Murine Gammaherpesvirus 68
Introduction

Murine Gammaherpesvirus 68 (MHV-68) is a rodent pathogen and a member of the gammaherpesvirus subfamily. Other viruses in this subfamily include the Epstein-Barr virus (EBV) and Kaposi’s sarcoma-associated herpesvirus (HHV-8) that both infect humans. MHV-68 is genetically similar enough to both EBV and HHV-8 that it can be used as an animal model for study of its pathogenesis. (1) Gammaherpesvirus structure includes a capsid containing viral DNA surrounded by a viral envelope with a surface of glycoprotein spikes. Between the inner capsid and outer envelope layers is the tegument layer, containing virion proteins (Fig 1). (1)
MHV-68 has the ability to establish latent infections within lymphocytes and make close associations with cell tumors. MHV-68 establishes latency unless the host immune system is compromised, and this latency can be regulated by multiple cellular controls, such as virus-specific open reading frames that result in gene products promoting the maintenance of latency or activation of lytic cycles. One of the major consequences of MHV-68 in mice is infectious mononucleosis. (1)
Other related herpesviruses, including MHV-60, MHV-72, MHV-76, and MHV-78 have also been isolated in bank voles, wood mice, and shrews. Surveys estimate that up to 70% of bank voles and wood mice in the UK carry the virus in the respiratory tract. There is geographical dispersion of the virus, but it was first isolated in Slovakia. These several related viruses caused cytopathic effect, or host cell damage, in epithelium from species other than their natural hosts, including chickens and primates. (2) Genomic sequencing has characterized MHV-68 as being more similar to herpes saimiri (HVS) with a natural host of squirrel monkeys, than to EBV with human hosts. MHV-68 itself was isolated from bank voles (Clethrionomys gladiolus).
Genomic Structure of MHV-68
The complete genome of MHV-68 has been sequenced and it has been calculated that at least 75% of its gene products are homologous to those of HHV-8 and HVS. (1) While multiple viruses within the gammaherpesvirus subfamily have genes arranged co-linearly, there are open reading frames (ORFs) that are virus-specific. (1) ORFs are key in analyzing the gene products of latent and lytic virus cycles, and determining what stimulates the switch from one cycle to the other. Within the gammaherpesvirus subfamily, there is a division between gamma-2 and gamma-1 herpesviruses that is determined by genomic sequencing. The main basis for the genomic distinction within the subfamily is the mechanistic differences in activation from latency to lytic cycles as evidenced by the coding of gene products (Fig. 2). (1)
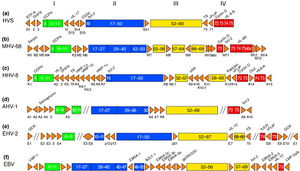
Open Reading Frames
Open reading frames of significance in MHV-68 include M1, M2, M3, M7, M11, and ORF37 and ORF73.
M1 is homologous to the poxvirus serpin protein family, but is not necessary for beginning the latency virus cycle. Its purpose is still unclear, but its gene product may regulate apoptosis or host inflammatory response. (1)
M2 one of the few genes of MHV-68 transcribed during the virus’ lytic cycle. Most genes are transcribed during latency. The M2 locus has been identified as the target for zinc finger antiviral protein (ZAP) binding. ZAP binds to mature M2 mRNA and reduces its expression. Reduced expression of M2 may prevent activation of the lytic cycle, since M2 is overexpressed to induce reactivation. (4)
M3 codes for a protein that binds to chemokines. (8) Chemokines recruit hemotopoietic cells to infection sites, meaning they modulate immune response in a way that opposes viral infections. The M3 chemokine-binding protein is secreted abundantly to block chemokine function and has been identified as the first binding protein to be involved in chemokine interaction in herpesviruses. (8)
M7 is a virus-specific ORF that codes for the membrane glycoprotein gp150, which plays a key role in the viral membrane’s attachment and fusion to host cell membrane. (1) M7 is considered a marker of late lytic replication.
M11 is a B-cell lymphoma 2 (bcl-2 gene) homolog that has been shown to inhibit apoptosis in EBV and HHV-8, and it is derived from host genome. Bcl-2 genes either induce or inhibit apoptosis, and damage in Bcl-2 genes has been linked to cancer. By inhibiting apoptosis in the cells it infects, M11 can prolong cell life to allow for an entire lytic replication cycle to occur. (1)
ORF37 encodes viral DNA exonuclease, an enzyme involved with the processing and encapsidation of viral DNA as well as modulation of host shutoff. Host shutoff is a mechanism highly conserved in gammaherpesviruses and is utilized to provide an opportunity for the virus to initiate the lytic replication cycle and infect its host. The virus inhibits all cellular transcription, mRNA processing, and protein translation to ensure only its gene products are produced and dominant. (7)
ORF73 is reported to be responsible for a gene product critically important for the initiation and continuation of latency, and is unnecessary for lytic replication cycle efficiency. This ability is important in MHV-68 because it remains latent for an extended period of time in its hosts and remains latent in the host post-lytic infection. (6)
Other Genetic Characteristics
MHV-68 is genomically unique in that at its far 5’ end there are eight tRNA-like sequences, the function of which has yet to be elucidated. The viral tRNAs could potentially be markers for latent infection. (1) Also genomically significant is the ability of gammaherpesviruses to integrate host DNA into the viral genome, as this horizontal transfer allows for continual evolution. In MHV-68 and other gamma-2 herpesviruses there are distinguishable cellular homologous gene products that have been derived from host genome. Gene products from host homologues are predicted to play a role in cell cycle and apoptosis regulation. (1) Genomic manipulation of MHV-68 DNA is useful as a model for study of ORF function by construction of deletion mutants. Gene deletion mutants are also important for investigation of MHV-68 infection mechanisms. (1)
Viral Replication Cycle
Herpesvirus infections and replication occurs in a series of steps (Fig 3), and MHV-68 follows this pathway beginning with attachment of the virion membrane to the host cell. This is accomplished due to the viral plasma membrane's derivation from the host cell intracellular membrane, which prevents its identification as a harmful virion and instead allows for fusion with the host cell membrane. Once the herpesvirus outer glycoproteins attach to the host membrane proteoglycans, the nucelocapsid containing double-stranded viral DNA is pushed into the host cell cytoplasm. The nucelocapsid moves through the cytoplasm and binds to the nuclear membrane. It injects its genomic contents into the nucleus through a nuclear pore. Once within the nucleus, the viral DNA circularizes and undergoes transcription. Within the tegument proteins there is a virion host shutoff factor protein (vhs) that serves to degrade host cell mRNA in the cell nucleus, thus favoring the viral gene transcripts. From this point, the virus can enter a latent or lytic replication cycle.
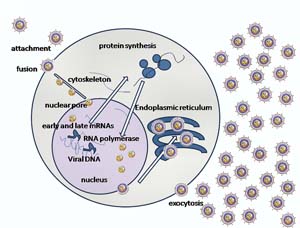
The differentiation between transcription of genes during latency and lytic cycles is key in understanding the dynamics of how MHV-68 interacts with host immune response. Because latency is established, mRNA is transcribed from genes that encode products to maintain latency, and these latency gene products exist in the host cell cytoplasm. The series of four vtRNAs at the 5’ end of the viral DNA are transcribed during latency at approximately one week post infection. (5) First the vtRNA is detected in scattered cells, but becomes more widespread as time goes on during latency, eventually becoming detected only within lymphoid follicles (5). The investigation of putative latency-associated ORFs revealed M3 as being abundantly transcribed during latency, but this number did not fluctuate, as did the number of cells positive for vtRNA transcription. (5) The localization of M3 RNA did not change from the scattered cells in the periartiolar lymphoid sheath (PALS), whereas vtRNA transcripts expanded to cells within GCs. (5)
Latently infected B-cell lymphoma derivative cell lines exhibit transcription of M2, M11, ORF73, and ORF74. (3)
Latent Cycle
Latency replication cycles are common in many viruses, and are a kind of dormancy within the host. The virus maintains infection within the host cells without causing harm, cell lysis, or proliferating its own virions. The viral genome remains in the host cells, yet transcription occurs for select cells, since viral demands differ during lysogenic and lytic cycles. The advantage of latency for viruses is the ability to remain undetected by the host immune system until reactivated into the lytic cycle.
MHV-68 establishes latency in lymphoid tissue, resulting in the enlargement of lymph nodes. (1) MHV-68 latency has an interesting rise and fall pattern in the number of infected cells, as infected splenocytes peak and then decrease as they are detectable within splenic germinal centres. Central to understanding the maintenance of latent infection is the identification of lymphoid tissue cell type that the virus persists within. While vtRNAs serve as a marker of latent infections, it remains to be determined what cell types are targeted. (1)
Lytic Cycle
For the herpesvirus to reactivate and lytic cycle, the well-conserved ORF50 must be transcribed to produce its protein product, replication and transcription activator (RTA). (3) M3 is also transcribed abundantly during the lytic cycle.
Many of the most abundant transcripts produce capsid proteins or glycoproteins, since herpesviruses express structural genes to a high degree. The least abundant transcripts include proteins involved in DNA replication, correlating to less enzyme expression. (3) Viruses in productive replication prioritize their transcripts, in regards to type of gene product as well as rate of peak expression. The timing of expression of viral genes is consistent with function in the viral replication cycle. ORFs associated with DNA replication were expressed at peak levels in conjunction with each other, suggesting arrangement for upcoming DNA replication. The majority of genes encoding cell structure components such as capsid proteins, tegument proteins, and glycoproteins, all peaked at relatively later times post-infection compared to DNA processing genes. The gene expression cascade follows the pattern of DNA replication proteins peaking first, then cell structure proteins, and finally packaging proteins. (3)
This is in alignment with traditional herpesvirus lytic replication, in which immediate and early mRNAs are transcribed first and exit the nucleus before transcription moves onto late-stage mRNA that encodes envelope proteins. Late proteins are packaged into capsids with concatemeric DNA that has been cleaved into linear chromosomes. Upon exiting the nucleus, the packaged viral capsid adopts part of the nuclear membrane as its outer envelope, and travels through the ER and Golgi to finally exit via exocytosis. Virus reactivation into the lytic cycle is associated with cell proliferation, since cells expressing M3 transcripts are densely compacted within the GC. (5) During reactivation it is claimed that vtRNA transcription ceases. (5)
Infection Mechanisms and Immune Response
Infection Sites and Cellular Targets
MHV-68 Infection sites consist of primarily lung epithelial cells, adrenal glands, and heart tissue, with latent infection in B lymphocytes. (2) Acute lung infection, once resolved, results in lifelong latent infection of lymphoid tissue (Fig 4). Viral targets are primarily B cells for latency rather than T cells. B cells and T cells are both types of lymphocytes, associated with immune response. B cells secrete specific antibodies and are triggered by the presence of a foreign antigen. T cells are responsible for directing immune response and attacking infected cells by recognizing antigens on cell surfaces.

The lung epithelium of the respiratory tract is the initial target of MHV-68, and spread occurs from the lung to the lymphoid system. (2) During lung infection, bronchiolitis develops and inflammatory response to the virus proceeds gradually. The gradual onset of inflammation can potentially be attributed to M3 gene product chemokine-binding protein activity. The immune system uses inflammation as a biological response to pathogens, consisting of series of macrophages and CD8+ T cells. (2) MHV-68 infects local lymph nodes from the lungs, including dendritic cells, macrophages, and B cells. (2) Evidence supports the role of dendritic cells being responsible for the transportation of virus to draining lymph node. Without B cells, the infection is transient as compared to persistent, and spread to other lymphoid regions does not occur. (2) Thus it is speculated that B cells are the primary cell population accountable for virus propagation within a host. B cells from the local lymph node or mediastinal lymph node increase in number of latently infected cells, which then travel through the lymph system to further infect the spleen. Latently infect B cell production is regulated by CD4+ T cells, operating under the claim that MHV-68 utilizes T- and B cell interaction for its benefit to maximize proliferation via B cell latency. (2)
There is evidence that viral tRNA transcription occurs in splenic germinal centres (GCs) during latent infection following acute lung infection, but when inoculated in mice lacking B cells latency is no longer observed. (1) There is controversy over whether exclusively B cells are targeted, as evidence challenging this claim exists, including the observation that post-acute lung tissue infection there has been persistent viral DNA in an “unidentified cell type”. (1) Additionally, when mice are inoculated intraperitoneally rather than intransally, there has been latency in mice lacking mature B cells. Justification for this observation is unclear but centers around the claim that intraperitoneal infection of large amounts of the virus must infect another cell type other than just B cells for establishment of latency. (1)
Consequences of Infection and Immune System Response
MHV-68 is important as a mouse model of gammaherpesviruses due to the relevance of human gammaherpesvirus infections, as indicated by the high amount of unaware human EBV carriers. (1) Carriers of the virus remain unaware unless their immune system becomes compromised, under which conditions the pathogenic virus will begin lytic cycle and likely result in an LPD. Investigation of immune response to varying viral life cycles is critical. MHV-68 infection is similar to EBV infection in that both viruses are controlled by T-cell subset CD8+, and depletion of these T-cells encourages viral proliferation to a fatal degree. (1)
Infection by gammaherpesviruses is associated with a number of diseases, specifically with disorders of proliferative lymph due to increased immune response. It has been noted that long-term MHV-68 infection in mice results in lymphoproliferative disease (LPD), but further studies have indicated limited potential in utilizing MHV-68 as a model for gammaherpesvirus disorders, as MHV-68 does not induce lymphoma efficiently in mice. (1) MHV-68 infection, like EBV, has the ability to prompt infectious mononucleosis (IM) that results in a transient lymphocytosis. The gene products responsible for triggering IM are yet to be determined, as are those products that are immunomodulatory during viral infection. (1)
While antibody response to MHV-68 is very gradual upon initial infection, two weeks post-inoculation shows increased antibody levels that are then continually maintained at high levels. Immunoglobin production is important in mitigating viral development and serum-neutralizing immunoglobin specifically may be useful in lessening the spread of MHV-68. (1)

MHV-68 causes host shutoff using vhs (viral host shutoff protein) and a DNA exonuclease produced from ORF37 (7). Mutants for ORF37 still resulted in infectious virus and mRNA loss characteristic of host shutoff in cells lacking type 1 IFN receptors. Type 1 interferons are proteins involved in immune response against viral infections. The role of ORF37 gene products and the implications for other host shutoff proteins in MHV-68 infection are investigated. Host shutoff in other gammaherpesviruses may function in ways similar to MHV-68. (7)
Interferons are also a significant component of immune response to viruses. Interferon (IFNs) proteins are a kind of cytokine, which is a broad group of cell-signaling proteins. (1) Cell signaling is an important communication mechanism for the immune response. Defense against MHV-68 involves INF-gamma, which plays a role in activating macrophages and interfering with viral replication. Mice lacking functional IFN-gamma are able to eliminate acute viral infection, suggesting that INF-gamma is not necessarily essential for viral clearance. (2) IFN-gamma has, however, been indicated as a critical component in the control of persistent MHV-68 infection. Persistent viral infections differ from acute viral infections in that they are longer lasting and occur when the host cannot clear the primary infection.
IFN-gamma is a type II interferon, and type I interferons such as IFN-alpha and IFN-beta also play a role in viral control. Both alpha and beta interferons are necessary for control of acute viral replication, in contrast to IFN-gamma. Mice lacking IFN-alpha or IFN-beta receptors die within days of inoculation of MHV-68. Thus these cytokines are key at the initial stages of viral infection. (2)
Research and Conclusion
MHV-68 is important for providing insight into viral mechanisms and is widely used in research. In 2003, researchers from the University of Cambridge investigated the function of ORF73 in plasmid maintenance and establishment of latency. Viral ORF73 mutants were generated, "D73" and "D73R", and observed to be "disabled for the establishment of latency in the spleen" (6). Figure 6 shows spleen of infected mice at 7 or 14 days post-infection, probed for viral tRNA using in situ hybridization. The presence of viral tRNA, noted by dark staining, indicates cells with MHV-68 wild type, D73 mutant, or D73-R mutant. No positive cells were in the spleens of D-73 MHV-68 infected mice. It was concluded most likely that "the phenotypes observed are due to disruption of ORF73." (6) Splenomegaly, or enlargement of the spleen, is caused by infectious mononucleosis, which results from MHV-68 infection. When this enlargement occurs, B cell and T cell populations increase, as B cells and CD4+ T cells are necessary for splenomegaly development. Splenomegaly increases the number of GCs, which are an important location for latently infected cells. (2)

MHV-68, while not pathogenic to humans, is a model virus for studying the pathogenesis and replication of related gammaherpesviruses, including the Epstein-Barr virus and HHV-8. Its complex interaction with the immune system response and strategic replication cycle make it a virus of importance for elucidation of viral mechanisms as well as future methods of viral treatment or eradication. Its significance on both a cellular and organismal level makes it relevant for use in research today.
References
Page created by Elizabeth Eder. Edited by student of Joan Slonczewski for BIOL 238 Microbiology, 2009, Kenyon College.