have flu-like symptoms, which can last for days or weeks.[3,7] However, in some fatal cases, the respiratory symptoms become more severe, resulting in pneumonia that requires mechanical ventilation. If the virus invades the endothelial cells and disseminates throughout the body, multiorgan failure can occur.[8,11]
Disseminating through the bloodstream allows Hendra virus to invade the central nervous system, likely by crossing the blood brain barrier.[11] There it causes encephalitis, characterized by confusion, ataxia, seizures, ptosis, and other neurological symptoms. In more recent outbreaks, encephalitis after a decrease in respiratory symptoms has been seen in human cases. This represents a marked difference between the recent outbreaks and past outbreaks that were primarily characterized by respiratory symptoms.[4]
Histologically, Hendra virus causes dramatic changes in the tissues and organs. Lungs are typically congested and hemorrhagic with lesions. Microscopically, the tissues are filled with necrotic patches and giant, multinuclear cells have been seen in both the lungs and the nervous tissue.[8,11] In infections where the virus has spread to the endothelial cells of the lungs, the cells were necrotic, which allows the virus to enter the blood stream.[11] The neural tissue of encephalitic patients is characterized by widespread lesions in the brain, plaque formation in the grey and white matter, thrombosis, necrosis of the brain tissue, edema, inflammation, and deterioration of the blood vessels.[4,11]
Epidemiology
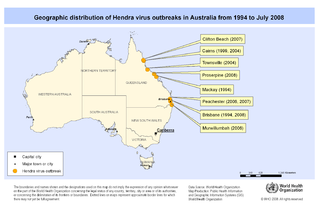
All of the Hendra virus outbreaks have occurred along coast regions of Queensland and New South Wales in Australia, with at least 29 equine cases and 7 human cases as of 2008.[4] Hendra virus antibodies have also been detected in dogs in two separate incidents, in 2011 and 2013, but the animals themselves were asymptomatic.[5] The overall fatality rate is high, about 65% for horses and 57% for infected humans.[3,13] Most of the human and equine Hendra infections (about 70%) occur during August to October, the birthing period of flying foxes.[8,9] However, the virus has not been continuously detected in flying fox populations, and there appears to be periods of high excretion of the virus balanced by periods of low excretion.[6] This means that it is technically possible for Hendra virus to occur at any point in the year, so long as an infected flying fox and a susceptible horse manage to interact so that Hendra virus is transferred. The fact that Hendra virus infections primarily occur at specific times of the year suggests that other, unknown factors are likely affecting transmission.
Although Hendra virus has only been documented to infect horses, humans, dogs and flying foxes (the reservoir species) naturally, the virus exhibits a wide host range in research. Cats, pigs, hamsters, ferrets, African Green Monkeys, guinea pigs, and mice have all been documented to exhibit symptoms when experimentally infected with Hendra virus. Other animals, such as rats and rabbits have a similar reaction as dogs to viral infection in that they produce antibodies but are asymptomatic when experimentally infected.[6]
Hendra virus infections typically occur in groups with prolonged contact with dead or dying infected horses and their body fluids. This is evident by the fact that 5 of the 7 confirmed cases were veterinarians or their assistants, who performed necropsies or procedures like nasal cavity lavages, which put them in close contact with body fluids, on Hendra virus infected horses.[4,13] The remaining cases involved trainers or stable workers who also interacted closely with the infected horses.[3] This close contact is necessary because the virus does not appear to easily infect humans, evident by data from the 2008 outbreak. In that outbreak, 20 people at a veterinary clinic had exposure to body fluid from Hendra virus infected horses, but only 2 developed symptoms, an attack rate of only 10%.[4] It is interesting to note that one of the most recent Hendra virus outbreaks, in 2008, was characterized by encephalitis, instead of the respiratory symptoms seen in earlier outbreaks.[4] Some variation in the strain that caused this outbreak an previous strains was observed, making it possible that the genetic changes altered the virus's pathogenesis slightly.[11]
Diagnosis
Hendra virus is typically diagnosed using serologic testing. This typically involves collecting serum or other tissue samples from the patient and running reverse PCR on the sample to look for the viral RNA. Other serologic tests include running tests to look for specific antibodies (typically IgM and IgG) in serum. These genomic-based tests are particularly useful because they can also point out genetic differences between the viruses implicated in different outbreaks.[4] The patient's history could also be useful in making a diagnosis, especially since Hendra virus outbreaks typically begin in horses and close contact with infected horses is necessary to become infected.
Treatment
Currently there is no standard treatment for Hendra virus in humans or horses. In the 2008 outbreak, the drug ribavirin was administered to human cases, one of which survived. This drug has been shown to delay death in Nipah virus infected hamsters. However, ribavirin's effects on Hendra virus are still unknown at the time of the outbreak.[4] The drug was used as a treatment and as prophylaxis to people exposed to infected horses because it caused few side effects in patients and no other treatment was known.[4] Later experiments have shown that ribavirin only delayed the start of Hendra virus infection and did not greatly influence the course or outcome of the disease.[14] A more recent, and more promising, treatment is a human monoclonal antibody. The antibody recognizes the region of the virus's attachment glycoprotein that binds to the host cell's receptor.[14] With regards to horses, many cases are only detected in the final stages of the disease, when the horse either dies from the disease or is euthanized. If the horse has recovered or is determined to be asymptomatic, it is euthanized because of the risk of transmission to humans.[3,7]
Prevention
Because of the lack of established treatment for Hendra virus and its high mortality rate in humans, preventing contact between humans and body fluids of infected horses is one of the best way to prevent Hendra virus infection. This can be done by wearing protective equipment like rubber boots, gloves, a face shield and respirator, and an overall or apron to prevent splashed fluids from landing on skin or eyes or being inhaled.[6] Prevention can also include minimizing the number of people with contact to the infected horse, quarantining the infected horse, and refraining from doing invasive procedures, such as necropsies, if adequate protective equipment is not available.[6] Although specific disinfectants have not been tested against Hendra virus to determine the most effective one, many disinfectants have proved effective against viruses with a similar structure as Hendra virus. These can be used to clean the quarantine area, equipment used on the infected horse, and other contaminated surfaces.[6] Prevention can also go beyond the death of an infected horse. This includes disposing of the carcass correctly, whether by deep burial or burning, while wearing appropriate protective equipment, and disinfected the burial site, burial equipment, and burial personnel after the carcass is disposed of.[6]
Another form of prevention is stopping the transmission of Hendra virus from flying foxes to horses. In 2012, a horse vaccine against Hendra virus was released for use in Australia. The vaccine uses a soluble version of the virus's attachment glycoprotein to stimulate an immune response. The vaccine proved very effective at preventing Hendra virus infection in experiments. Vaccinated horses did not develop symptoms after being inoculated with the virus, and the virus was not detected in any of their body fluids.[6] Currently, the vaccine is the best form of prevention because it effectively stops the transmission between flying foxes and horses.
Virulence Factors and Immune Response
Many of the molecular mechanisms of Hendra virus pathogenesis are still unclear, making it difficult to determine virulence factors.[11]
Viral Structure and Virulence Factors
In terms of overall structure, the virus has a lipid envelope enclosing the linear ribonucleoprotein core. The core is made up of single-stranded, non-segmented negative sense RNA bound to nucleocapsids proteins. Matrix proteins that help determine viral shape lie underneath the viral envelope, which also contains phosphoproteins and a large polymerase protein, both of which help transcribe the viral genome.[14] Regarding pathogenesis, the two most important, or at least well known, proteins project from the lipid envelope. One is the attachment glycoprotein (G), and the other is the fusion glycoprotein (F).[6] The virus targets the ligands ephrin-B2 and ephrin-B3 on the host cell surface. It first attaches to the receptor using the attachment glycoprotein. Next, the fusion glycoprotein mediates fusion of the viral lipid envelope with the host cell membrane.[6] Potentially one reason why Hendra virus can be isolated from so many body tissues and organs is the fact that ephrin-B2 is expressed by cells throughout the body, ranging from neurons and endothelial cells to the spleen and lymph nodes. Additionally, ephrin-B3 is mostly expressed in the central nervous system, which would also explain the ability of the virus to colonize those organ, causing encephalitis.[6] The ephrin ligands, likely because they serve an important role in cell signaling, are very similar structurally across animal species, which provides a probable explanation for the wide host range of Hendra virus.[15] Despite the fact that many aspects of Hendra virus pathogenesis are unknown, the important of the two glycoproteins, and their receptors, for pathogenesis is well established. Experiments have shown that cell lines lacking ephrin-B2 do not allow Hendra virus to enter, thereby preventing pathogenesis.[15]
With regards to other viral proteins, the P gene can code for four proteins: P, W, V, and C.[14] The different proteins coded by the P gene are believed to have some effect on the immune system. This is because the P, V, and W genes are encoded by an RNA editing mechanism, which has been shown to suppress interferon production.[14] Specifically, the V protein has been shown to interact with the W protein to prevent the activation of interferon beta promoter and block interferon signaling in infected cell.[14] However, these observations have not been consistent across experiments, and more research needs to be done to specifically narrow down how the viral proteins are affecting the immune response
Role of the Immune Response in Infection
Histologically, Hendra virus infection results in severe damage to the human or equine host. Viral replication and release from endothelial cells and neurons likely contributes some to the damage and symptoms.[6] However, Hendra virus does activate the immune response. When the virus infects epithelial cells in the respiratory tract, inflammatory cytokines like IL-6, IL-8, MCP-1, and G-CSF, are produced.[11] This results in a massive inflammatory response in the lungs, which is damaging to the body's tissues. This inflammatory response can even progress to Acute Respiratory Distress Syndrome (ARDS)-like disease.[11] Thus, the body's own inflammatory response could be causing some of the respiratory symptoms of the infection. Viral dissemination through the bloodstream is also closely associated with the immune response. The virus can travel through the bloodstream either independently or bound to leukocytes like CD3+ leukocytes, which it can bind to without actually entering.[11] Additionally, in pigs the virus has been shown to be capable of infecting monocytes, natural killer cells, and T cells expressing CD6 and CD8. The ability to infected CD6 expressing leukocytes could potentially contribute to viral pathogenesis because this receptor binds to an adhesion molecule, ALCAM, that is expressed by endothelial cells in the small vessels of the blood-air and blood-brain barriers.[11] Therefore, infection of these cells could provide the virus with a direct means of entering the central nervous system.
Finally, viral infection of the central nervous system corresponds to the expression of additional inflammatory cytokines like TNF-alpha and IL-1beta. These cytokines can break down the blood brain barrier, another characteristic of Hendra virus infection, by increasing vascular diameter and permeability, and also damage the neurons through the inflammatory response.[11] However, like many aspects of Hendra virus pathogenesis, it is still unclear how much of the damage comes from the viral infiltration and replication and how much comes from the immune system's response. The inflammatory cytokines can be expressed in the brain by microglia. However, it is also possible that the breakdown of the blood brain barrier is caused directly by viral replication in the vessels that compose the barrier.[11] Clearly more research needs to be done to clarify the molecular mechanisms of Hendra virus pathogenesis. Despite this uncertainty, it is clear that an infection is characterized by a strong inflammatory response, the breakdown of endothelial cells around the body, and systematic infection by the Hendra virus.
Damage Response Framework
A common trend of pathogenic microbiology is to classify pathogens into groups. This can be based on a variety of factors, such as the organ system infected, the route of infection, the type of pathogen, and so on. A more recent classification system groups pathogens on the amount of damage done to the host. Called the damage response framework, this classification system takes a more comprehensive look at disease by acknowledging both sides of the host-pathogen interaction. That is, the damage seen as symptoms during an infection can be caused by the microorganism or by the host's response to the microorganism.[16] Thus, damage to a host can range from none up to death. It is also possible that the microorganism provides some benefit to the host. From this point of view, every step of an infection can be plotted on a graph of damage versus time. A horizontal line in the middle of the graph represents a neutral interaction. The host is neither helped nor harmed by the microorganism. Going up the graph represents an increase in damage, which can culminate in death. Going down the graph represents a benefit to the host by interaction with the microorganism.
Hendra virus has a relatively complex damage response framework. At the initial moment of infection, the graph starts at the horizontal line. During the incubation period, the graph does not move because the virus is establishing itself in the body. After the incubation period, which can range from 7-6 days up to 9-16 days, symptoms begin to appear. Generally, they are very mild and flu-like initially, lasting for about 3 to 4 days.[4,7,8] At this point, the damage response framework increases slightly, because symptoms indicate that some damage is occurring to the host. From here, the damage response framework can take multiple pathways. It is possible that the symptoms remain mild and flu-like. In the human cases where this has been observed, the symptoms last around 8 days.[7,8] For these cases, the damage response framework levels out and then returns to the horizontal line after 8 days, when the infection has been cleared. In another human case, the flu-like symptoms grew more severe, requiring ventilation. Kidney function also deteriorated, and the patient died about 7 days after symptoms grew more severe.[9] In this case, the virus spread systemically through the patient's body, causing multi-organ failure. In this damage response framework, the graph increases sharply, culminating in death.
Three of the Hendra cases involved encephalitis. For one case, the initial flu-like symptoms went away after about 4 days. However, mild neurological symptoms appeared the next day and gradually became worse over the next 4 days. The patient remained unconscious and on ventilation for 4 weeks after that before dying.[4] In this situation, the damage response framework would go down after the flu-like symptoms, and then immediately go back up with the onset of neurological symptoms. The graph would gradually increase over the 4 day period that symptoms worsened, and would then level out for 4 weeks. Death could be indicated by a sharp increase in the end of the graph. Another Hendra case had a very similar disease progression, in that after the flu-like symptoms abated neurologic symptoms developed and worsened. However, this patient eventually recovered after 37 days of treatment.[4] So for this damage response framework graph, after the graph plateaus at the neurologic symptoms it would gradually go down until reaching the horizontal line.
The last case of Hendra virus would have the most unusual damage response framework. After the incubation period, where the damage response framework graph would not move from the horizontal line, the patient experienced 12 days of flu-like symptoms as well as drowsiness and a stiff neck.[8] In the damage response framework, the graph would go up slightly, likely higher than the cases involving just flu-like symptoms, and plateau for 12 days. At this point, the patient recovered, so the damage response framework graph would return to the horizontal line, because no symptoms indicates a lack of damage to the host. Almost one year later, the patient again became sick with back pain, seizures and mood swings. These gradually grew worse until he died 25 days later.[8] In the damage response framework graph, the line would remain at the neutral position for about 12 months before steadily beginning to climb. This would indicate the worsening neurological symptoms and eventual death.
Clearly, Hendra virus is a complicated illness that can have many different clinical presentations. However, the damage response framework provides a way to visually represent the disease progression and understand the pathogenesis with regards to host damage.
References
1. Wang LF, Yu M, Hansson E, Pritchard LI, Shiell B, Michalski WP, Eaton BT. 2000. The Exceptionally Large Genome of Hendra Virus: Support for Creation of a New Genus within the Family Paramyxoviridae. J Virol 74:9972–9979.
2. Eaton BT, Eaton BT, Broder CC, Broder CC, Middleton D, Middleton D, Wang LF, Wang LF. 2006. Hendra and Nipah viruses: different and dangerous. Nat Rev Microbiol 4:23–35.
3. Murray K, Rogers R, Selvey L, Selleck P, Hyatt a., Gould a., Gleeson L, Hooper P, Westbury H. 1995. A Novel Morbillivirus Pneumonia of Horses and its Transmission to Humans. Emerg Infect Dis 1:31–33.
4. Playford EG, McCall B, Smith G, Slinko V, Allen G, Smith I, Moore F, Taylor C, Kung YH, Field H. 2010. Human Hendra Virus Encephalitis Associated with Equine Outbreak, Australia, 2008. Emerg Infect Dis 16:219–223.
5. Field H, Young P, Yob JM, Mills J, Hall L, Mackenzie J. 2001. The natural history of Hendra and Nipah viruses. Microbes Infect 3:307–314.
6. 2011. Guidelines for veterinarians handling potential Hendra virus infection in horses. The State of Queensland (Department of Agriculture and Fisheries).http://www.daff.qld.gov.au/documents/Biosecurity_GeneralAnimalHealthPestsAndDiseases/Hendra-GuidelinesForVets.pdf.
7. Hanna JN, McBride WJ, Brookes DL, Shield J, Taylor CT, Smith IL, Craig SB, Smith G. 2006. Hendra virus infection in a veterinarian. Med J Aust 185:562–564.
8. Paterson DL, Murray PK, McCormack JG. 1998. Zoonotic Disease in Australia Caused by a Novel Member of the Paramyxoviridae. Clin Infect Dis 27:112–118.
9. Haplin K, Young PL, Field HE, Mackenzie JS. Isolation of Hendra virus from pteropid bats: a natural reservoir of Hendra virus. J Gen Virol. 2000 Aug;81(Pt 8):1927-32.
10. Marsh GA, Haining J, Hancock TJ, Robinson R, Foord AJ, Barr J a., Riddell S, Heine HG, White JR, Crameri G, Field HE, Wang LF, Middleton D. 2011. Experimental Infection of Horses with Hendra virus/Australia/horse/2008/Redlands. Emerg Infect Dis 17:2232–2238.
11. Escaffre O, Borisevich V, Rockx B. 2013. Pathogenesis of Hendra and Nipah virus infection in humans. J Infect Dev Ctries 7:308–311.
12. Rockx B, Brining D, Kramer J, Callison J, Ebihara H, Mansfield K, Feldmann H. 2011. Clinical outcome of henipavirus infection in hamsters is determined by the route and dose of infection. J Virol 85:7658–7671
13. Mendez DH, Judd J, Speare R. 2012. Unexpected result of Hendra virus outbreaks for veterinarians, Queensland, Australia. Emerg Infect Dis 18:83–85.
14. Marsh G a., Wang LF. 2012. Hendra and Nipah viruses: Why are they so deadly? Curr Opin Virol 2:242–247.
15. Bonaparte MI, Dimitrov AS, Bossart KN, Crameri G, Mungall B a, Bishop K a, Choudhry V, Dimitrov DS, Wang L-F, Eaton BT, Broder CC. 2005. Ephrin-B2 ligand is a functional receptor for Hendra virus and Nipah virus. Proc Natl Acad Sci U S A 102:10652–10657.
16. Casadevall A, Pirofski L. 2003. The Damage Response Framework of Microbial Pathogenesis. Nat Rev 1:17-24.
Created by Sabrina Waugh, student of Tyrrell Conway at the University of Oklahoma, 2015