Legume-Rhizobium
Introduction
Rhizobia are symbiotic diazotrophs (prokaryotic organisms that carry out dinitrogen fixation) that form a symbiotic association with legumes. This association is symbiotic in that both the plant and rhizobia benefit. The plant supplies the rhizobia with energy in the form of amino acids and the rhizobia fix nitrogen from the atmosphere for plant uptake. The reduction of atmospheric dinitrogen into ammonia is the second most important biological process on earth after photosynthesis (Sylvia, 2005). The actual process of dinitrogen fixation can only be carried out by diazotrophs that contain the enzyme dinitrogenase. Nitrogen is the most critical nutrient needed to support plant growth. Unfortunately, atmospheric dinitrogen (78% of air we breathe) is extremely stable due to triple bonds which can only be broken by energy intensive ways. These include electrical N2 fixation by lightning where oxides of N come to ground with rain, the Haber-Bosch process in industrial fertilizer production, and biological N2 fixation in legumes by bacterial symbionts such as Rhizobium etli. Biological fixation of nitrogen was the leading form of annual nitrogen input until the last decade of the 20th century (Russelle, 2008). It is gaining attention once again as sustainability becomes a central focus to feed a world population of over 7 billion people.

Biological interaction
Biological N2 fixation takes energy which comes at the expense of photosynthate (sucrose). Generally, legumes gain extra nitrogen for plant growth to offset the loss of photosynthate in this mutualistic association. The rhizobia invade plant roots and induce a nodule in which the bacteria reduce atmospheric nitrogen to ammonia and supply the plant with nitrogenous compounds (Young, 1989). The plant gains the ability to grow in nitrogen poor soils, and the bacteria gain a protected niche where they multiply and eventually escape back into the surrounding soil when the nodule senesces (Young, 1989).
Because biological N2 fixation requires such a large amount of energy, it is important to understand the energy transfer in the process. The stepwise reaction of energy transfer is characterized by the following steps: N2 yields diamine which yields hydrazine which yields NH4+. Each yield requires 2 e- for a total of 6 electrons needed. Electrons come in via Fe protein and are donated by ferredoxin. ATP is used to reduce N2 to NH4+ whereby ATP hydrolysis takes place and the Fe protein reduces the MoFe protein which reduces the nitrogen (Dixon and Wheeler, 1986). 4 ATPs are used per electron in the N2 fixation process. N2 + 6e- + 8H+ yields 2 molecules NH4+. So a total of 24 ATPs would be required to make 2 molecules of NH4+ from one molecule of N2.
Nodulation
The actual process of nodulation is a very coordinated effort between the legume and the Rhizobium bacteria in the soil. Infection typically occurs in root hairs of legumes. Many rhizobia and host plants are highly specific and legumes can either attract rhizobia to root hairs directly by excretory compounds or by induction of nod gene activity in the bacteria.
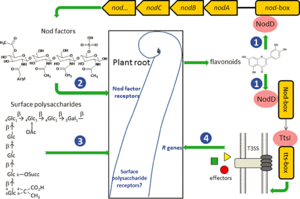
Communication between legume and Rhizobium
1. Flavonoids are released by the host root. The flavonoid is at the highest concentration at the root and interacts with the product of bacterial nodD gene. The nodD gene produces the protein, nodD, which is the sensor that recognizes chemicals excreted by host plant roots (Russelle, 2008).
2. Rhizobia colonize the soil in the vicinity of the root hair in response to the flavonoids. This process is autoregulated where favonoids stimulate Nod factor production, which stimulates flavonoid secretion (Russelle, 2008).
3. Response to Nod factors is extremely rapid and the disruption of cell wall happens very quickly. Disruption of crystallization of cell walls take place, thereby allowing entrance by the rhizobia. At the same time Rhizobia multiply in the rhizosphere. The root hair is then stimulated and curls to the side where the bacteria are attached which stimulates cell division in the root cortex.
4. A "shepherd's crook" is formed and entraps the rhizobia which then erode the host cell wall and enter near the root hair tip. An infection thread is formed as rhizobia digest the root hair cell wall. Free-living Rhizobium bacteria are converted to bacteroids as the infection elongates by tip growth down root hair and toward epidermal cells.
5. Infection thread branches and heads toward the cortex and a visibly evident nodule develops on the root as the plant produces cytokinin and cells divide. Nodules can contain one or more rhizobial strains and can be either determinant (lack a persistent meristem and are spherical) or indeterminate (located at the distal end of cylindrically shaped lobes) (Russelle, 2008). Many infections are aborted due to a breakdown in communication between rhizobia and the host plant leaving nodule number strictly regulated by the plant.
6. Once inside the nodule, rhizobia are released from the infection thread in a droplet of polysaccharide. A plant-derived peribacteroid membrane, which regulates the flow of compounds between the plant and bacteroid, quickly develops around this droplet via endocytosis. This process keeps the microbes "outside" the plant where the rhizobia are intracellular but extracytoplasmic (Russelle, 2008). The loss of the ammonium assimilatory capacity by bacteroids is important for maintaining the symbiotic relationship with legumes.
Niche
The amount of N2 fixed depends on the soil population of bacterial symbionts, soil acidity, and often overlooked soil nitrogen availability. Nodulation will only be initiated when the plant is in low nitrogen status. Rhizobium populations are sensitive to changes in environmental conditions.
Favorable Environment
A balanced pH with high levels of nutrients and good physical properties is favored by rhizobia. A variety of C and N compounds can be utilized by rhizobia. A single rhizobial cell in a favorable environment can infect a root hair and generate 1010 progeny (Russelle, 2008).
Unfavorable Environment
Rhizobia can be reduced in numbers by strong soil acidity which has high hydrogen ion concentration. Plant growth can also be limited by toxic levels of aluminum and manganese. A reduction in rhizobial pools can be due to nutrient limitations including deficiencies in calcium, phosphorus, and molybdenum, low or high soil temperatures (rhizobia are mesophiles), and poor soil physical properties that restrict aeration and moisture supply. (Sylvia, 2005). Soil acidity reduces nodulation and overall N2 fixation. Soil nitrate concentration and phosphorus concentration can also affect rhizobia populations. Furthermore, indegenous rhizobial populations are maintained over time and depend on how often host plants are grown and on competitive ability of different rhiboia strains (Furseth et al., 2012). Hundreds of microbial species can fix nitrogen as most nitrogen fixing prokaryotes are free-living organisms or associate with plants. Unfortunately, the environmental factors have to optimal for many of the aerobic, microaerobic, anaerobic, and even photosynthetic bacteria, and actinomycetes to be reliable sources of nitrogen fixation.
Microbial processes
Estimates of the amount of N2 fixed range from 57 – 600 kg/ha per year and vary widely. (Evans and Barber, 1977). The rates of N2 fixation, unfortunatley, cannot be measured accurately (LaRue and Patterson, 1982). Legumes prefer to take up available soil nitrogen from soil solution as fixation by bacteria is expensive to the plant. N2 fixation is constrained in many agricultural soils where nitrogen levels are high from routine addition of fertilizer. Nitrate in the soil reduces fixation where nitrate reduction uses photosynthate.
Role of Nitrogenase
Nitrogenase is the actual enzyme responsible for conversion of N2 to ammonium. Nitrogenase exists in three forms that include molybdenum nitrogenase, vanadium nitrogenase, and iron nitrogenase. Mo-containing nitrogenase is the most widely studied and is the enzyme utilized by Rhizobium (Russelle, 2008). This enzyme actually is made up of two enzymes, dinitrogenase and dinitrogenase reductase. Nitrogenase is regulated by N supply and by O2 which can inhibit nitrogenase. Leghemoglobin, responsible for giving efficitive nodules their pink color, binds oxygen and transfers it to bacterial electron transport chain so that ATP synthesis can occur. Thus, the concentration of free O2 is lower in the nodule. Unfortunately, high amounts of ATP and oxygen reductant are needed to meet the demands of the enzyme, but at the same time, nitrogenase is oxygen sensitive. This is often refered to as the 'paradox' of symbiotic nitrogen fixation (Schulze, 2004).
Key Microorganisms
There are currently six phylogenetically distinct genera of rhizobia. The taxonomy of these organisms is still in flux due to rapidly advancing analytical techniques.
Allorhizobium
Allorhizobium is a monospecific genus.
Allorhizobium species
A. undicola produces nodules on Neptunia prostrata. This plant is an aquatic legume indigenous to humid tropics used for both human consumption and green manure (Russelle, 2008).
Azorhizobium
Azorhizobium produce nodules on the aquatic legume Sesbania rostrata. Azorhizobium are unique in that they have the ability to grow with N2 as the only nitrogen source. (Russelle, 2008).
Azorhizobium species
A. caulinodans is the only species for this genus.
Bradyrhizobium
Bradyrhizobium as a genus grows slowly and is widely known for symbiosis with soybean, but other crops such as peanut, lupine, and cowpea can form symbiosis with Bradyrhizobium.
Bradyrhizobium species
Bradyrhizobium japonicum and B. elkanii are found in symbiosis with soybean. B. lupini is a symbiont of lupine. B. spp. nodulate peanut.
Mesorhizobium
Mesorhizobium grow faster than Bradyrhizobium, but slower than Rhizobium and Sinorhizobium. They nodulate trefoils, chickpea, and found in the nodules of several legume species in China.
Mesorhizobium species
Mesorhizobium loti nodulates trefoils, M. huakuii nodulates Astragalus, M. ciceri and M. mediteraneum nodulate chickpea, and M. tianshanense are found in symbiosis with several legume species in China (Russelle, 2008).
Rhizobium
Rhizobium form symbiosis with vetches, peas, lentil, clovers, and beans.
Rhizobium species
R. leguminosarum nodulates vetch, R. tropici is a symbiont of beans and other hosts, Rhizobium etli nodulates both alfalfa and beans, R. gallicum can nodulate bean, R. giardinii nodulates leuceana, R. galegae nodulates galega, and R. spp NGR234 nodulates 112 genera of legumes and the nonlegume Parasponia andersonii.
Sinorhizobium
Sinorhizobium produce nodules in alfalfa, medics, sweetclover, sesbania.
Sinorhizobium species
Sinorhizobium meliloti nodulates alfalfa, medics, and sweetclover. S. fredii nodulates soybean; S. saheli and S. terangae nodulate roots of Sesbania, Acacia, Leucaena leucocephala, and Neptunia prostrata.
Current Research
Seed-applied Rhizobia Inoculants
Biological fixation of nitrogen can contribute large amounts of plant usable nitrogen to the soil nitrogen pool. Only a small percentage of soybean producers use rhizobia inoculants as a seed treatment in an attempt to maximize the biological fixation of atmospheric nitrogen to plant usable forms in the soil. Results of this experiment indicate that the greatest responses of rhizobia inoculation on yield came on soils having low levels of indigenous rhizobial populations. Yield responded positively to inoculation at only 3 out of 18 environments. The investigators linked low response to high levels of rhizobial populations which were maintained by regular crop rotation with soybean, which kept rhizobial levels sufficient for plant nodulation and rhizobium bacteria reproduction. Researchers concluded that soybean growers should only inoculate soybean seed with rhizobia if soybean had not been grown for 5 or more years at a particular tract of land (Furseth et al., 2012).

Pesticides Reduce Rhizobia Efficiency
Symbiotic nitrogen fixation (SNF) is noted to reduce the need for excessive synthetic fertilizer additions by replacing man-made nitrogen with a naturally produced form. Utilizing crop rotation with legumes could save millions or billions of dollars currently being spent on synthetic nitrogen forms used extensively in monoculture agriculture, namely continuous corn production. Creating environments that will allow chemical signals to be exchanged between the host plant and rhizobia are extremely important in efficient SNF. Phytochemical signals sent out by host plant roots are only specifically recognized by certain soil bacterium leading to high host specificity by rhizobium. Disrupting this signal is costly and was examined by the investigators. The researchers tested five phytochemicals ranging from natural derived phytochemicals to various insecticides (some of which are no longer used in production agriculture, namely DDT) and found that all chemical treatment groups exhibited significantly lower plant yields, inhibited establishment of symbiosis, reduced SNF, and negatively impacted N fixations and plant biomass production (Fox et al., 2007).
Improving Symbiotic N Fixation
Much of the energy consumed in nitrogen fixation is wasted in the production of H2. Luckily there are strains, namely the hydrogenase positive Hup+ strains, that can oxidize the H2 produced during the nitrogenase reaction and recover energy. Using these strains of Bradyrhizobium japonicum as an inoculant could increase soybean yield and increase nitrogen fixation efficiency. Improvement of these strains could lead to a higher amounts of nitrogen fixation (Maier, 1996). Microbial biologists have taken the first step of identification of genes that can enhance nitrogen fixation and discovery of competition strategies against indegenous bacteria (Kent, 1998). Furthermore, utilization of anti-rhizobial peptides called trifolitoxins to reduce the inability of inoculum strains to compete with native root nodule bacteria for root nodulation would be beneficial. The addition of the plasmid pHUTPFXPAR to a rhizobium population increased nodulation competitiveness and increased efficiency by way of Hup+ had significant yield increase compared to unioculated treatment. Further research is needed to ensure that this type of antibiotic-resistant gene will not affect indeginous rhizobial communities via lateral gene transfer.
References
Evans, H.J., and Barber, L.E., 1977. "Biological nitrogen fixation for food and fiber production. What are some immediately feasible possibilities"? Science. 197:332-339.
Furseth, B.J., Conley, S.P., and Ane, J-M. 2012. "Soybean Response to Soil Rhizobia and Seed-applied Rhizobia Inoculants in Wisconsin". Crop Science. 52:339-344.
LaRue, T.A., and Patterson, T.G., 1982. "How much nitrogen do legumes fix"? Adv. Agron. 34:15-38.
Russelle, M. P. "Biological Dinitrogen Fixation in Agriculture". Nitrogen in Agricultural Systems. Ed. Schepers, J.S. and Raun W.R. 2008. 281 - 359.
Schulze, J. 2004. "How are nitrogen fixation rates regulated in legumes?" J. Plant Nutr. Soil Sci. 167:125-137.
Sylvia, D.M., Fuhrmann, J.J., Hartel, P.G., and Zuberer, D.A., 2005. Principles and Applications of Soil Microbiology. 2nd Edition. 373 - 404.
Wang, D., Yang, S., Tang, F., Zhu, H., 2012. "Symbiosis specificity in the legume-rhizobial mutualism". Cellular Microbiology: 14(3):334-342.
Edited by Blake Meentemeyer, a student of Angela Kent at the University of Illinois at Urbana-Champaign.