Retroviral gene therapy
Retroviral gene therapy
Retroviral therapy is the use of retroviral vectors to provide remedy to disease via the genetic modification of a patient’s own cells. Retroviral vectors are themselves derived from natural retroviruses such as HIV. The name retrovirus refers to the unique ability of these viruses to convert viral RNA into DNA. A critical part of the viral life cycle is the integration of this viral DNA into the host cell’s genome, conferring a permanent genetic change to the cell. Therefore, retroviruses may be used as a vector for gene therapy, a method of treatment dealing specifically with the alteration of genes to achieve a therapeutic effect. Gene therapy techniques are divided into three main categories; viral(including retroviral gene therapy), nonviral, and physical. The use of retroviruses bears a significant advantage over these other forms of gene therapy. Nonviral and physical techniques are less efficient in transfection and, in the case of nonviral vectors, have a more limited expression. Viral techniques, however, are more efficient in transfection and better integrate viral genes into the target genome. [1]
Introduction to retroviruses
Retroviruses belong to the family retroviridae and may be separated into three main subfamilies; oncoviruses, lentiviruses, and spuma viruses. [2] While many retroviruses are benign, some are dangerously pathogenic. HIV for example, causes significant damage to the human immune system and is extremely deadly when left untreated. Retroviridae are some of the oldest viruses, emerging between 460 and 550 million years ago. [3] One of the most important features of retroviruses is the permanent integration of viral genes into the DNA of their host. These genes are then inheritable by the offspring of the host. As a result, a significant portion of the vertebrae genome is derived from retroviral gene transfer. In fact, around 8% of the human genome consists of sequences incorporated by retroviral particles. [4]

Integration of viral DNA is an important aspect of the retroviral life cycle. Retroviruses utilize a multitude of viral genes to accomplish their replication. All retroviral particles themselves consist of at least three genes, one which encodes for internal structural proteins(gag), one which encodes for the viral envelope(env), and one which encodes for a special type of polymerase called reverse transcriptase(pol). [5] Reverse transcriptase is responsible for the conversion of viral RNA into DNA. All retroviruses contain prepackaged reverse transcriptases. Once a retrovirus has entered a host cell, the virus uncoats, releasing reverse transcriptases, RNA, and other viral molecules such as integrase into the cytoplasm. [6] Immediately after uncoating, a reverse-transcription complex forms which initiates the conversion of viral RNA to DNA. As demonstrated in retroviruses like HIV, the viral RNA is first primed for transcription via the attachment of host t-RNA(Lysine t-RNA in HIV). After which, a reverse-transcriptase binds to the t-RNA bound viral RNA forming the reverse-transcription complex. [7] The complex produces minus strand DNA which is further transcribed to form double stranded DNA. [8] This double stranded is then integrated into the host DNA. To integrate, the integration complex must enter the nucleus. Therefore in some retroviruses, integration can only occur in dividing cells during the breakdown of the nuclear envelope. Others, however, are able to enter the nucleus through pores and are thus able to infect non dividing cells. The integration process begins with the removal of two nucleotides from each 3’end of the DNA exposing the terminal 3’hydroxyl group. After which the viral DNA is inserted into a random part of the host genome. Both processes are catalyzed by the viral enzyme integrase. Once integrated, viral DNA is referred to as the provirus.

The morphogenesis of a retroviruse from the provirus is divided into three stages; assembly, budding and maturation. All three stages rely on the gag polyprotein generated from the gag reading frame. [9] In many retroviruses, it is believed that budding and maturation occur mainly near the plasma membrane of the host cell. [10] Maturation, however, occurs after the retroviral particle buds off of the cell surface and is freed into the extracellular environment. [11] Assembly begins with the provirus, which is transcribed by host mRNA machinery after integration. The first genes to be transcribed encode regulatory proteins including, Rev and Tat. While Tat upregulates transcription of the provirus, Rev enables the export of large unspliced viral mRNA transcripts. The largest of these transcripts is 9kb long and encodes for the gag protein. Once exported, the transcripts are translated by host ribosomes forming viral proteins. Once formed the gag protein mediates the recruitment of the viral genomic RNA. A region of the protein termed the nucleocapsid domain selects for two copies of the viral genome, binding them using a packaging signal located near the 5’ end of the transcript. After which, the Gag protein and the attached RNA move to the plasma membrane to begin assembly. [12] Once at the plasma membrane, Gag acts as a structural protein, coordinating the construction of the immature viral molecule from a mix of host protein, viral protein, and lipids as well as gag itself. In HIV, Gag protein makes up around 50% of the entire virion while host proteins as well as other viral proteins make up an estimated 20% of the HIV particle. [9] The gag protein consists of four domains, the previously discussed nucleocapsid region, the MA region, the CA region, and the C terminus region p6. During assembly, the MA region is primarily responsible for binding to the plasma membrane while the CA region mediates assembly and promotes the construction of the viral shell. Once both processes are complete, the virus moves on to the budding stage. To initiate budding, retroviruses hijack the host’s ESCRT pathway which is conventionally used to create multivesicular endosomes. [9] [13] As budding occurs, the virion crosses the cell’s plasma membrane using it to form its own lipid envelope. The gag protein plays a major role in budding by recruiting ESCRT proteins to the release site. [14] This step helps to mediate membrane fission and release. During recruitment, the ESCRT proteins CHMP1, CHMP2, and CHMP4 facilitate closure of the viral membrane’s neck. These ESCRT establish a spiraling filament at the neck constricting both the cell and viral membrane. After, membrane fission is directed by the recruitment of VPS4 ATPases which initiate the breakdown of ESCRT assemblies thereby catalyzing release of the viral particle. [15] After the virion releases from the membrane, it enters the final stage of replication, maturation. Maturation involves the structural rearrangement of the virion via the cleavage of the gag protein via viral protease at a number of sites. [16] The result of this cleavage is the production of multiple processed proteins which then rearrange dramatically. [9] Part of this rearrangement involves the encapsidation of the genomic RNA into a viral core which can be injected into a new host cell. After rearrangement is completed, the virion has matured and is capable of infecting other host cells.
Retrovirus particle production
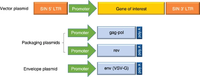
The clinical production of retroviral vectors involves the use of what’s called a packaging cell line. Packaging cell lines are transfected with a series of plasmids. Each plasmid encodes for a different necessary gene. One plasmid, referred to as the vector plasmid, contains the genes of interest that are to be transduced into the targeted host cells. The others, referred to as the packaging and envelope plasmids, contain genes encoding proteins for the retroviral particle and allow for the transfection of the host cell. These genes include the gag, env and pol sequences. The use of separate plasmids is a necessity as it ensures that genes encoding for replication factors are not transferred into the targeted host cell. If the provirus has the ability to replicate within the host cell, this would present a potential danger to the transfected individual. Therefore, the separation of genes into different plasmids is an important safety measure in the production process. Furthermore, accessory virulence factors unnecessary to viral transfer are removed completely. This further increases the safety of the vector. Once transfection is complete, the packaging cells begin producing retroviral vectors. After a few days, the vectors are removed using flow filtration and then purified. [17]
Risks
The use of retroviral vectors in gene therapy is not without risk. Retroviruses even after modification present multiple dangers to human hosts. One danger of using viruses as vectors in general is the possibility of the provirus gaining the ability to replicate within the host cell. Replication competent viruses may arise during vector production as a result of recombination between the vector and the packaging plasmids. Therefore, an important part of the manufacturing process is checking for, and reducing factors that may lead to the development of vectors capable of replicating. [18] [19] Furthermore, special measures are taken to prevent replicative sequences from recombining with the vector plasmid. In modern manufacturing approaches, the plasmid encoding the target gene is modified to contain deletions on its LTR sequence. These deletions alter the plasmid to a state of self inactivation preventing recombination with other plasmids. [17] Another possible risk factor is insertional mutagenesis, which may lead to the activation of oncogenes(genes which can promote tumor formation). Furthermore, the risk of insertional mutagenesis is substantially increased if the vector gains replication competence, which is one of the main reasons why replication competence makes a vector dangerous. The use of replicative vectors in gene transfer has been linked to lymphoma as a direct consequence of this fact. Non replicative vectors also carry risk however. Insertional mutagenesis due to retroviral gene therapy has been linked to cancer. During one trial, four out of nine patients treated for SCID-X1(X-linked severe combined immunodeficiency) developed leukemia after retroviral therapy. [10] The trial represented a major shift in the use of retroviral gene therapy and displayed the limits of using viral vectors as a means of treatment.
Cell targeting
Animal viruses can only infect certain host cells. This specificity derives from the use of host cell receptors as attachment sites. These receptors are bound using attachment proteins in the viral envelope. Many host receptors exist only on the membranes of certain cells, and therefore, the receptor targeted by the virus's attachment protein determines which cells it can infect. This is referred to as the tropism of the virus. Once attached, the virus enters the cell. Entry is mediated either by the host cell receptor the virus attaches to originally, or a completely different receptor. [20] For example, although HIV uses CD4 and CCR5 receptors to mediate entry into T-cells, the virus initially binds to receptors like nucleolin as a means of attaching to the cell. [6] The type of attachment protein used by a retrovirus is particularly important when that retrovirus is being employed in viral gene therapy. Oftentimes, the attachment protein or viral envelope of a retrovirus is modified so that the retrovirus targets a different type of host cell. This process is called pseudotyping and usually involves the insertion of either a naturally occurring viral envelope, or an engineered one in place of the virus's original envelope. [21] Pseudotyping a retrovirus allows for the vector to target virtually any host cell. [21] Pseudotyping may also be used to vastly expand the tropism(cell types in which a virus can infect) of the virion. Retroviruses modified with the VSV protein envelope may infect a wide variety of cells from hematopoietic stem cells to neurons. [22] In 1996, researchers were able to target skin fibroblasts and CD34+ cells using HIV based vectors transfected with Mo-MLV envelope proteins. [23] As discussed before, HIV primarily infects CD4+ T cells in nature, however with the envelope modifications, the tropism of the HIV based vector shifted and the vector gained the ability to infect other cell types.
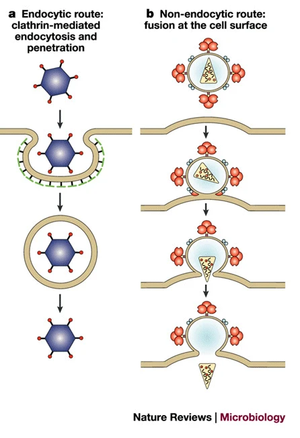
History of retroviral gene therapy
The use of modified viruses to cure human ailments through gene transfer was first suggested in a 1966 paper by Edward Tatum. The paper presented an optimistic view of how gene therapy could be used in the future, going as far to propose that such a method would provide a cure to cancer. [24] Two years later, Roger et al[25] confirmed that gene therapy was possible by using the tobacco mosaic virus to transfer added genetic material.[16] Soon after in the 1970s, the first gene therapy trial was conducted on humans. The trial infected hyperargininemic patients with the Shope papilloma virus in the hope that the virus would transfect the gene for arginase. While the trial failed to reduce arginine levels in patients it set the groundwork for further trials utilizing viruses as a method of gene therapy[26]. After which, in 1990, Steven Rosenberg and colleagues used retroviral vectors carrying the neomycin resistance marker gene to treat melanoma demonstrating the validity and safety of retroviral gene transfer in humans. [27] After which, retroviral vectors became an extremely favored form of gene therapy up until the carcinogenic nature of retroviral vectors was discovered.
Fighting HIV with HIV
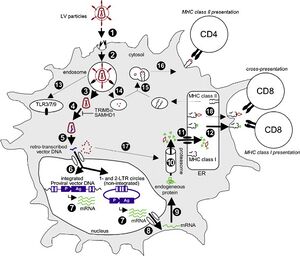
HIV or human immunodeficiency virus, is a major public health concern worldwide. Infection may lead to the development of acquired immunodeficiency syndrome which is life threatening. [28] However, HIV has high utility as a vector for gene therapy due to its transduction efficiency and ability to infect nondividing cells. Some HIV derived vectors are themselves used to combat HIV infections. HIV gene therapy involves the insertion of DNA coding for proteins which interfere with the viral life cycle. Some forms of HIV therapy target the CCR5 receptor, which HIV uses to bind to immune cells. Deletion of the CCR5 receptor does not confer any significant damage to the immune system making it a relatively safe way to impede infection by HIV. [29] Other forms of therapy include the insertion of genes which make immune cells more effective in fighting. This may mean the introduction of antibody genes which allow cells to produce molecules capable of neutralizing the virus. Furthermore, HIV-derived vectors have been tested as a means to vaccinate against HIV. Lentivectors like HIV are able to infect dendritic cells, cells which detect and present antigens to lymphocytes. [30] Vaccination using HIV vectors would involve the insertion of viral antigen genes into these dendritic cells. The inserted genes would then be translated into antigen proteins which the cell could processe and present as antigen receptors on the cell surface. Upon binding to these receptors, HIV specific immune cells would become activated and begin to proliferate. This would prepare the immune system for an HIV infection by preemptively activating the immune system to fight HIV virions.
Retroviral Therapy Against Cancer
Gene therapy of all types have found useful applications in the treatment of cancer and retroviral gene therapy is no exception. Cancer is a leading cause of death worldwide accounting for 23% of premature deaths in the United States. [31] Cancer results from an accumulation of mutations in cellular DNA, ultimately resulting in aberrant expression of cellular processes and the formation of tumors. These tumors may disrupt the functioning of the vital organs leading to disease and death. [32] Retroviral gene therapy offers a possible mode of treatment for cancer. Many retroviral therapy techniques involve stimulating the immune system to recognize and target cancer cells. An early trial using mice used retroviral vectors to inhibit metastasis formation. [33] The study targeted tumor cells with the β–galactosidase (lacZ) gene initiating an inflammatory response against them and the destruction of the tumor. Other techniques use retroviral vectors to target tumor cells with deleterious genes that result in cell death. Techniques like this have been approved for humans in the form of drugs like Rexin-G. Rexin-G is a modified vector which transfects tumor cells with what’s called a cytocidal cyclin G1 construct which initiates cell death via apoptosis. [34] Rexin-G has recently been approved by the FDA as a means to treat pancreatic cancer. Other studies have used retroviral vectors to modify immune cells so that they may target tumor formations. Morgan et al[35] used a γ-retroviral vector to transfer genes encoding a chimeric antigen receptor(CAR) into T cells. This antigen receptor allowed the transfected T cells to recognize and initiate an immunological response against glioblastoma cells. Modification of T cells to express CARs has also been used to combat melanoma and B cell malignancies like lymphoblastic leukemia and non-hodgkin's lymphoma with great success. The study Turtle et al.[36] treated non-hodgkin's lymphoma patients with a combination of lymphodepletive chemotherapy and retroviral T cell therapy. Twenty seven out of the twenty nine patients tested achieved complete remission. This demonstrates the high effectiveness of retroviral T cell therapy as well as the efficacy of the use of retroviruses to fight cancer.
Retroviral gene therapy to treat genetic disorders
Genetic error accounts for thousands of heritable diseases. These diseases result from either the loss of function of a vital gene, or the gain of function of a harmful one. Sickle cell anemia for example is a consequence of the former. The disease results from a mutation on the beta globin gene leading to disruption of expression and aberrant formation in blood cells. Like other genetic disorders, individuals with sickle cell anemia experience lower qualities of life and may develop complications resulting in a major decrease to their life expectancy. [37] Retroviral gene therapy however offers a possible cure to these diseases. Since genetic disorders are a result of errors in the DNA itself, the modification of DNA by retroviral vectors allows the problem to be fixed at the source. Retroviral vectors have been shown to be a particularly effective form of treatment for inherited blood disorders. [38] For example, in one of the first gene therapy trials, researchers used gamma-retroviral mediated gene delivery to treat a blood disorder called Adenosine deaminase deficiency(ADA). ADA is an autosomal recessive disorder which causes a host of metabolic complications and the severe weakening of the immune system. The trial involved the transduction of the gene coding for adenosine deaminase into the T cells of patients in order to correct their natural lack of the molecule. Although treatment only lasted for 2 years, the adenosine deaminase gene was continually expressed, ultimately remedying the deficiency. [39] The usefulness of retroviral gene therapy extends beyong just blood disorders however. Some studies suggest that retroviral vectors may in the future act as a means to cure neurological disorders, such as huntingtons disease. [40] The future prospects of retroviral gene therapy are exciting and may mean the curing of a wide arrange of genetic disorders.
References
- ↑ Andrew. "Gene therapy: the first decade." Trends in biotechnology 18, no. 3 (2000): 119-128.
- ↑ Johnson, and Lawrence Kleiman. "Primer tRNAs for reverse transcription." Journal of virology 71, no. 11 (1997): 8087-8095.
- ↑ Alexander. "Origin of the retroviruses: when, where, and how?." Current opinion in virology 25 (2017): 23-27.
- ↑ Dixie L., and Jonathan P. Stoye. "Mammalian endogenous retroviruses." Microbiology spectrum 3, no. 1 (2015): 3-1.
- ↑ John M. "Structure, replication, and recombination of retrovirus genomes: some unifying hypotheses." Journal of General Virology 42, no. 1 (1979): 1-26.
- ↑ 6.0 6.1 Sébastien, and Ali Saïb. "Early steps of retrovirus replicative cycle." Retrovirology 1, no. 1 (2004): 1-20.
- ↑ Kevin P., Yamuna Kalyani Mathiharan, Kalli Kappel, Aaron T. Coey, Dong-Hua Chen, Daniel Barrero, Lauren Madigan, Joseph D. Puglisi, Georgios Skiniotis, and Elisabetta Viani Puglisi. "Architecture of an HIV-1 reverse transcriptase initiation complex." Nature 557, no. 7703 (2018): 118-122.
- ↑ Evguenia S., Sara R. Cheslock, Wen-Hui Zhang, Wei-Shau Hu, and Vinay K. Pathak. "Retroviral mutation rates and reverse transcriptase fidelity." Front Biosci 8, no. 4 (2003): d117-134.
- ↑ 9.0 9.1 9.2 9.3 Wesley I., and Hans-Georg Kräusslich. "HIV-1 assembly, budding, and maturation." Cold Spring Harbor perspectives in medicine 2, no. 7 (2012): a006924.
- ↑ 10.0 10.1 Micha, Eyal Shimoni, Nir S. Gov, and Itay Rousso. "Retroviral assembly and budding occur through an actin-driven mechanism." Biophysical journal 97, no. 9 (2009): 2419-2428.
- ↑ Owen, and Barbie K. Ganser-Pornillos. "Maturation of retroviruses." Current opinion in virology 36 (2019): 47-55.
- ↑ Kun, Xiao Heng, and Michael F. Summers. "Structural determinants and mechanism of HIV-1 genome packaging." Journal of molecular biology 410, no. 4 (2011): 609-633.
- ↑ Eiji, and Wesley I. Sundquist. "Retrovirus budding." Annu. Rev. Cell Dev. Biol. 20 (2004): 395-425.
- ↑ Daniel R., Marc C. Johnson, Watt W. Webb, and Volker M. Vogt. "Visualization of retrovirus budding with correlated light and electron microscopy." Proceedings of the National Academy of Sciences 102, no. 43 (2005): 15453-15458.
- ↑ Melissa D., Jack J. Skalicky, Collin Kieffer, Mary Anne Karren, Sanaz Ghaffarian, and Wesley I. Sundquist. "ESCRT-III recognition by Vps4 ATPases." Nature 449, no. 7163 (2007): 740-744.
- ↑ 16.0 16.1 Owen, and Barbie K. Ganser-Pornillos. "Maturation of retroviruses." Current opinion in virology 36 (2019): 47-55.
- ↑ 17.0 17.1 Michael C., and Una O’Doherty. "Clinical use of lentiviral vectors." Leukemia 32, no. 7 (2018): 1529-1541.
- ↑ Yajin, Susan Sun, Ibe Oparaocha, Laurent Humeau, Brian Davis, Reuben Cohen, Gwendolyn Binder, Yung‐Nien Chang, Vladimir Slepushkin, and Boro Dropulic. "Generation of a packaging cell line for prolonged large‐scale production of high‐titer HIV‐1‐based lentiviral vector." The Journal of Gene Medicine: A cross‐disciplinary journal for research on the science of gene transfer and its clinical applications 7, no. 6 (2005): 818-834.
- ↑ Donald S. "The use of retroviral vectors for gene therapy-what are the risks? A review of retroviral pathogenesis and its relevance to retroviral vector-mediated gene delivery." Genetic vaccines and therapy 2, no. 1 (2004): 1-13.
- ↑ Dimiter S. "Virus entry: molecular mechanisms and biomedical applications." Nature Reviews Microbiology 2, no. 2 (2004): 109-122.
- ↑ 21.0 21.1 Alok V., and Salemiz Sandoval. "Pseudotyped lentiviral vectors: one vector, many guises." Human Gene Therapy Methods 28, no. 6 (2017): 291-301.
- ↑ Shuang, Mingjie Li, and Ramesh Akkina. "Generation of high-titer pseudotyped lentiviral vectors." In Viral Vectors for Gene Therapy, pp. 125-134. Humana Press, New York, NY, 2019.
- ↑ Jakob, George Harmison, Stephanie Kluepfel-Stahl, Roscoe O. Brady, Stefan Karlsson, and Manfred Schubert. "Transduction of nondividing cells using pseudotyped defective high-titer HIV type 1 particles." Proceedings of the National Academy of Sciences 93, no. 26 (1996): 15266-15271.
- ↑ Edward L. "Molecular biology, nucleic acids, and the future of medicine." Perspectives in biology and medicine 10, no. 1 (1966): 19-32.
- ↑ Stanfield, and Peter Pfuderer. "Use of viruses as carriers of added genetic information." Nature 219, no. 5155 (1968): 749-751.
- ↑ David, and Karine Breckpot. "Lentiviral vectors in gene therapy: their current status and future potential." Archivum immunologiae et therapiae experimentalis 58, no. 2 (2010): 107-119.
- ↑ Ana P., and Bruce J. Baum. "Gene therapy: some history, applications, problems, and prospects." Toxicologic pathology 36, no. 1 (2008): 97-103.
- ↑ Jade, Babafemi Taiwo, Soraya Seedat, Brigitte Autran, and Christine Katlama. "Hiv." The Lancet 392, no. 10148 (2018): 685-697.
- ↑ Nunzio, Francesca, T. Felix, N. J. Arhel, S. Nisole, P. Charneau, and A. S. Beignon. "HIV-derived vectors for therapy and vaccination against HIV." Vaccine 30, no. 15 (2012): 2499-2509.
- ↑ Diana, James W. Young, and Jacques Banchereau. "Dendritic cells." Advances in immunology 72 (1999): 255-324.
- ↑ Bruce N., Lois Swirsky Gold, and Walter C. Willett. "The causes and prevention of cancer." Proceedings of the National Academy of Sciences 92, no. 12 (1995): 5258-5265.
- ↑ Jiro, Victorio Rodriguez, and Gerald P. Bodey. "Causes of death in cancer patients." Cancer 33, no. 2 (1974): 568-573.
- ↑ Robert K., Glenn Dranoff, Richard C. Mulligan, and Robert I. Tepper. "Gene therapy of metastatic cancer by in vivo retroviral gene targeting." Nature genetics 10, no. 4 (1995): 430-435.
- ↑ Seth, Noah Federman, Erlinda M. Gordon, Frederick L. Hall, and Sant P. Chawla. "Rexin‑G®, a tumor‑targeted retrovector for malignant peripheral nerve sheath tumor: A case report." Molecular and Clinical Oncology 6, no. 6 (2017): 861-865.
- ↑ Richard A., Laura A. Johnson, Jeremy L. Davis, Zhili Zheng, Kevin D. Woolard, Elizabeth A. Reap, Steven A. Feldman et al. "Recognition of glioma stem cells by genetically modified T cells targeting EGFRvIII and development of adoptive cell therapy for glioma." Human gene therapy 23, no. 10 (2012): 1043-1053.
- ↑ Cameron J., Laïla-Aïcha Hanafi, Carolina Berger, Theodore A. Gooley, Sindhu Cherian, Michael Hudecek, Daniel Sommermeyer et al. "CD19 CAR–T cells of defined CD4+: CD8+ composition in adult B cell ALL patients." The Journal of clinical investigation 126, no. 6 (2016): 2123-2138.
- ↑ J. B., A. J. Duits, F. A. Muskiet, H. Ten Cate, R. A. Rojer, and D. P. Brandjes. "Sickle cell disease; a general overview." Neth J Med 62, no. 10 (2004): 364-74.
- ↑ Barbara, Eugenio Montini, Giulietta Maruggi, Alessandro Ambrosi, Massimiliano Mirolo, Silvia Selleri, Erika Biral et al. "Integration of retroviral vectors induces minor changes in the transcriptional activity of T cells from ADA-SCID patients treated with gene therapy." Blood, The Journal of the American Society of Hematology 114, no. 17 (2009): 3546-3556.
- ↑ R. Michael, Kenneth W. Culver, A. Dusty Miller, Charles S. Carter, Thomas Fleisher, Mario Clerici, Gene Shearer et al. "T lymphocyte-directed gene therapy for ADA− SCID: initial trial results after 4 years." Science 270, no. 5235 (1995): 475-480.
- ↑ Mark H. "Growth-factor gene therapy for neurodegenerative disorders." The Lancet Neurology 1, no. 1 (2002): 51-57.