Pleurotus ostreatus
Overview
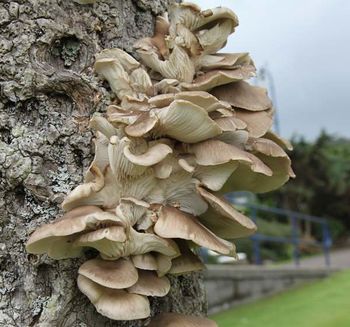
Written by Chris Goodall, Kenyon 22'
Since the commencement of the Anthropocene, humans have continued to produce increasingly significant amounts of conventionally deemed waste products. Ranging from carcinogens to complex hydrocarbons, the recent accumulation of these synthetic and organic compounds throughout global ecosystems continuously compounds into an ever increasing environmental concern.[1] Recently, efforts to combat this problematic trend have brought rise to the field of mycoremediation, a subfield of bioremediation. Mycoremediation works to potentialize non discriminate fungal enzymes as a means of concentrating, removing, and recycling complex pollutants. Among a small handful of other fungal species, Pleurotus ostreatus, a saprotroph commonly known as an Oyster Mushroom, serves a fundamental role in both the development and implementation of this field. Ironically first cultivated as a wartime ration, scientists are now looking now to this eukaryote for its potential in regard to heavy metal and organic bioremediation.
The processes of Mycoremediation practiced by Pleurotus ostreatus can occur through the following three avenues: biodegradation, biosorption, and bioconversion.[2] Biodegradation pertains to the process of disassembling complex organic peptides into more elementary mineral components (CO2, NO3, H2O). In addition to this unique enactment of mineralization, Pleurotus ostreatus also harnesses these mineral components for growth resulting with the creation of protein dense fruiting bodies (mushrooms) as a secondary product. Biosorpton centralizes less around catabolic activity and rather involves absorptive interactions with heavy metal ions. Because this process focuses around concentration rather than degradation, the fruiting bodies produced are both toxic and inedible, but have the potential for further repurposing.[3] Lastly, bioconversion pertains to the recycling of agro-industrial sludges, an umbrella term for compounded organic waste. Essentially applied biodegradation with more nutrient dense waste, this process serves as a useful alternative to recirculating said sludge as fertilizer in agricultural systems.[2]
In the following, I hope to properly highlight the significant and vastly untapped bioremediation and nutrient cycling potential of Pleurotus ostreatus.
Pleurotus ostreatus: The Next 'Heavy Metal Champion'
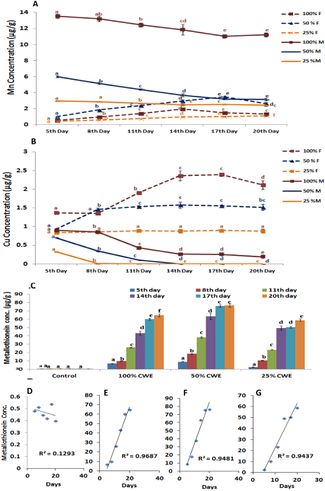
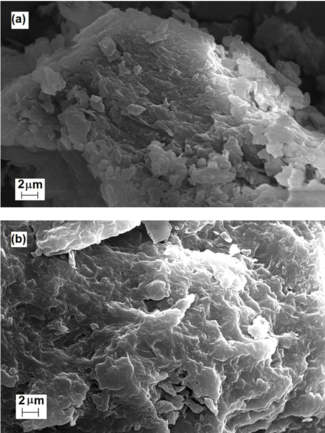
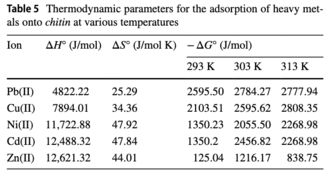
When looking to Pleurotus osteratus' potential to enact heavy metal bioremediation it's proper to first expand upon the importance of and mechanisms behind the previously outlined concept of biosorption. Heavy metal contaminants laden throughout industrial wastewater systems pose as an environmental concern due to their inability to biodegrade and their compounded toxicity under concentration. Failure to properly handle these effluents results with the accumulation of such throughout the organisms composing adjacent ecosystem invariably posing a significant health risk to the well being of the food chain.[4] Some of the more prevalent metals found in these industrial effluents include lead, cadmium, cobalt, iron, zinc, nickel, and copper.[5] All of which have the potential to form free radicals and cause detrimental confirmation changes in enzymatic function or membrane stability.[6] Prior to the more recent and widespread use of mycelium biosorption, ulterior methods have included ion exchange, chemical precipitation, coagulation, and membrane filters.[7] Although effective to a certain extent, historically, each one of these processes ends up also producing various secondary toxic sludges. Specifically looking to ion exchange, as it pertains to a similar uptake method practiced by Pleurotus ostreatus, the synthetic version of this process produces a significant amount of hydroxide sludge that almost counteracts efforts to detoxify the effluent produced.[8] It appears that instead of releasing heavy metals into waterways, industrial companies have opted for alkali sludge. Fortunately, the first step of biosorption practiced by Pleurotus osteratus poses a cheaper and more effective solution to this back ended problem.
As suggested by Tay et al. 2011, Pleurotus ostreatus uses a coupled mechanism of chemisorption with a potassium ion gradient to draw heavy metal ions into its mycelial tissues.[9] This proposed mechanism has then been observed in subsequent publications through interactions with chromium, copper, and lead.[10] The rate of nutrient uptake produced sigmoidal curves indicating second order kinetics with distinguishable lag, log, and stationary phases. Furthermore, the observed maximum biosorption capacity per mg mycelial tissue far out-succeeded the conversion factor of its synthetic ion-exchange counterparts.[9] It should be noted that the maximal biosorption potential of Pleurotus ostreatus is connected to the concentration of heavy metal ions present in the efflux. More specifically, as indicated by the research of Wassila et al. 2019, depcited in Figure 2, the uptake rates of coal efflux into the mycelial tissues of Pleurotus ostreatus under diluted concentrations resulted with greater biosorption capabilities.[5]
In addition to lacking the production of a secondary waste (in this case being an alkali sludge), under low concentrations of metals in efflux Pleurotus ostreatus mediated biosorption is also vastly less expensive than its synthetic counterparts.[11] Interestingly, the process of biosorption also doesn't necessarily rely on the Pleurotus species to be alive. According to Srinath et al. 2002, because the process of biosorbtion is metabolism independent, both living and dead cells have the potential to enact it.[12] As a result, efflux priorly too hazardous even for the survival of this versatile eukaryote can still undergo the process of biosorption with the implementation of inanimate Pleurotus osreatus.
The ion exchange gradient only comprises half of the process of biosorpton though. The second step of two centralizes around the mechanisms that concentrate and bind these heavy metals into the growing mycelial tissues. Prior to the use of mycoremedaiton, the processes of chemical precipitation and coagulation have been used to enact a similar procedure at the the limitation of being a highly time consuming process.[13] In recent years, organic polymers have also been used to bind to and absorb heavy metals, but came with the drawback of, again, producing additional secondary pollutants form a series of chemical interactions.[14] Similar to the more recent use of organic coagulants in the process of chemical precipitation, the mycelial tissue of Pleurotus ostreatus act in a variety of ways to bind and manage heavy metals.
The first of which is a process known as cell-wall adsorption.[15] This metabolically-independent process is enacted by Pleurotus osteratus through binding heavy metals to the melanin and chitin that compose its hyphal cell walls. Furthermore, melanin and chitin work exceedingly well as heavy metal receptors on account of the carboxyl, phosphate, and nitrogen containing ligand groups associated with these structural carbon compounds.[16] As previously mentioned, and on account of the lacking need for metabolic input, inanimate Pleurotus cells retain the ability to also bind heavy metals at the expensive of lacking ATP production capabilities to sequester these minerals from the hyphae into growing tissues. A secondary mechanism of handling heavy metals, as practiced by Pleurotus ostreatus and observed within the electron scanning image of its hyphal tissue produced by Tay et al. 2011 in Figure 3, involves the creation of metal precipitates known as oxalates. These concentrated pockets of heavy metal ions then intermittently reside throughout the hyphal network in an immobilized state as the species continues growth.
Regardless of being a metabolically-independent process, there still exist factors that influence uptake rates of heavy metal ions in Pleurotus ostreatus. First examining the effects of pH, it has been reported that the binding of heavy metals to chitin and associated ligand groups is limited under conditions of extreme acidity.[8] Because the high presence of hydrogen ions physically block the available surface area of the chitin molecules, the frequency of interactions with the alkali groups of these structural carbon components are significantly reduced. Similar to the responses observed with heavy metal ion uptake, the concentrations of metals present sequentially factor into biomass incorporation rates.[17] Further paralleling the kinetic results of the uptake rate of the ion gradient, the rate of concentration dependent incorporation also assumes a pseudo-second-order kinetic model. Lastly, although more specific on an elemental basis, as referenced by the work of Huma et al. 2017 in Figure 4, temperature also influences the rate of this structural ion assimilation.
In addition to serving as a cheaper and more effective alternative to synthetic coagulants, biosorption also mimics the innate potential of these precipitants in regard to the purification and repurposing of the concentrated heavy metals. Although the majority of existing research on the topic of heavy metal biosorption currently focuses around the mechanisms of uptake and comparative efficiencies to alternative treatments, the pre-acknowledged incorporation of heavy metal ions into the growing tissues and fruiting bodies of this microorganism suggest the potential for further extraction from the carbon network in which they are entwined.[3]
In summary, although previously overlooked for many years, biosorption has gained traction on account of its ability to outcompete industrial remediation counterparts from both an economic and environmental standpoint.[9] Harnessing Pleurotus ostreatus' natural heavy metal cycling potential in a more specific industrial setting has been proven to outcompete other prior biochemical remediating agents in regard to time, efficiency, versatility, and the lack of creating secondary pollutants. Furthermore, the naturally entwined process of the heavy metal ion gradient and subsequent concentration of these ions within growing hyphal tissues condenses multiple current remediation practices into a single, more effective, process.
Developments in Biodegradation and Organic Nutrient Cycling
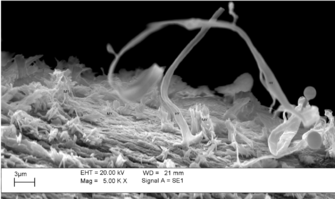
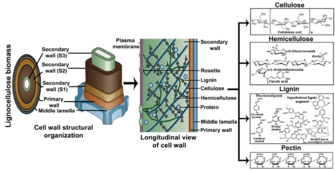
Although still significant in an environmental context, heavy metal ions from industrial efflux only compose one sector of concerning environmental pollutants. As the human race further progresses into the anthropocene, so does our demand for and reliance on sources of energy. Unfortunately, this ever increasing demand coincides with a greater potential for the production of both raw and processed organic waste. Being an essential component in the process of detrital cycling, current research now looks to Pleurotus ostreatus and other fungi for their applied potential to recycle complex molecules into their mineral components.[18] This process of mycoremediation functions under the sector of activity deemed biodegradation and has shown an extensive potential to act upon carbon based compounds
Aside from immediate concerns of oceanic petroleum spills,[19] the human race is still faced with the consequential problem of disposing the secondary pollutants produced from the fruits of these activities such as petroleum based plastic polymers. In light of the traditional approach of dumping plastic into the oceans or simply burying and forgetting about it in landfills (as of 1994 it was estimated that plastics compose 20-30% of total landfill composition[20]) recent developments into OXO-biodegradable plastics have shown a promising potential for reducing the rate of raw plastic accumulation throughout global systems.[21]
More specifically developments in the potential implementation of organic polymer biodegradation by Pleurotus ostreatus has shown exponentially increased rates of mineralization in comparison to systems lacking such presence.[22] Prior to exploring the specific mechanisms implemented for the biodegradation of these OXO-plastics, it's important to first outline the fundamental pathways implemented by microorganisms, and more specifically white rot fungi, in the process of breaking down a complex polymer. Because many polymers are too large to pass through a cellular membrane, the initial step of microbial mediated biodegradation involves one of two pathways known as direct and indirect biodegradation[22]
Focusing specifically on indirect biodegradation– as the research on Pleurotus ostreatus by Mohn, 1997 seems to indicate the use of this mechanism– this aerobic process involves the secretion of enzymes that oxidize larger polymers into subsequent monomers.[23] Upon oxidation, the fungal species continues to scavenge upon these monomers drawing them into cellular tissues for further metabolism. In the case of Pleurotus ostreatus, this process is mainly carried out through laccase cometabolism, a sub-category of lignocellulolytic activity. Simply put and as referenced by Manavalan et al. 2014's structural diagram in Figure 6 and electron scanning micrograph in Figure 5, the fungi uses a specialized series of catabolic enzymes to break down polymers into monomers through oxidation and hydrolysis. Additionally, the work of da Luz et al. 2013 found that similar to how seeding chocolate when tempering it jumpstarts the recrystallization process, adding an elementary carbon source to the fungal OXO-biodegradable plastic system showed a similar jumpstart reaction that further complemented the process of laccase cometabolism and produced evidence of complete mineralization within the first 45 days of culturing.[22]
In addition to understanding Pleurotus ostreatus' unique metabolic potential to fully degrade organic polymers, it's also critical to acknowledge the secondary benefits of complete mineralization. In contrast to how biosorption renders the growing fruiting bodies of the species inedible, when biodegrading organic molecules, the fruiting bodies of the mycelium become a protein dense product in themselves. Pleurotus species alone currently compose 25% of global mushroom cultivation, are packed with protein and essential vitamins especially important to inhabitants of developing countries, and are usually cultured on straw or barley.[24][25] Problematically, shifts to industrialization tend to decrease agricultural productions while further creating excess amounts of waste materials. One of the most common occurrences of these waste products comes in the form of sawdust, a nutrient poor lignin rich substance that, according to the U.S. Department of Agriculture, truly lacks any sort of economic value.[26]
Despite this apparent lack of nutritional presence or conventional applicability, the work of Yildiz et al, 2001. looked to the untapped potential of sawdust in the context of mycoremediation, and more specifically, in regard to the use of the substance to culture Pleurotus ostreatus. In spite of the sheer lack of nutrients present in sawdust, Yildiz et al, 2001. found that if supplemented with slightly more nutrient dense wastes such as paper, vegetative stocks, or leaves, the growth rate of the fruiting bodies of the Pleurotus ostreatus species would increase significantly.[25] The highest observed outcome from a mixed sawdust media involved 50% sawdust and 50% hazelnut leaves producing a product with an 102.5% biological efficiency.[25] Considering that the fruiting bodies of these mushrooms are arising from previously deemed useless materials, these results are quaintly impressive. Pleurotus ostreatus' usage of priorly deemed useless biomass expands upon prior conceptions of harnessable source for nutrient production and further exemplifies the potential application of this species for the mycoremediation of organic sources of waste in an environmentally productive context. Additionally, because the fruiting body of this mushroom serves as a significant source of protein, further cultivation efforts of this fungi using organic waste as a substrate could defer the sheer demand for meat products indirectly contributing to reductions in global methane production.
Carcinogen Catabolism
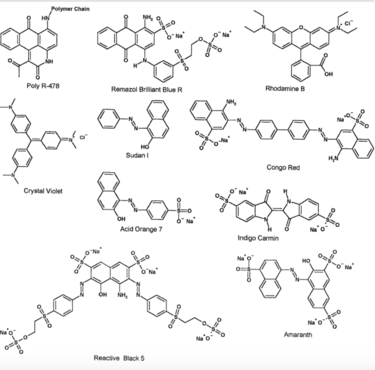
Although technically falling under the context of organic waste, due to their synthetic origins, dyestuffs released in wastewater produce exceptionally toxic and carcinogenic aromatic amines depicted in Figure 7 and deserving of their own section. Furthermore, on account of the relative size and stability of these compounds, traditional wastewater treatment strategies have proven glaringly ineffective.[27] Many of these practices rely on the use of cheap absorptive waste materials, but the use of such ends up counteractively producing secondary, more concentrated, pollutants.[28]
AZO dyes in particular, a significant class of dyestuffs, pose as a high risk threat to humans on account the potential carcinogenic implications of these aromatic amines diffusing through skin.[29] Consequentially, the majority of existing mycoremediation research centralizes around the metabolism of such. Because of their extensive potential to synthesize reactive oxygen species and harness non-specific ligninolytic enzymes, the remedial potential of white rot fungi, such as Pleurotus ostreatus has drawn considerable amounts of attention.[30] Specifically looking to Poly R-478 (a violet dye) and RBBR (Remazol Brilliant Blue R) on account of their recalcitrant nature, Novotny et al. 2001 found that laccase and MnP produced by Pleurotus ostreatus could fully decolorize the dyes in 9 and 12 days respectively.[31] Then taking into consideration the comparative reductions in economic cost and lack of secondary sludge production in regard to other treatment methods (such as organic absorbents or photocatalytic decolorization) the unparalleled potential of this species is only further emphasized.[28]
Additionally, similar to how livestock are bread for specific qualities, the latest research in the realms of dyestuff mycoremediation has looked to genetically engineer offspring of Pleurotus ostreatus and other white rot fungi to enhance their ligninolytic activity potential.[32]
Because the process of dyestuff decolorization by Pleurotus ostreatus involves the full mineralization of these aromatic amines, paralleling the end result of organic decomposition, the fruiting bodies produced through this process again have the potential to serve as feed. Contrary to organic compounds though, the usage of such relies on the full decolorization of the dyestuff prior to harvesting to ensure the absence of possible toxins. Additionally, on account of the more strict parameters involved with the consumption of fruiting bodies produced through dye bioremediation, there exists a feeble amount of research regarding the cultivation and consumption of this end product.
Conclusion
Going forth, this single eukaryote has quite the workload cut out for itself. Even though, in comparison to other fungal species, Pleurotus ostreatus isn't necessarily the most specialized agent of mycoremediation, its extensive potential to act on substances from heavy metals to complex polymers and carcinogenic compounds truly works demonstrate the barrage of benefits this organism has to offer. And that's prior to even considering the implications of complete mineralization. The creation of a non-toxic nutrient dense food source from what was previously deemed worthless, nutrient poor waste serves as a product in itself. Furthermore, as global industrialization efforts inevitably continue to develop while placing greater amounts of stress on agricultural systems, the fruiting bodies of this mushroom will most likely have the potential to upheave other, more traditional sources of protein. The resilience of this microorganism is quite frankly shocking and well deserving of a more significant amount of attention.
References
- ↑ United States Environmental Protection Agency. "Environmental Regulations and Technology: Control of Pathogens and Vector Attraction in Sewage Sludge." 2003.
- ↑ Jump up to: 2.0 2.1 Shweta et al. "Mushroom as a product and their role in mycoremediation." 2014. doi:10.1186/s13568-014-0029-8.
- ↑ Jump up to: 3.0 3.1 Gupta and Mohapatra. "Microbial biomass: an economical alternative for removal of heavy metals from waste water." PMID: 15242288.
- ↑ Almeida et al. "Chromium precipitation from tanning spent liquors using industrial alkaline residues: A comparative study." 1998.
- ↑ Jump up to: 5.0 5.1 Vaseem et al. "Heavy metal pollution due to coal washery effluent and its decontamination using a macrofungus, Pleurotus ostreatus." 2017. doi.org/10.1016/j.ecoenv.2017.07.001.
- ↑ Velásquez et al. "Biosorption and bioaccumulation of heavy metals on dead and living biomass of Bacillus sphaericus." 2009.
- ↑ Li et al . "Recovery and reuse of surfactant SDS from a MEUF retentate containing Cd2+ or Zn2+ by ultrafiltration." 2009
- ↑ Jump up to: 8.0 8.1 Saba Shamim. "Biosorption of Heavy Metals." 2018. doi:10.5772/intechopen.72099
- ↑ Jump up to: 9.0 9.1 9.2 Tay et al. "Biosorption of cadmium ions using Pleurotus ostreatus: Growth kinetics, isotherm study and biosorption mechanism." 2011.
- ↑ Bueno et al. "Biosorption of lead(II), chromium(III) and copper(II) by R. opacus: Equilibrium and kinetic studies." 2008. doi.org/10.1016/j.mineng.2007.08.013.
- ↑ Xiangliang et al. "Biosorption of Pb(II) by Pleurotus ostreatus immobilized in calcium alginate gel." 2005. doi.org/10.1016/j.procbio.2004.12.007
- ↑ Srinath et al. "Chromium (VI) biosorption and bioaccumulation by chromate resistant bacteria." 2002. doi:10.1016/S0045-6535(02)00089-9.
- ↑ Bazrafshan et al. "Heavy metals removal from aqueous environments by electrocoagulation process– a systematic review." 2015. doi:10.1186/s40201-015-0233-8
- ↑ Adhoum et al. "Treatment of electroplating wastewater containing Cu2+, Zn2+ and Cr(VI) by electrocoagulation." 2004. doi:10.1016/j.jhazmat.2004.04.018.
- ↑ Gadd. "Geomycology: biogeochemical transformations of rocks, minerals, metals and radionuclides by fungi, bioweathering and bioremediation." 2007. doi.org/10.1016/j.mycres.2006.12.001.
- ↑ Mohanasrinivasan et al. "Studies on heavy metal removal efficiency and antibacterial activity of chitosan prepared from shrimp shell waste." 2013. doi:10.1007/s13205-013-0140-6
- ↑ Boulaiche et al. "Removal of heavy metals by chitin: equilibrium, kinetic and thermodynamic studies." 2019. doi:10.1007/s13201-019-0926-8
- ↑ Kulshreshtha et al. "Mushroom as a product and their role in mycoremediation." 2014. doi:10.1186/s13568-014-0029-8
- ↑ Slonczewski and Foster. "Microbiology: An Evolving Science." 2016.
- ↑ EPA. "Characterization of Municipal Solid Waste in the United States: 1994 Update." 1994.
- ↑ da Luz et al. "Abiotic and biotic degradation of oxo-biodegradable plastic bags by Pleurotus ostreatus." 2014. doi:10.1371/journal.pone.0107438
- ↑ Jump up to: 22.0 22.1 22.2 da Luz et al. "Degradation of oxo-biodegradable plastic by Pleurotus ostreatus." 2013. doi:10.1371/journal.pone.0069386
- ↑ Monh. "Indirect bioremediation: biodegradation of hydrocarbons on a commercial sorbent." 1997. doi:10.1023/A:1008285705628
- ↑ Akinyele and Fakoya. "Anti-Growth Factors Associated with Pleurotus ostreatus in a Submerged Liquid Fermentation." 2012. doi:10.21161/mjm.03912
- ↑ Jump up to: 25.0 25.1 25.2 Yildiz et al. "Some lignocellulosic wastes used as raw material in cultivation of the Pleurotus ostreatus culture mushroom." doi:10.1016/S0032-9592(02)00040-7)
- ↑ U.S.D.A. FOREST SERVICE. "USES FOR SAWDUST, SHAVINGS, AND WASTE CHIPS." 1969.
- ↑ Singh et al. "Bioremediation of Direct Blue 14 and Extracellular Ligninolytic Enzyme Production by White Rot Fungi: Pleurotus Spp." 2013. doi: 10.1155/2013/180156.
- ↑ Jump up to: 28.0 28.1 Forgacs et al. "Removal of synthetic dyes from wastewaters: a review." 2004. doi:10.1016/j.envint.2004.02.001
- ↑ Platzek et al. "Formation of a carcinogenic aromatic amine from an azo dye by human skin bacteria in vitro." 1999. doi:10.1191/096032799678845061
- ↑ Young and Yu. "Ligninase-catalysed decolorization of synthetic dyes." 1997. doi:10.1016/S0043-1354(96)00380-6).
- ↑ Novotny et al. "Capacity of Irpex lacteus and Pleurotus ostreatus for decolorization of chemically different dyes." 2001. doi:10.1016/S0168-1656(01)00321-2/
- ↑ Srivastava et al. "Direct red decolorization and ligninolytic enzymes production by improved strains of Pleurotus using basidiospore derived monokaryons." 2014.
Authored for BIOL 238 Microbiology, taught by Joan Slonczewski, 2018, Kenyon College.